The Pharmacology of Prostaglandin Analogues
Deepta Ghate MD
Carl B. Camras MD
Department of Ophthalmology and Visual Sciences, University of Nebraska Medical Center, Omaha NE
Supported in part by an unrestricted grant from Research to Prevent Blindness, New York, NY
Corresponding author: Carl B. Camras, MD
Department of Ophthalmology and Visual Sciences, University of Nebraska Medical Center, 985540 Nebraska Medical Center, Omaha, NE 68198-5540, Phone number: 1-402-559-4276, Email: cbcamras@unmc.edu
Introduction
Prostaglandin analogues (PGAs) have revolutionized the management of glaucoma. With their intraocular pressure (IOP) reducing efficacy combined with few systemic side effects and once a day dosing, PGAs have become the drugs of first choice for IOP reduction.(1,2) During the last decade, there has been an explosion in our knowledge of this class of drugs.
This chapter provides a brief historical perspective on the ocular use of PGAs and reviews their pharmacology, ocular and systemic pharmacokinetics, mechanism of action, clinical properties, and the pathophysiology behind their side effect profiles.
Historical Perspective
The word prostaglandin (PG) is derived from the prostate gland. Independently, both Von Euler and Goldblatt first isolated what are now known as PGs, from seminal fluid in 1935.(3,4) Ambache first isolated a substance he called irin, in 1955, from rabbit irides which induced miosis.(5) This biologically active substance irin was later found to contain a mixture of PGs and other compounds.(6,7)
In the 1960s and 1970s, PGs were known to be present ubiquitously in the human body. In the ocular tissues, they were assumed to mediate the inflammatory response, causing a breakdown of the blood-aqueous barrier (BAB) and increasing IOP when injected into the anterior chamber of experimental animals.(8) As other immunomodulators and inflammatory mediators were discovered, the PGs were recognized to have less of a causal role in ocular inflammation(9) and were found to have a variable response on IOP depending on the dosage.(10) PGF2α or PGE2 at a dosage of 25-200 μg applied topically to the rabbit eye caused an initial hypertensive phase followed by prolonged IOP reduction for 15-20 hrs, whereas reducing the PGF2α dosage to 5μg maintained an IOP reduction without the initial hypertensive phase.(10) These results, demonstrating a reduction in IOP after topical application of PGs in conscious animals first suggested that PGAs might be useful in glaucoma therapy.
Initial clinical studies with PGAs included the PGF2α tromethamine salt,(11,12) that was initially evaluated in the rabbit(10)and in the monkey.(13) Other PGAs evaluated included PGE2 and its analogues(10,14)and PGD2 analogues.(15) Although some IOP reduction was found in these studies, the main limiting factor was severe conjunctival hyperemia, burning, irritation and foreign body sensation after instillation. Development of the isopropyl ester of PGF2α allowed dosing with a considerably lower concentration with correspondingly fewer ocular side effects.(16,17,18,19) Similar to the relationship between dipivefrin and epinephrine, the prodrug PGF2α-isopropyl ester is converted to the free acid PGF2α by the esterases in the cornea.(16) This esterified prodrug provided evidence that ocular side effects could be reduced by drug modification without sacrificing the IOP lowering efficacy of the drug.
A terminal phenyl ring attached to C17 in PGF2α and the saturation of the double bond between C13 and C14 was found to improve the receptor selectivity of the compound at the prostanoid FP receptor, reduced the hyperemic effect and resulted in a more chemically stable drug.(20,21,22) These modifications translated into the development and ultimate clinical usage of latanoprost (13,14-dihydro-17-phenyl-18,19,20-trinor-PGF2α isopropyl ester; Xalatan®). Similar to the PGF2α– isopropyl ester, latanoprost also is a prodrug. Ester hydrolysis of latanoprost is rapid and complete, and the biologically active latanoprost acid is formed.(23,24) Similar to latanoprost, travoprost (Travatan®) is a prodrug of a well known prostanoid FP receptor agonist, fluprostenol,(25) and is hydrolyzed to its biologically active carboxylic acid, travoprost acid. Based on the ethyl amide group at the C1 position rather than the isopropyl ester, bimatoprost (Lumigan®) has been controversially classified by some as a “prostamide.”(26) Others have provided strong evidence that bimatoprost is an amide prodrug of its free acid, bimatoprost acid, which is known to be a potent agonist at the FP prostanoid receptor. This controversy is addressed further in the Pharmacokinetics Section.
Four PGAs have been developed for commercial use to date, latanoprost (approved in the USA in 1996), bimatoprost (approved in the USA in 2001), travoprost (approved in the USA in 2001) and unoprostone (currently not available in the USA). The chemical structures of the PGAs in commercial use in the USA are depicted in Figure 1.
Pharmacokinetics
Latanoprost and travoprost are prodrugs that undergo ester hydrolysis rapidly and quickly in the cornea to form their free acids.(24,27) Latanoprost acid and travoprost acid are potent and selective FP prostanoid receptor agonists.
The pharmacology of bimatoprost is contentious. One of the hypotheses proposed is that bimatoprost is a “prostamide” drug, in the category of endocannabinoid derivatives similar to anandamide, which has been shown to form a PGE2 ethanolamide derivative through the cyclooxygenase-2 pathway.(28) The hypothesis proposed is that bimatoprost does not undergo hydrolysis to its free acid and that its mechanism of action does not involve activation of the prostanoid FP receptor.(29) As compared to PGF2α, bimatoprost has extremely low activity at the human prostanoid FP receptor and to other prostanoid receptors.(26) A drug AGN 204396 has recently been postulated to be a “prostamide” antagonist(30) based on its ability to block the effect of bimatoprost on the feline iris, but not that of either latanoprost acid, travoprost acid or PGF2α. AGN 204396 has been reported to have minimal antagonist activity at the FP receptor, which leads to the hypothesis that it is an antagonist at the “prostamide” receptor.(30) Whereas initial studies purportedly failed to demonstrate activity at a wide range of other receptors, ion channels and transporters including cannabinoid receptors,(26) a recent study shows that bimatoprost does have some activity at the cannabinoid CB1 receptor.(31) Nevertheless, some investigators hypothesize that bimatoprost binds to a novel “prostamide” receptor, whose biochemistry and pharmacology have not yet been elucidated.(26,29)
Woodward et al(26) found that after topical application of bimatoprost in human volunteers, bimatoprost was detected in the blood whereas the free acid metabolite was not. The lower limit of detection of bimatoprost acid in this study was 25nM, which is higher than the concentrations required to activate the FP receptor. The same authors(26) also found that bimatoprost was more stable in iris-ciliary body tissue homogenates, with minimal conversion to bimatoprost acid as compared to latanoprost, which is rapidly and completely converted to its free acid metabolite. In Ussing chambers with in situ cadaveric corneal and scleral tissues, bimatoprost has been found to have better penetration through the sclera as compared to the cornea. Topical bimatoprost in cynomologous monkey eyes resulted in higher bimatoprost concentrations in the iris and ciliary body as compared to its concentration in the aqueous humor.(32) The authors(29) use this evidence to conclude that it is the bimatoprost amide, rather than the free acid, that is the active drug and that the amide drug lowers IOP by activating the “prostamide” receptor rather than the prostanoid FP receptor.
Other authors have disputed this theory by providing evidence that bimatoprost is a PGA much like latanoprost or travoprost. It is a prodrug that is converted in the cornea to its free acid (bimatoprost acid), which is known to be a potent agonist at the prostanoid FP receptors. The free acid of bimatoprost is chemically identical to the free acid of latanoprost apart from a double bond rather than a single bond between C13 and C14 (Figure 1). The conversion of bimatoprost to its free acid has been demonstrated in vitro in isolated bovine(33,34) and human corneas(33) and in other ocular tissues (sclera, iris/ciliary body) in rabbits and humans(35,36,37) and in vivo in rabbits and monkeys.(38) Aqueous humor analysis in human volunteers undergoing cataract surgery demonstrated a higher concentration of bimatoprost acid as compared to bimatoprost amide after topical instillation of bimatoprost pre-operatively (Table 1).(39,40,41) The concentration of bimatoprost acid in the aqueous humor after topical instillation of bimatoprost in humans is sufficient to explain its ocular hypotensive effect by activation of the FP prostanoid receptors.(39,40,41,42)
Table 1. Bimatoprost amide, bimatoprost acid and latanoprost acid concentrations (means) in the aqueous humor of patients treated with bimatoprost and latanoprost | |||||||||||||||||||||||||||||||||||||||||||||||||||||||||||||||
---|---|---|---|---|---|---|---|---|---|---|---|---|---|---|---|---|---|---|---|---|---|---|---|---|---|---|---|---|---|---|---|---|---|---|---|---|---|---|---|---|---|---|---|---|---|---|---|---|---|---|---|---|---|---|---|---|---|---|---|---|---|---|---|
|
Neither travoprost, latanoprost nor bimatoprost lower IOP in FP receptor knockout mice, providing further credence to the hypothesis that the FP receptor plays a critical role in the IOP-lowering effect of all these drugs.(43,44,45) The absence of an identifiable “prostamide” receptor, coupled with the proven ability of the human eye to hydrolyze bimatoprost to its free acid to produce biologically active concentrations in the aqueous humor, (Table 1) all seem to provide irrefutable evidence that topical bimatoprost acts through the FP prostanoid receptor like the other PGAs.(42)
The maximum aqueous humor concentration of the free acid after topical application of latanoprost 0.005% to human eyes in patients undergoing cataract surgery was 100 nM (33 ng/ml) at approximately 2.5 hours after administration and less than 1 nM (0.2ng/nl) at 24 hours (Figure 2).(24) The elimination half-life of latanoprost acid from the aqueous humor was 2.8 hours. Another study with a similar design demonstrated latanoprost acid aqueous humor levels of 29 nM at 1 hour, 41 nM at 3 hours and 3 nM at 6 hours after latanoprost instillation (Table 1).(40) The ester prodrug latanoprost was not detected in the aqueous humor at any point of time
Table 1 depicts the bimatoprost and bimatoprost acid concentration in the aqueous humor. Unlike latanoprost, bimatoprost is not 100% hydrolyzed to its free acid in the aqueous humor demonstrating that bimatoprost is an inefficient prodrug as compared with latanoprost. For this reason, bimatoprost is used topically at a much higher commercial concentration (0.03%) as compared to either latanoprost (0.005%) or travoprost (0.004%), so that sufficient concentrations of the free acid can be achieved. Thus, a higher concentration of the amide prodrug is required although the relative potencies of the free acids at the FP prostanoid receptors have been shown to be travoprost acid slightly greater than bimatoprost acid greater than latanoprost acid.(20,25,46,47) Despite these differences in potency, all three PGA acids are full agonists at the FP receptor as compared to the endogenous FP receptor agonist, PGF2α.
Endogenous PGs are autocoids, which exert a local effect in ocular tissues without metabolism followed by transport into the systemic circulation where they undergo rapid metabolism in organs such as the lungs and the liver. Following intravenous administration of radio-labeled latanoprost, the t1/2 of its acid was 14 minutes in plasma; following topical administration, the t1/2 of its acid was 17 minutes in plasma.(24) In patients on chronic latanoprost therapy for more than a year, the plasma concentrations of latanoprost acid were low enough to be undetectable in 50% of the patients studied. The plasma clearance of latanoprost acid is high, primarily due to liver metabolism. No latanoprost or latanoprost acid was found in the urine or feces. In a mass balance study using radio-labeled latanoprost, the majority of the radioactivity was recovered in the urine after both topical and systemic administration, mostly as metabolites of latanoprost.
Mechanism of Action
PGAs reduce IOP by enhancing aqueous outflow through the uveoscleral pathway and to a somewhat lesser extent through the conventional trabecular meshwork outflow pathway (Figure 2). They have little or no action on aqueous humor flow and episcleral venous pressure.
The conventional or the trabecular outflow pathway is a pressure-dependent route with aqueous draining sequentially through the trabecular meshwork, Schlemm’s canal, collector channels, episcleral veins, anterior ciliary veins, and into the systemic circulation. In the uveoscleral outflow pathway, the aqueous exits from the anterior chamber through the base of the iris or ciliary band, into the interstitial spaces between the ciliary muscle fiber bundles, into either the ciliary vasculature or alternatively into the suprachoroidal space and through the sclera to exit through the orbital lymphatics.
Our knowledge of the mechanism of action of PGAs in humans is derived from the noninvasive techniques of tonography or fluorophotometry. Neither of these methods delineates the anatomic pathways responsible for the enhanced outflow. These experimental methods allow us to determine a facility of outflow that is pressure dependent and to calculate a pressure independent outflow. The facility of uveoscleral outflow is assumed to be small or non-existent because the uveoscleral outflow pathway has been shown to be pressure-independent. The pressure-dependent outflow facility measurement is assumed to reflect the trabecular outflow. An intracameral tracer study in cats demonstrated a pronounced increase in uveoscleral outflow associated with an IOP reduction with no change in the uveoscleral facility after PGA2 instillation.(48) To our knowledge, this is the only study to date that specifically addresses the possibility of a PG induced effect on the facility of uveoscleral outflow. Discussions concerning the possibility of a PG-induced effect on the facility of uveoscleral outflow and the pressure-dependence of the uveoscleral outflow pathway have been published.(49,50,51,52,53,54) Further studies would be useful to resolve this issue.
The PGAs have been presumed to act predominantly by enhancing the uveoscleral outflow.(55) Several investigators have demonstrated that PGAs increase uveoscleral outflow in monkeys(56,57) and humans.(58,59,60,61) Further proof was found by demonstrating that topical pilocarpine physiologically blocks the IOP lowering effect of PGF2α in monkeys(62,63,64) because it induces contraction of the ciliary muscles, obliterates the intermuscular spaces and closes off the uveoscleral pathway.(65)
In addition to the numerous studies demonstrating enhanced uveoscleral outflow, several studies have shown an increase in the total outflow facility in monkeys and humans with topical latanoprost(58,59,66,67,68,69,70,71) as well as travoprost,(72) bimatoprost(60,61,73) and unoprostone.(74) Although the increase in total outflow facility might reflect increased pseudofacility (e.g. due to pressure induced reduction of aqueous flow during the measurement), uveoscleral outflow facility, ocular volumetric changes, or some combination thereof,(55,57) it likely reflects a concomitant change in the trabecular outflow facility.(49) Latanoprost has been shown to cause an increase in the aqueous vein flow, observed by real time microscopy, 30-60 minutes after instillation in 5 human volunteers, which provides direct proof that latanoprost affects the conventional trabecular outflow pathway.(75)
Most studies show that PGAs have little effect on aqueous humor flow.(58,59,60,67,71,72,74) A few studies have shown a slight initial increase in aqueous humor flow after PGA treatment.(61,73,76,77) In all the previously described studies, the PGAs appear to have no effect on episcleral venous pressure.
The effects of PGAs are mediated through binding to the prostanoid receptors in the eye. Naturally occurring PGF2α binds with its greatest affinity to the prostanoid FP receptor(78) although moderate binding also occurs with the EP3 receptor.(79) Travoprost acid, latanoprost acid and bimatoprost acid bind to the FP receptor with high affinity and show some affinity for the EP1 receptors and low affinity for the EP2 and EP3 receptor.(47) In monkeys, the FP receptor is found in the iris, ciliary body and the sclera.(80) In humans, the FP receptor and its mRNA were found to be widely distributed in ocular tissue with maximal concentrations found in the corneal epithelium, ciliary epithelium, the circular portion of ciliary muscle, and iris stromal and smooth muscle cells.(81) A single dose of latanoprost, travoprost or bimatoprost had no effect on IOP in FP receptor knockout mice as compared to wild type mice, demonstrating that the FP receptor plays a critical role in the IOP lowering ability of the PGAs.(43,44,45)
Studies in EP receptor knockout mice suggest that the EP3 receptor, but not the EP1 or EP2, may play a small role in the IOP lowering effects of the PGAs.(82) Pre-application of diclofenac inhibited the IOP response to PGAs in the wild type mice, but did not affect the IOP response in the EP3 knockout mice.(82) This finding suggests that the topical application of PGAs in mice can stimulate the production of endogenous PGs(83) some of which activate the EP3 receptors that contribute to the IOP reduction.
The FP receptors are classic G-protein-coupled receptors and activation increases inositol phosphate, protein kinase C and intracellular calcium.(84) FP receptor activation also results in the activation of the nuclear transcription factors c- Fos(85) and c- Jun.(86) These nuclear transcription factors form heterodimers that bind to AP-1 transcription regulatory elements found in the promoters of a large number of genes including the matrix metalloproteinases (MMPs).(87) FP receptor activation has been shown to induce MMP synthesis in ciliary muscle cell cultures(88) and in vivo in the ciliary body of monkeys.(89) Latanoprost acid has also been shown to increase gene transcription of MMP-1, MMP-3 and MMP-9 in a dose dependent manner in ciliary muscle cells in vitro.(90)
MMPs are enzymes typically associated with extracellular matrix (ECM) remodeling and turnover.(87) PGAs are hypothesized to lower IOP by widening and decompressing the connective tissue space in the ciliary muscle bundles by remodeling the ECM within the tissues of the uveoscleral outflow pathway(88,91,92) possibly due to the increased MMPs. Collagen types I and III are the major components of the fibrillar collagen found in the ECM of the interstitial spaces between ciliary muscle fiber bundles.(93) PGAs have been demonstrated to reduce the content of collagen types I, III, IV, VI, fibronectin, laminin, and hyaluronic acid in ciliary muscle cell cultures from humans(91) or cynomologous monkeys.(91,92) In vivo experiments with cynomologous monkeys have shown that topical treatment with PGAs resulted in significantly increased optically empty spaces between ciliary muscle bundles as compared to untreated and vehicle-treated control animals.(94) Latanoprost may also activate α2 ß1 integrin receptors that cause compaction of the ECM in the ciliary muscle.(95)
The trabecular meshwork also has FP(96) and EP prostanoid receptors(97) which are activated by the PGAs used in glaucoma therapy.(98) PGAs induce gene expression in isolated human trabecular meshwork cells.(99) The trabecular meshwork also expresses MMPs and tissue inhibitors of MMPs.(100,101,102) In tissue culture trabecular meshwork cells,(103) latanoprost increased the expression of several MMPs as well as tissue inhibitors of MMPs. In monkey eyes, topical treatment with PGAs resulted(94)in regional changes in the ultrastructure of the trabecular meshwork as well as changes in the ciliary muscle.
PGF2α has been shown to produce a dose-dependent relaxation of the carbachol contracted isolated monkey ciliary muscle although it has no effect on the resting muscle tension,(104) which has been hypothesized to explain the early drop in IOP after instillation of a single drop of a PGA.(57,104) Other investigators have shown that although PGE1, PGE2 and other synthetic PGAs may affect the contractile response in isolated animal ciliary muscle tissue, PGF2α and latanoprost acid had minimal if any effect on the contractile response.(105,106,107,108,109). The authors postulate that the EP2 and the DP receptors, but not the FP receptor, are involved in the inhibition of ciliary muscle contraction. The FP receptor, through its mediators, causes calcium mobilization, traditionally associated with muscle contraction as opposed to ciliary muscle relaxation.(55) A recent study demonstrates that bimatoprost showed a concentration dependent contractile effect in isolated human ciliary tissue much greater than that of latanoprost, travoprost or PGF2α.(31) This contractility was diminished by FP receptor antagonists and the cannabinoid receptor CB1 antagonist, providing further evidence that bimatoprost acts through the conventional prostanoid or cannabinoid receptors rather than through novel receptors that have yet to be cloned.
PGA induced vasodilatation leading to ciliary body tissue expansion is another hypothesis to explain the enhanced uveoscleral outflow. This theory is not supported by the finding that latanoprost has minimal if any vascular effects in the anterior uvea.(110,111) Latanoprost has been shown to cause reorganization of actin and vinculin in culture(91) and may alter the uveoscleral outflow by affecting the cytoskeleton of the ciliary muscle cells.
To conclude, current evidence demonstrates that the PGAs reduce IOP by activating FP receptors in the ciliary body, which activate a molecular transduction cascade, which leads to enhanced synthesis of MMPs. These MMPs may cause changes in the ECM of the uveoscleral pathway and enhance uveoscleral outflow. Similar changes probably also occur in the trabecular meshwork to affect conventional outflow. Other hypotheses include ciliary muscle relaxation, ciliary muscle vasodilation, or cytoskeletal re-organization (Figure 3). The IOP lowering effects of the PGAs do not appear to be mediated by changes in aqueous flow or episcleral venous pressure.
Efficacy
Latanoprost was the first PGA approved for clinical use in the USA in 1996 and amongst the PGAs, it is the drug that has undergone the most extensive clinical trials for efficacy, drug interactions and side effects. The initial clinical studies compared latanoprost to timolol, the former “gold standard” for glaucoma therapy. Several RCTs with crossover design reported that latanoprost 0.005% given once daily lowered IOP more effectively than timolol 0.5% given twice a day.(112,113,114,115,116,117,118,119) Pooling data from 8 different clinical trials in a heterogeneous worldwide population from eight different countries and four different races in Asia, Europe, North America and South America, Hedman and Larsson(120) studied 1389 primary open angle glaucoma (POAG) or ocular hypertensive (OHT) patients and found that latanoprost 0.005% given once daily (n=652) reduced IOP by 7.9 mm Hg as compared to 6.4 mm Hg with timolol 0.5% given twice daily (n=737). Pooled data analysis of phase III clinical trials from Scandinavia, the UK and the United States have shown that latanoprost reduced the mean IOP by 7.7 mm Hg (31% reduction of IOP) as compared to timolol 0.5%, which reduced the IOP by 6.5 mmHg (26%) after 6 months of double masked treatment.(121) A meta-analysis of 11 RCTs comparing latanoprost and timolol showed that latanoprost reduced IOP by 30% and timolol reduced IOP by 27% at 3 months(122). Van der Valk(123) et al performed a meta-analysis of 28 clinical glaucoma trials with 8 different drugs. These trials represented a combined number of 6953 patients for the IOP trough and 6841 for the IOP peak. Figure 4 depicts the relative changes in IOP from baseline after glaucoma therapy.(123) As is evident from the figure and the overlapping confidence intervals, the PGAs are the most efficacious class of drugs for lowering IOP and no statistically significant difference exists amongst the 3 PGAs. Several RCTs, comparing the 3 PGAs, have also found no significant difference in the IOP lowering efficacy of latanoprost, bimatoprost and travoprost.(124,125,126)
![]() Figure 4. Meta-analysis results for relative change in IOP from baseline, for peak and trough time points from 28 randomized clinical trials (n= 6953 for IOP trough and 6841 for IOP peak). |
Latanoprost and other PGAs yield a high rate of response for IOP reduction. Different clinical trials report varying rates depending on their definition of response. Rossetti et al defined nonresponse with latanoprost as IOP lowering less than 15% at 1 month and found a 4.1% rate of nonresponse.(127) Camras and Hedman,(128) using the same definition, found a rate of nonresponse at 3 months of 18% with latanoprost and 28% with timolol. Of these nonresponders, 26% of the latanoprost group and 6% of the timolol group were labeled responders at each of the other 4 visits (2 weeks, 6 weeks, 4.5 months and 6 months after therapy was initiated). Hedman et al, in their pooled data analysis of 2 phase III trials,(129) defined “IOP treatment failure” after treatment with latanoprost as insufficient IOP reduction as judged by the investigator. With this “soft” endpoint they found an 8% overall risk of treatment failure with latanoprost. The risk of treatment failure increased with increasing baseline IOP and the diagnosis of POAG as compared to OHT (probably due to lower IOP targets in patients with POAG). Only 6% of patients were withdrawn during the 2-year follow up due to insufficient IOP reduction.
PGs are thought to act at least partially by the reorganization of the ECM of the ciliary muscle (see Mechanism of Action section). This might theoretically lead to a slow increase in efficacy over time. Hedman et al (129) found that in most eyes, the full effect of latanoprost was reached in 2-8 weeks and that the IOP was not significantly different when assessed at 2 weeks compared to 1 year of treatment with latanoprost. A pooled data analysis of 1389 glaucoma patients found that 26% of the 713 latanoprost treated patients showed an increase in treatment response from 2 weeks to 3 months. (120) However, 16% of the same patients also showed a decline in IOP response over the same period. Random variation in IOP may account for at least some of this variability.(128) Camras and Hedman found that patients treated with latanoprost had a significantly lower IOP at 6 months as compared to 2 weeks of treatment. They also found that of all the patients defined as non-responders (IOP reduction <15%) at 2 weeks, 90% were defined as responders on at least one subsequent visit.(128)
Unlike timolol, latanoprost reduces IOP on a circadian basis with no significant difference in the IOP lowering effect during the nocturnal hours as compared to the diurnal hours.(130,131,132) Bimatoprost and travoprost(125,132) are also effective on a circadian basis.
Several dose-response studies were performed with latanoprost. The commercial concentration of latanoprost 0.005% was chosen as it was near the top of the dose response plateau for IOP reduction.(133,134) Increasing the concentration gave rise to a small increase in IOP reducing efficacy but also caused increased conjunctival hyperemia. Once daily latanoprost demonstrates greater IOP lowering efficacy as compared to twice daily latanoprost,(115,135,136,137,138) perhaps because twice daily administration results in desensitization of the FP receptor-signal transduction system in the target cells. Similarly, once daily administration of bimatoprost(139,140,141,142) is more efficacious than twice daily administration. All three PGAs are used once daily.
Latanoprost may be more effective when administered once daily in the evening than in the morning(114) although others(143,144) have found no significant difference between morning and evening administration of latanoprost. A meta-analysis of 5 randomized controlled trials (RCT) showed that the evening dosage was more effective than the morning dosage of latanoprost(122) although IOP efficacy was assessed during the daytime rather than the nocturnal hours in all these studies.
An important aspect of the overall efficacy of glaucoma drugs is persistency or the ability of glaucoma patients to be compliant with their glaucoma medications. PGAs with their once daily dosing and lower systemic side effects should theoretically have higher persistency rates than other classes of glaucoma medications. Several studies have proven that patients treated with PGAs demonstrate greater persistency than those treated with other ocular hypotensive medications.(145,146,147,148,149) A recent study using health insurance claims data found a persistence rate of 69% for latanoprost, 71% for travoprost, and 68% for bimatoprost, and an average adherence rate to all the PGAs of 76% over a period of 12 months.(150) An Australian study used national pharmacy data to also conclude that the PGAs and the the timolol-dorzolamide fixed combination had the maximum persistency as compared to other classes of glaucoma medications(151)
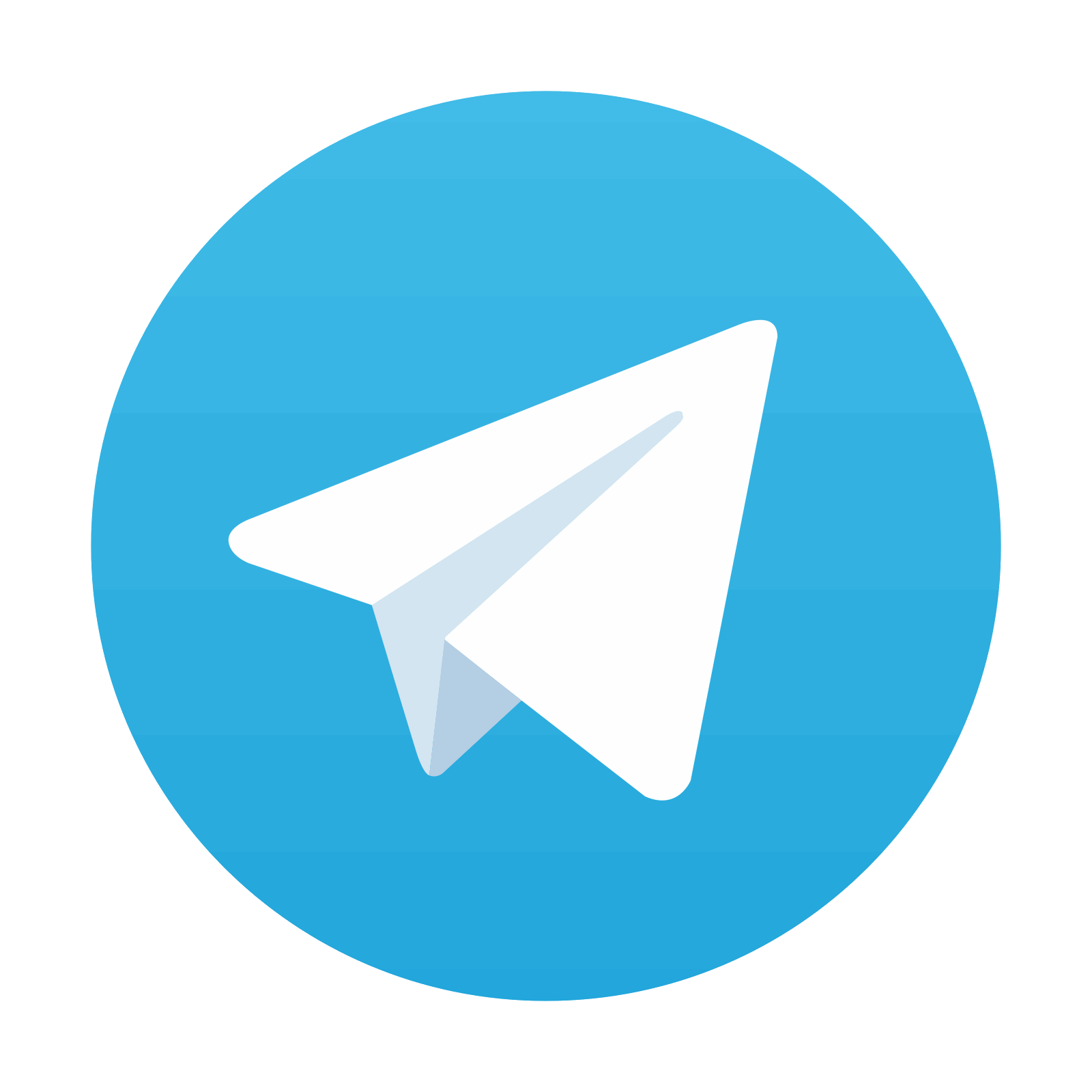
Stay updated, free articles. Join our Telegram channel

Full access? Get Clinical Tree
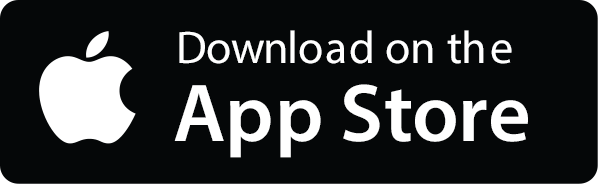
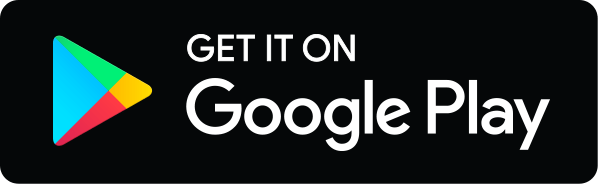