Tuberculosis and Nontuberculosis Mycobacterium
Jean Deschênes
David E. Lederer
Saad Ahmed Sultan Al-Khalifa
Tuberculosis (TB) is a chronic bacterial infection caused by Mycobacterium tuberculosis and is characterized by the formation of granulomas. Nearly one-third of the world’s total population is infected with the TB bacilli.1 Although the most common site of infection is the lungs, other organs may be involved. This chapter discusses the epidemiology and classification, pathogenesis, diagnosis, ocular manifestations, treatment, and complications of treatment of Mycobacterium tuberculosis. Additionally, nontuberculous mycobacteria is reviewed highlighting clinically relevant information.
Epidemiology and Classification
Epidemiology
Although the major bacterial pathogen of tuberculosis is the Mycobacterium tuberculosis organism, it is only one member of the Mycobacterium tuberculosis complex. The Mycobacterium tuberculosis complex constitutes a genetically closely related group of bacteria that is thought to represent subspecies of Mycobacterium tuberculosis. The Mycobacterium tuberculosis complex consists of Mycobacterium tuberculosis, Mycobacterium africanum, Mycobacterium bovis, Mycobacterium microti, and Mycobacterium canetti.3 The host range, epidemiology, pathogenecity, and laboratory genotype and phenotype serve to characterize the varying subspecies. With the improvement in laboratory techniques, the taxonomy and speciation of Mycobacterium tuberculosis complex will continue to be challenged.4
The World Health Organization considers tuberculosis to be a global pandemic with 2 billion persons infected with the M. tuberculosis bacilli, equaling one-third of the world population. One in ten persons infected with the M. tuberculosis bacilli will go on to develop active tuberculosis and there are 5,000 tuberculosis-related deaths daily. Although 98% of these deaths are in the developing world there are still 15 million persons infected with M. tuberculosis in the United States.1,2
Within the United States there has been a steady decline of new cases of TB since its rising incidence in the early 1990s. This transient increase in TB was attributed largely to the impact of the increasing number of persons infected with HIV. The decline in incidence has paralleled the greater use of directly observed therapy as well as the proportion of persons completing therapy within 1 year of diagnosis.5
The natural history of TB is that by 5 years 50% of persons infected with pulmonary TB will die, 25% will obtain a self-cure from a strong immune system response, and 25% will remain chronically infected. Furthermore, 90% of immunocompetent persons who become infected with the M. tuberculosis bacilli will remain healthy. Yet there is a 50% lifetime risk of developing active tuberculosis in an HIV-positive person who becomes coinfected with M. tuberculosis. As HIV progresses and CD4+ T-lymphocyte counts diminish, extrapulmonary and disseminated tuberculus disease becomes more common.6
Highly active antiretroviral therapy (HAART), a combination of at least three antiretroviral drugs, has become the standard of care for HIV treatment. Although HAART can result in immune system reconstitution and a decrease in susceptibility to develop tuberculosis, the initiation of treatment is complicated. An experienced and knowledgeable physician in this rapidly evolving area is recommended to minimize HAART and TB drug therapy interactions and monitor for side effects. For example, in immune reconstitution syndrome a paradoxical worsening of signs and symptoms of manifestations of TB may occur after commencing anti-TB and HAART treatment simultaneously.
Furthermore the emergence of multidrug resistant strains of tuberculosis (MDR-TB) continues to complicate treatment algorithms. It can arise from inadequate or incomplete treatment regimens and may occur in a previously diagnosed individual with TB or a new case originating from an infective source with MDR-TB bacilli. The growing burden of MDR-TB on the society is still small with 3.2% of all newly diagnosed TB cases in 2000 being MDR-TB.7 Yet, because the major survival selection factor for MDR-TB is failure to complete therapy, directly observed therapy will serve to eliminate this problem without increasing the financial burden of tailoring or extending chemotherapy regimens.8
Although 20% of tuberculosis occurs extrapulmonary, the prevalence of rates of ocular TB is difficult to estimate. Historically, ocular TB was thought to represent 1.4% of all cases,9 yet more recent data suggest that 18% of culture-proven patients with TB have ocular lesions suggestive of tuberculosis.10 A recent review of 92 well-documented single cases of intraocular tuberculosis showed the most common presenting manifestation was choroidal mass with or without inflammatory signs in 34%, choroiditis/chorioretinitis in 27%, vitritis in 24%, iridocyclitis/anterior chamber reaction in 13%, and panophthalmitis in 11%.11
Classification
Primary infection must be differentiated from primary disease. Primary TB infection is caused by inhalation of infected particles. These droplets are produced when a person infected with pulmonary or laryngeal TB cough, sneeze, speak, or undergo diagnostic manipulation (such as bronchoscopy). The American Thoracic Society notes four key factors determining the likelihood of transmission of TB. These are: the number of organisms being expelled into the air, the concentration of organisms in the air determined by the volume of the space and its ventilation, the length of time an exposed person breathes the contaminated air, and presumably the immune status of the exposed individual.12
Primary infection results in granuloma formation and a dormant stage in which the body’s immune system keeps the tubercle bacilli sequestered. These individuals are asymptomatic and noninfectious. In persons in whom the body’s immune system is unable to keep the bacilli sequestered, primary disease will result. In individuals with primary disease the bacilli undergo multiplication and spread through the lungs and hilar lymph nodes and into the bloodstream. Such individuals are highly contagious, and extrapulmonary or even disseminated (miliary), TB can occur.
Secondary disease is characterized by a reactivation of previously dormant foci from the primary infection. This process is most common in the first 2 years following infection. Reactivation occurs because of a decrease in the cellular immune response. Reactivation TB, or secondary disease, can involve both pulmonary and extrapulmonary disease. Extrapulmonary TB includes pleural effusions, scrofula (painless lymphadenitis, usually cervical), gastrointestinal infection (ileocecal, peritoneal), pericarditis, genitourinary involvement, spondylitis (Pott’s disease), articular and osseous TB, head and neck involvement (laryngeal disease), chronic skin nodules (lupus vulgaris), central nervous system involvement (meningitis, intracranial tuberculoma), and ocular infection. Reinfection with new bacilli is possible, although rare. Secondary disease with ocular manifestations has been termed presumed ocular tuberculosis indicating the difficulty that may arise in establishing this diagnosis with certainty (see Diagnosis section).
The American Thoracic Society further classifies individuals into the following categories. 0: No tuberculosis exposure, not infected; 1: Tuberculosis exposure, no evidence of infection; 2: Latent tuberculosis infection, no disease; 3: Tuberculosis, clinically active; 4: Tuberculosis, not clinically active; 5: Tuberculosis suspect (diagnosis pending).12
Pathogenesis
In 1921, the first attenuated strain of M. bovis was given to humans as a vaccine to prevent TB. Since then, our understanding of the immune system’s response to TB has advanced considerably and M. tuberculosis represents one of the first pathogens to have a complete genomic sequence obtained.13
Upon inhalation the bacilli is phagocytozed by pulmonary alveolar macrophages that initiate the host response. Macrophage-derived proinflammatory cytokines (TNF, IL-1, IL-6) and transcription factors (NF-IL6, NF-κB) are critical to the early immune response. Cellular recruitment occurs and a granuloma is formed to wall off the incompletely destroyed bacilli.14 The tuberculous granuloma consists of a well-circumscribed area of central necrosis surrounded by giant epitheliod cells. It may eventually fibrose and undergo calcification that is visible on a chest radiograph.
The TH1 CD4+ subset of lymphocytes is predominantly active in eradicating TB bacilli and the TH2 CD4+ subset may allow TB infection to persist. Both coexist during infection with TB and help to determine the host response and explain skin test observations. The balance between activating and inhibiting cytokines may influence the systemic course of TB infection. CD4+ lymphocytes maintain quiescence of latent TB foci by active immunologic surveillance. Cytokines such as IL-2, IL-12, IFN-γ, and TNF-α common to TH1 CD4+ activation have the potential for enhancing immune responsiveness to TB. Alternatively, IL-10 and TGF-β tend to limit the host’s eradication of TB.
Although the humoral response to TB has aided in the identification of lipid and protein components of the bacilli, the specific antibody response remains elusive. Development of diagnostic tools and vaccination are the principal areas of humoral-related immune response research.
Enhancing an individual’s preexisting immunity to TB has been done historically with the BCG (Bacille Calmette-Guérin) vaccine (see “Treatment”), although novel approaches are needed. BCG is known to have its best efficacy in preventing systemic dissemination and extrapulmonary TB in infants and children. It also has a modest ability to prevent primary infection with TB. Repeated administration of BCG may boost immunity to TB but is fraught with the limitations of live attenuated bacteria—particularly in the immunosuppressed patient. A booster of purified subunit TB antigens such as ESAT-6 or 85Bag can heighten the immune response to TB, but maximal clinical benefit is likely to be achieved with a multivalent vaccine.15 Vaccination with auxotrophic mutants,16 low-dose immunotherapy with IL-2,17 heat-killed Mycobacterium vaccae, IFN-γ,18 thalidomide (a specific TNF-α inhibitor),19 and pentoxifylline (a nonspecific cytokine inhibitor)20 all have had some success in controlling TB infection. The recognition of HLA-DR2 susceptibility to TB21 and specific gene mutations such as the IFN-γ22 and defective production of TNF-α,23 which predispose to progressive disease, are important in regulating the host response to TB.
Eales’ disease represents an example of a local ocular immune response believed to be secondary to hypersensitivity to a capsular protein on the tubercle bacillus.24 Most patients are PPD positive (see Diagnosis section). PCR analysis of aqueous fluid, vitreous fluid, and epiretinal membranes have been positive for tuberculosis further strengthening this connection.25,26,27
The complicated area of cellular and humoral immunity, as well as that of host genetic factors to the M. tuberculosis organism, continues to be active areas of investigation.
Diagnosis
Acid-fast microscopy of sputum or, in extrapulmonary TB, other body fluids, and/or tissues followed by culture confirmation remain the cornerstone of the diagnosis of TB. New rapid culture techniques, polymerase chain reaction (PCR), and sensitivity testing all have roles to play in the diagnosis of TB. Chest radiographs may also support the clinical diagnosis.
The classical chest radiograph can show calcified granulomas, multinodular infiltrates with cavitation in the upper segments of one or both lungs, or a diffuse (miliary) pattern. However, TB may produce any form of pulmonary radiographic abnormality, especially in immunosuppressed persons. Tuberculous activity should be assessed with serial imaging to differentiate early reactivation from chronic healed lesions. Computed tomography (CT) may show characteristic nodules and branching linear structures (tree-in-bud pattern) as well as cavitation with scarring.28
Direct ocular involvement is most commonly the result of choroidal involvement although any part of the eye, orbit or visual pathway may be infected (see Ocular Manifestations section). When the clinical and radiologic findings are suggestive of TB, the diagnosis is supported further by a moderately or strongly positive PPD skin test (see tuberculin skin test below). A patient with intraocular inflammation and a positive PPD test result has a 1% likelihood of having active TB.29 This low probability means that the PPD is not useful in the routine evaluation of patients with intraocular inflammation. Its indiscriminate use may lead to improper diagnosis, increased costs, and occasionally inappropriate therapy. In a retrospective study of patients with tuberculous intraocular inflammation, 67% of patients had positive PPD results.30 Studies from Japan have shown that although 21% of cases of uveitis may be PPD positive only 8% were clinically suspected to have intraocular tuberculosis.31 Thus, PPD should be used in the screening evaluation of ophthalmic patients only if there is information that would lead to a high pretest likelihood of TB. A history of weight loss and night sweats, histroy of chronic or recurrent pulmonary infection or past exposure to TB and a suggestive chest radiograph increase the likelihood of diagnosis.
The complexity in diagnosis is due to the lack of a 100% specific and sensitive diagnostic test. Acid fast smears along with culture and sensitivity provide confirmatory and susceptibility data. Other useful tests include the tuberculin skin test, chest radiograph, complete physical examination and PCR testing.
The tuberculin skin test is based on the fact that infection with M. tuberculosis produces a delayed-type hypersensitivity reaction to specific antigenic constituents of the organism that are contained within the extracts of culture filtrates (tuberculins). These purified protein derivatives (PPD) are small proteins, polysaccharides, and lipids isolated from M. tuberculosis. The standard test dose of 0.1 mL of 5-TU (tuberculin units) of PPD injected intradermally (Mantoux method) using a 27-gauge needle on a tuberculin syringe is performed on the forearm to raise a bleb. On analyzing the results at 48 to 72 hours, the important feature is the presence of induration as measured by palpation and not erythema.32
The American Thoracic Society lists three cutoff values of induration to indicate a positive test based on sensitivity and specificity of the tuberculin skin test as well as the prevalence of TB in a given population. A test is considered positive with ≥5 mm of induration for persons at greatest risk of developing TB (HIV-infected persons, immunosuppressed persons, persons in recent contact with a confirmed TB case and persons with chest radiograph findings consistent with TB). A test is considered positive with ≥10 mm of induration for persons at intermediate risk of developing TB (recent arrivals from high prevalence countries, injection drug users, residents and employees of high risk congregate settings, mycobacteriology laboratory personnel, persons with high-risk medical conditions, children younger than 4 years of age and all nonadults exposed to those in high-risk categories). Finally, a test is considered positive with ≥15mm of induration for person at least risk of developing TB (none of the aforementioned risk factors for TB development).12
The PPD testing and interpretation can be complicated by a multitude of factors. Other than the risk factors noted above there are other confounding factors. These include (but are not limited to) BCG vaccination status, waning immunity of infected individuals, active/severe infections, recent live vaccination for other organisms and improperly performed or interpreted tests. An experienced physician is critical in determining the accuracy of diagnosis.
Although tuberculin skin testing may aid in the diagnosis, a negative result does not exclude a diagnosis of TB. Other ways to confirm a diagnosis of TB include smears and culture of the mycobacterium. The mycobacteria are strictly aerobic bacilli, nonspore-forming and nonmotile. The high-lipid content of the cell wall enables binding with certain dyes (fuchsin) and the resulting complexes resist decolorization with acid alcohols. Thus these organisms are called acid-fast. Commonly used acid-fast stains are the Ziehl-Neelsen, Kinyoun stains, and fluorochrome techniques. Fluorochrome staining (using phenolic auramine or auramine-rhodamine with methylene blue counterstaining) is the preferred method of staining because it is more sensitive and can stain nonviable organisms; whereas the Ziehl-Neelsen and Kinyoun stains may not stain nonviable organisms. The acid-fast organisms appear bright orange-yellow against a dark background on light microscopy.
The M. tuberculosis organisms are slow growing with a generation time of about 20 hours. The most commonly used culture medium is the egg-based Lowenstein-Jensen medium. M. tuberculosis grows best at 35°C to 37°C in 5% to 10% carbon dioxide. Cultures may become positive at 18 to 24 days with egg-based media or slightly earlier with agar media. Cultures should be examined weekly for 6 to 8 weeks before being discarded as negative. More rapid methods for detection of mycobacteria currently are available. Commercial broth-based systems can detect growth within 1 to 3 weeks and represent a valuable contribution to diagnosis.33 Additionally, PCR and nucleic acid amplification techniques show promise to further enhance diagnostic speed and accuracy, but final conclusions on every day utility are still pending.34,35 Nevertheless, the ophthalmic literature cites many examples of PCR detection of mycobacterial DNA and may be the test of choice based on small sample availability for presumed ocular cases.36,37,38,39 Nested PCR and ELISA testing of serum, whereas less studied than tradition PCR, have also been noted to be useful in diagnosing ocular TB.39,40 Speciation of various mycobacterium can be accomplished based on agar morphology, and biochemical testing—including the classic positive niacin and nitrate tests, catalase activity, nucleic acid hybridization and high performance liquid chromatography (HPLC) techniques.12 Drug susceptibility testing can be accomplished with standard culture techniques (e.g., the proportion method compares the number of colonies growing on a standard medium with the number on a drug-containing medium) and is available for the liquid broth systems as well.
Any combination of the previously noted techniques may be used to attain a diagnosis of presumed ocular tuberculosis. Although no generally accepted guidelines are available, Gupta et al. suggest specific combinations of clinical signs (intraocular cellular reaction, snow balls, perivascular cuffing, choroidal or optic disc granuloma, and subretinal abcess), diagnostic testing (positive AFB/culture/PCR/DNA testing from intraocular samples, PPD, chest radiography, and AFB/culture of other extrapulmonary sites), and therapeutic trials (response to multidrug anti-TB therapy over 4–6 weeks) may provide evidence to support a diagnosis of ocular tuberculosis.10
Ocular Manifestations
In patients with chronic ocular inflammation and a compatible history, TB should be considered, especially if the inflammation is unresponsive to conventional anti-inflammatory therapy. The most common form of ocular involvement is uveitis9; however, any of the ocular tissues can be involved. Often there is detectable systemic disease,41 but eye disease also can occur without clinically evident extraocular TB. The incidence of tuberculous ocular involvement in systemic TB is between 1% and 18%.9,10,42 However, the true incidence may be higher because the facilities to repeatedly screen patients for ocular manifestations seldom are in place where TB is most prevalent. Ocular studies with adequate microbiological support in the postchemoprophylaxis, AIDS-influenced, modern era of TB are lacking.
The mechanisms of ocular and adnexal involvement include active infection and immunogenic disease.
Active infection can occur as a primary ocular infection caused by introduction into the eye by contaminated hands or fomites, or exposure to dust or sputum particles laden with bacilli.43 More commonly, active ocular or adnexal infections occur secondarily by hematogenous spread of tubercle bacilli from a distant site of active primary or secondary stage systemic disease or by contiguous spread (e.g., nasal sinuses, meninges). The result is granuloma (tubercle) formation that can involve any part of the eye, orbit, or visual pathways.
Eyelids
Primary infection of the eyelid is characterized by ulceration and lymphadenopathy. However, lid involvement usually occurs as lupus vulgaris, a slowly progressive, chronic process without regional lymphadenopathy, which begins as a small tubercle under the epithelium. The nodule becomes more superficial in location, varying in size from pinpoint to pea-sized, with surrounding erythema.44 The nodules increase in numbers, forming a lupus-patch. Large areas can become involved and result in severe cicatrization, causing ectropion and corneal exposure.
Orbit
Orbital involvement is a rare ocular manifestation of tuberculosis. Orbital involvement can include cellulitis, periostitis, osteomyelitis, abscess formation, and chronic dacryoadenitis. Tuberculous orbital involvement may displace the globe, cause irregular destruction of the orbital walls, and induce hyperostosis.45 These lesions usually are of metastatic origin. Fistula formation can occur.46 Dacryocystitis can be primary or secondary to adjacent osseous TB. Occasionally in orbital TB, only a biopsy with immunohistochemical analysis could confirm the diagnosis.47
Conjunctiva
Tuberculosis of the conjunctiva is very rare. Infection can be primary, in which the source is exogenous (e.g., airborne particles, trauma). Secondary infection can be endogenous via the bloodstream or by direct extension from an adjacent focus. Bulbar conjunctival nodules in a patient with a history of TB should make one suspicious of conjunctival TB.48 Conjunctivitis may start slowly as a granulomatous lesion or present as an acute purulent or pseudomembranous conjunctivitis. Primary conjunctivitis can be accompanied by visible lymph node involvement, that is, Parinaud’s oculoglandular syndrome. Gross lymph node involvement is not typical of secondary infections but may occur.49,50
Cornea
Corneal involvement usually is allergic in origin (phlyctenulosis, interstitial keratitis) or is secondary to spread from adjacent structures (sclerokeratitis). Primary infectious keratitis has been described but is rare. Phlyctenular keratoconjunctivitis is the most common form of external ocular TB (Fig. 58.1). Phlyctenulosis may be caused by tubercle protein hypersensitivity.51
Interstitial keratitis typically affects one eye (Fig. 58.2). The tuberculoproteins of the cell wall of M. tuberculosis are responsible for causing allergic reactions in the cornea, producing interstitial keratitis.52 The clinical course is prolonged and may involve frequent attacks of inflammation. Infiltration in TB usually is peripheral and sectoral and spares the central cornea (Fig. 58.3). Often there is associated scleritis. TB usually affects the superficial and middle layers of corneal stroma; vascularization follows, with the vessels usually located in the anterior stroma. Residual localized opacification ensues because of necrosis in areas of infiltration. These features are in contrast to luetic interstitial keratitis, which involves the stroma more posteriorly, affect the central and peripheral cornea, and tends to leave less opacification because corneal infiltration usually is not as severe.53
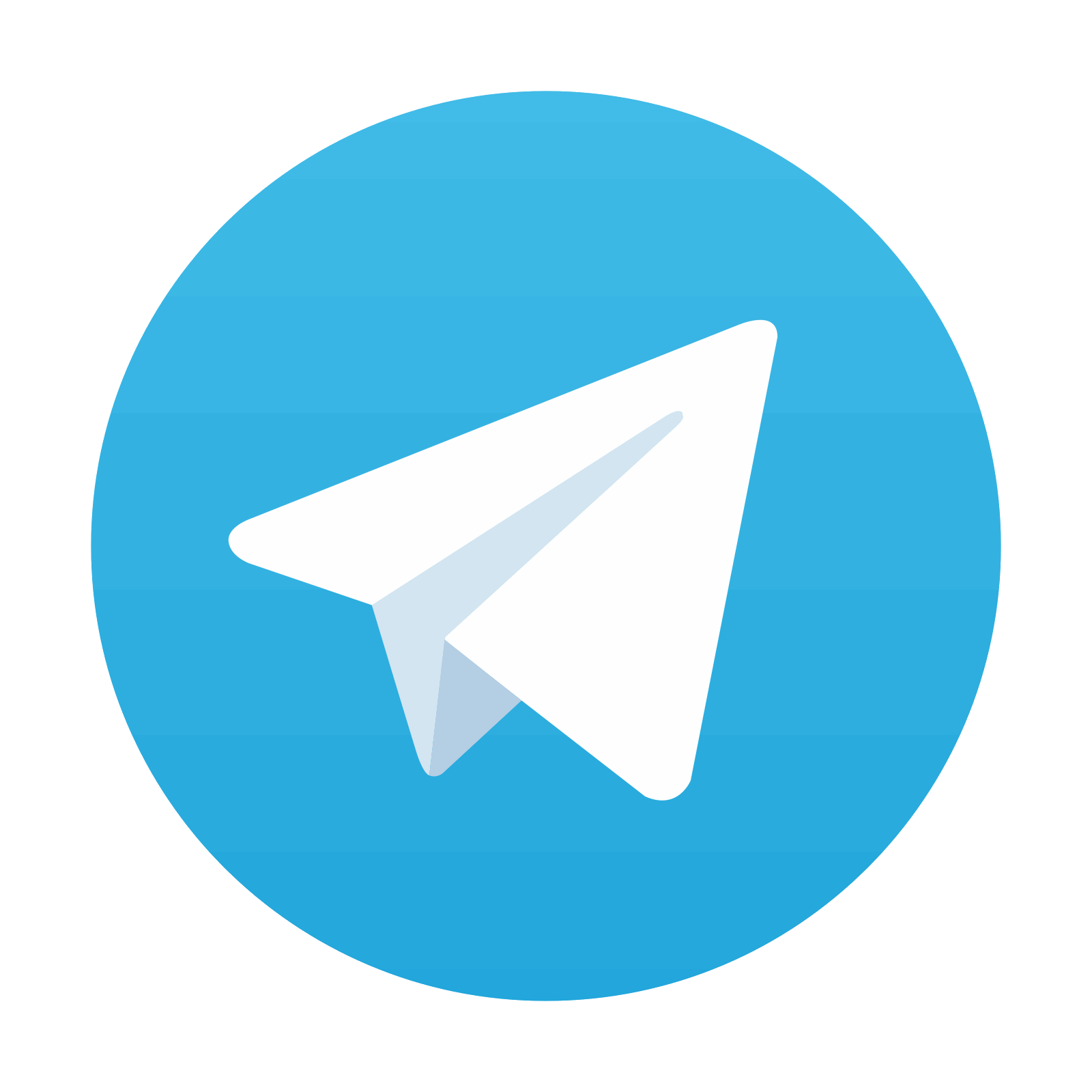
Stay updated, free articles. Join our Telegram channel

Full access? Get Clinical Tree
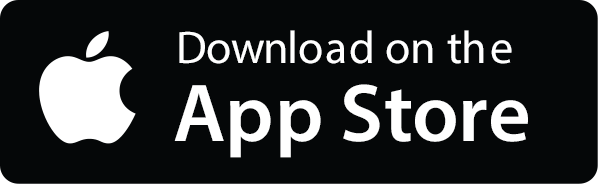
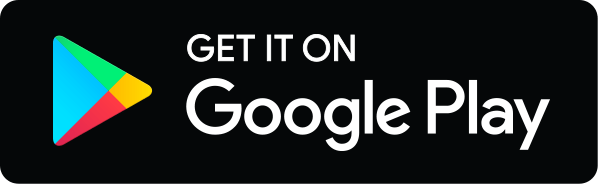