Systemically and Topically Administered Carbonic Anhydrase Inhibitors in the Treatment of Glaucoma
Janet B. Serle
Milan P. Ranka
Carbonic anhydrase inhibitors (CAIs) have been used to treat glaucoma since 1954, when Becker1 first demonstrated the clinical efficacy of systemically administered acetazolamide. CAIs are sulfonamide derivatives that lower intraocular pressure (IOP) by decreasing aqueous humor formation. These drugs inhibit carbonic anhydrase (CA), one of the enzymes that regulate aqueous humor formation. Systemic administration of CAIs is extremely effective in reducing IOP in most patients but is accompanied by side effects that can range from mild and annoying to debilitating and life threatening, the latter necessitating discontinuation of these drugs. On rare occasions, CAIs may induce irreversible blood dyscrasias, which can be fatal. The substantial incidence of side effects and the rare occurrence of fatal complications have led to much debate about the use of and indications for chronic administration of systemically administered CAIs in the management of glaucoma. The numerous side effects led to an ultimately successful 40-year search for topically effective CAIs. During the mid 1990s, the first effective topical CAI, dorzolamide, was approved for clinical use. Subsequently brinzolamide, another topical CAI, was approved for clinical use in 1998. Topically applied CAIs are well tolerated, with few ocular side effects, and are not associated with the systemic side effects encountered with the use of systemically administered CAIs.
Historical Perspective
In his 1949 Proctor Award Lecture of the Association for Research in Ophthalmology, Friedenwald2 initially theorized that aqueous humor formation was an active process involving transport into the posterior chamber of electrolytes and ascorbic acid and requiring large quantities of HCO3- as a buffer. Subsequently, Kinsey3 demonstrated a high concentration of HCO3- in the aqueous humor relative to plasma in rabbits, and Wistrand4 indirectly demonstrated a high concentration of the enzyme CA in extracts of rabbit iris and ciliary body tissues. These findings supported Friedenwald’s theories.
The enzyme CA had been previously identified in the kidney. Under the direction of Richard Roblin at the American Cyanamid Company, Maren5 was searching for a CAI to use as an oral diuretic agent and was involved in the synthesis of acetazolamide in 1954. Becker1 assimilated the findings of high concentrations of HCO3- in the posterior chamber of the eye, the presence of CA in ciliary body tissues and in the kidney, and the possible similarity of secretory mechanisms in the eye and the kidney; he elected to evaluate acetazolamide as an ocular hypotensive agent in glaucoma patients. Becker’s demonstration of the marked ocular hypotensive effect of acetazolamide in glaucoma patients led to the widespread clinical use of oral CAIs for the treatment of glaucoma and to numerous clinical and laboratory evaluations of acetazolamide and other CAIs. The discovery of the substantial effect of acetazolamide on IOP encouraged Maren to extensively research the chemistry, physiology, and pharmacology of CAIs.
Subsequently, in the 1980s, Sugrue,6,7 a pharmaceutical chemist working at Merck & Co., Inc., modified the chemical structure of a series of thienothiopyran-2-sulfonamide derivatives and discovered dorzolamide. He demonstrated its effectiveness in inhibiting the enzyme CA II in vitro and its efficacy in lowering IOP in in vivo preclinical investigations. Dorzolamide is the first topically active and well-tolerated CAI to be used clinically. Brinzolamide, a drug with similar structural and binding effects at the enzyme CA II, was developed by Alcon Pharmaceuticals Inc. and approved for clinical use in 1998.
Carbonic Anydrase
The enzyme CA is a soluble, single-stranded, nearly spherical protein with a molecular weight of 30 kd. At the center of the active site, it has a zinc atom linked to three histidine molecules and water.8 These zinc metalloenzymes are inhibited by aromatic sulfonamides containing an unsubstituted R-SO2-NH2 group. The R-SO2-NH2 sulfonamide group exists at body pH in equilibrium with the anionic form R-SO2-NH-. It is the R-SO2-NH- anion that attacks the zinc active site of CA, replacing OH- and inhibiting the enzyme. Any substitution onto the terminal amino group, such as occurs in the antibacterial sulfonamides, renders the compound impotent as a CAI.
CA catalyzes the reversible reaction in which carbon dioxide (CO2) is hydrated (H2O) to form carbonic acid (H2CO3) (i.e., CO2 + H2O⇔H2CO3) in the ciliary epithelium as well as in other tissues in the body. H2CO3 dissociates into bicarbonate (HCO3-) and hydrogen ion (H+). In the eye, HCO3- then passes into the posterior chamber along with sodium ions (Na+). This movement of HCO3- and Na+ draws copious amounts of fluid along with it, thus producing aqueous humor. Although this reaction proceeds in a reversible fashion in the absence of a catalyst, the presence of the enzyme CA provides an enhancement of the reaction rate by more than 100-fold.9
Sixteen isoenzymes of CA have been identified in mammals.10 Several of these isoenzymes (CA I, II, IV, V,VII, and XIV) have been isolated in the eye.11,12,13 CA II, an intracellular enzyme, is the most widely distributed CA isoenzyme in the eye and is thought to be the predominant isoenzyme responsible for controlling aqueous humor production.12 CA II is especially abundant in pigmented and nonpigmented epithelial cells of the ciliary processes,11,12 in Müller cells of the retina, and in a subset of cone photoreceptor cells.11
In addition to ocular tissues, CA has been found in red blood cells,14 the renal tubules,15 gastric mucosa, salivary glands, the pancreas, and in several sites in the brain including neurons, glia, and in cells responsible for the production of cerebrospinal fluid.16
Systemically, CA plays a role in two major physiologic functions, respiration and urine formation. In red blood cells within tissue capillaries, CO2 is hydrated to form H2CO3 (CO2 + H2O⇔H2CO3). This reaction, when catalyzed by CA, proceeds to the right 13,000 times faster than the uncatalyzed reaction. As the erythrocyte proceeds from tissue capillaries to pulmonary capillaries, H2CO3 is converted to H2O and CO2 (H2CO3⇔CO2 + H2O). In pulmonary capillaries, CO2 then diffuses across the red cell membrane into the plasma and then across the alveolocapillary membranes into alveolar gas. Thus, CA plays a critical role in the removal of the body’s main metabolic by-product, CO2.
In the proximal and distal renal tubules of the kidney, the enzyme CA facilitates the production of H+, which acidifies the urine. In the kidney, the catalytic hydration of CO2 generates H2CO3, which dissociates into H+ and HCO3-. The H+ is excreted into the urine, and the HCO3- diffuses back into the plasma. By inhibiting CA, H+ excretion is greatly decreased, thus producing a mild metabolic acidosis. A second result of the decrease in H+ excretion in the urine is the loss of Na+ in the distal tubule in lieu of H+. In patients who do not have renal disease, total body levels of Na+ and potassium (K+) are initially decreased by up to 10%.17,18 At this point, however, a new equilibrium is reached, as the acidosis forces the reaction to the left (CO2 + H2O ⇔ H2CO3), ensuring no further loss of these electrolytes.18,19
Thus, CA inhibition can produce a mixed respiratory and metabolic acidosis.8 This acidosis occurs as a consequence of (a) accumulation of tissue CO2 and reduced concentration of CO2 in expired air and (b) decreased H+ excretion by the kidneys.20 This acidosis is compensated for by hyperventilation in patients who do not have compromising pulmonary disease, which ensures a sufficient release of CO2.21
Mechanism of Intraocular Pressure Reduction
The reduction in IOP caused by CAIs is primarily due to local enzyme inhibition, thenzyme CA II must be inhibited by >99% in order to reduce aqueous humor formation22,23 and in part may be due to systemic acidosis.24,25,26,27 Studies on the effects of acetazolamide on nephrectomized animals have suggested that IOP could be reduced in the absence of systemic acidosis.24,28,29 In some clinical evaluations, the degree of IOP reduction after acetazolamide or methazolamide administration in the absence of acidosis is less than that seen in conditions of acidosis.30,31 In other studies, the degree of acidosis does not seem to affect the IOP response.22,32 CAIs reduce IOP by inhibiting aqueous humor formation. This has been demonstrated with the use of indirect measurement techniques24,33 as well as direct fluorophotometric measurement techniques of aqueous humor flow rates.32,33,34 Fluorophotometric studies with systemically administered CAIs have documented reductions in aqueous humor flow rates of 21% to 40% in normal human volunteers and in a few glaucoma patients after acute oral dosing with 250 to 750 mg acetazolamide.34,35,36 McCannel and associates36 found that acetazolamide reduced aqueous flow rates by 24% below the nocturnal rate in the eye of a sleeping patient; timolol produced no such reaction. Aqueous flow measurements performed during the day suggested that acetazolamide and timolol 0.5% are additive after acute dosing.35,36 Outflow facility is unaltered by CAIs.1,33
Clinical Uses of Systematically Administered Carbonic Anydrase Inhibitors
Numerous clinical evaluations of systemically administered CAIs have been performed. Very few have been conducted in masked, randomized fashion, and the number of subjects participating in these studies has been small. The limitations of the study designs allow us to make some generalizations about CAIs, but the conclusions of many of these trials should be evaluated critically. Much of the information the authors cite about systemically administered CAIs is anecdotal and based on small numbers of patients who are taking additional ocular hypotensive agents.
Three oral CAIs are commercially available: acetazolamide (Diamox), methazolamide, (Neptazane), and dichlorphenamide (Daranide). The longest clinical experience has been with acetazolamide, as this drug was the first to be synthesized and has been used clinically since 1954.1
Two different single-dose dose–response studies37,38 using oral administration of 63, 125, 250, or 500 mg acetazolamide suggested mild enhancement of the IOP effect with increasing dosages. The onset of IOP reduction began 1 hour after dosing, and maximum IOP reductions occurred 2 to 5 hours after dosing and ranged from 30% to 41%. The duration of the IOP effect of single doses of orally administered acetazolamide is 6 to 8 hours. The dose–response characteristics and duration of action of acetazolamide support the common clinical regimen of four times daily dosing with acetazolamide tablets. Patients can be started on a low dosage of acetazolamide, such as 62.5 mg or 125 mg four times daily and advanced to 250 mg four times daily as necessary and tolerated. Dosages are generally limited to a total of 1,000 mg daily, because a higher dosage is associated with more frequent and severe side effects. The dosage in infants and children is 5 to 10 mg/kg administered every 4 to 6 hours.39 The tablets can be crushed and added to foods (e.g., applesauce) or juices.
An alternative formulation of acetazolamide tablets is acetazolamide 500 mg sustained-release capsules. The onset of IOP reduction is 1 to 2 hours after single-dose capsule administration.38,40 Maximum IOP reductions occur 6 to 18 hours after dosing, with durations of up to 23 hours.38,40,41 Twice-daily dosing with acetazolamide sustained-release capsules caused greater reductions in the magnitude of IOP reduction than once-daily dosing.38,41 Twice-daily dosing with 500 mg sustained-release capsules caused IOP reductions of a magnitude similar to that caused by 250 mg acetazolamide tablets administered four times daily.41 The advantages of prescribing sustained-release capsules include the following: (a) patient compliance is enhanced with twice-daily dosing compared with four times daily dosing; and (b) some patients are purported to be able to tolerate the capsules better than the tablets, experiencing fewer side effects.40,42
Acetazolamide can be administered intravenously (IV) in doses of 250 to 500 mg if acute, rapid IOP reduction is imperative, as the onset of IOP reduction begins within 2 minutes of IV administration.43,44 IV dosing can be repeated in 2 to 4 hours, or an oral CAI can be administered.
Methazolamide was initially evaluated in patients in 1960.45 The chemical structure is similar to acetazolamide, but methazolamide has a plasma half-life three times that of the nonsustained-release form of acetazolamide: 14 hours versus 5 hours.46 Fifty-five percent of methazolamide is bound to plasma proteins compared with 93% of acetazolamide, allowing for enhanced diffusion of methazolamide into the tissues. Thus, lower and less frequent doses of methazolamide can be used as opposed to acetazolamide; however, clinical evaluations of methazolamide using doses of up to 450 mg daily have suggested that the drug was not as effective as daily doses of 500 to 2,000 mg of acetazolamide.31,32,45 The difference in efficacy of these two drugs has been explained in part by the findings of absence of systemic acidosis encountered with low doses of methazolamide46 and milder systemic acidosis with higher doses of methazolamide.45 Methazolamide doses of up to 50 mg three times daily caused less severe metabolic acidosis than acetazolamide in doses as low as 62.5 mg four times daily.46 Systemic acidosis may be one of the explanations for the greater incidence of side effects reported with acetazolamide than with methazolamide.31,32,45 Unlike acetazolamide, methazolamide is neither secreted nor concentrated by the kidney.
Dichlorphenamide is available in 50-mg tablets. It has a side effect profile similar to acetazolamide because of its potential to induce metabolic acidosis47 and reduce urinary citrate excretion. It is usually prescribed in doses of 50 to 100 mg every 6 to 8 hours.
Anecdotal information suggests that a small number of patients intolerant to one CAI may be able to tolerate one of the other available CAIs.40,45
The numerous side effects of systemically administered CAIs and the availability of effective topical CAIs has reduced the clinical use of and altered the indications for prescribing oral CAIs. Oral or systemically administered CAIs are effective for managing acute IOP elevations, such as during subacute or acute attacks of angle-closure glaucoma, in patients who need IOP reductions while awaiting surgery, in patients where topical administration of medications is not feasible, and in patients who develop ocular or skin irritation with topical administration of CAIs.
Oral Carbonic Anhydrase Inhibitors and Systemic Side Effects
Despite the well-documented IOP-lowering effects of oral CAIs, their use is limited by side effects. As mentioned, the side effects of orally administered CAIs can range from mild and annoying to debilitating and life threatening.
Calcific renal calculi have been documented in patients treated with CAIs. In a retrospective case-control study, Kass and colleagues48 demonstrated that the rate of developing one or more kidney stones per year during acetazolamide therapy was 11 times higher in the study group than in the control (untreated) group. Therapeutic doses of orally administered acetazolamide 250 mg four times daily,32,49,50 methazolamide 100 mg three times daily,46 or dichlorphenamide 50 mg four times daily or 100 mg three times daily50 decreased urinary citrate excretion by up to 86% compared with pretreatment values. Significantly less reduction of urinary citrate excretion, however, was induced with oral methazolamide 25 mg three times daily.32,46 The overall incidence of developing renal stones while on methazolamide seems to be less than with acetazolamide, which may be due to the lower doses of methazolamide that are most commonly used.45,51,52 CAIs induce urolithiasis by reducing urinary excretion of citrate. Citrate chelates urinary calcium salts into a soluble complex and prevents stone formation. In the absence of citrate, calcium is not adequately solubilized and serves as a nidus for stone formation.
A relative hypokalemia and hyponatremia can occur with chronic CAI use.17,21,53,54 These effects are usually self-limited, as is the diuretic effect of CAIs.17,55 The hypokalemia is usually not of clinical significance unless the patient is concurrently using a K+-depleting medication, such as a hydrochlorothiazide diuretic. K+ supplementation is generally not indicated in the majority of patients taking CAIs alone.53 Additionally, K+ supplementation does not alleviate the CAI-associated side effects in patients who are not taking concurrent K+-depleting drugs.56 If the patient is on a K+-depleting drug, serum K+ levels should be followed and supplementation prescribed as indicated.
A mild mixed respiratory and metabolic acidosis can occur with chronic use of oral CAIs. The acidosis may contribute to the malaise and fatigue often encountered with chronic CAI use.56 Systemic acidosis, as a consequence of CO2 retention, may contribute to acute respiratory failure in patients with severe chronic obstructive pulmonary disease. These patients are unable to increase their minute respiratory volume in order to compensate for the acidosis.47,57,58,59 Acute systemic acidosis can precipitate sickling in patients with sickle cell trait or disease, and therefore CAIs should be avoided in these patients.60
The likelihood of salicylate toxicity in patients taking high doses of aspirin may be increased with concurrent CAI use because of CAI-related systemic acidosis.61 Salicylate toxicity is related to tissue rather than to blood salicylate levels.62 Nonionized salicylic acid passes from the bloodstream into the central nervous system and other cells more rapidly than does ionized salicylate. Systemic acidosis increases the proportion of nonionized drug63 and thus increases the quantity of salicylate entering the central nervous system and other tissues, leading to toxicity.
Liver failure, characterized by confusion, asterixis, and elevated blood ammonia, may be seen in patients with chronic liver diseases. The mechanism by which CAIs cause liver failure remains unclear.
Teratogenicity has been demonstrated in laboratory animals and anecdotally in humans receiving systemically administered CAIs.64,65,66,67 As a consequence, female patients planning a pregnancy should be counseled about the potential teratogenic effects, and consideration should be given to switching to another ocular hypotensive regimen before pregnancy.
Blood dyscrasias, including aplastic anemia, agranulocytosis, thrombocytopenia, and neutropenia, have been documented in patients taking systemically administered CAIs.68,69,70,71 Blood dyscrasias may be idiosyncratic, occurring after as little as a single dose of medication, or they may be dose related. The National Registry of Drug-Induced Ocular Side Effects received 79 case reports of suspected hematopoietic toxicity caused by CAIs occurring between 1972 and 1984.72 These adverse hematologic reactions occurred in 68% of patients within 6 months of initiating therapy. Thirty-two percent of these cases resulted in death secondary to aplastic anemia. These severe adverse experiences have engendered considerable debate in the literature as to the advisability of routine monitoring of the complete blood counts of patients on CAI therapy. It has been suggested that patients administering oral CAIs be monitored every 6 months.71,72,73 Unfortunately, the majority of the hematologic complications reported occurred before 6 months of therapy. Thus, monitoring every 6 months would not have been soon enough to detect the blood dyscrasias. Of greatest benefit to the individual patient is probably routine careful questioning about anemia, infection, and poor clotting, with follow-up as indicated.74
The following side effects are less serious than those described above 68,69,70,71,72,73, but they tend to be much more frequent and can be particularly annoying to the patient:
A symptom complex of malaise, fatigue, weight loss, depression, anorexia, and often loss of libido has been reported in up to 48% of patients. The patients in one study56 who complained of this symptom complex were more acidotic than those without such complaints.
Paresthesias in the proximal and distal extremities have been reported in up to 75% of patients.75 This side effect usually does not necessitate the discontinuation of the drug and tends to diminish with chronic use.
Maculopapular and urticarial skin eruptions have been reported in patients using methazolamide.76
Patients may complain of strange tastes occurring during consumption of carbonated beverages. Similar complaints have been elicited when CA has been topically dispensed on the tongue.
Development of Topically Active Carbonic Anhydrase Inhibitors
Since the 1950s, numerous investigators77,78,79,80,81 have attempted to formulate a topically active CAI that would have fewer side effects than the systemic formulations. The systemically administered CAIs that are effective ocular hypotensive agents (e.g., acetazolamide, methazolamide, ethoxzolamide, dichlorphenamide) were relatively ineffective when administered topically. Modifications of these systemically active agents that were evaluated for topical use include trifluoromethazolamide, a derivative of methazolamide that reduced IOP in rabbits77,78 but was unstable in solution and required a long duration of ocular exposure; aminozolamide gel, an analogue of ethoxzolamide that significantly reduced IOP in patients with ocular hypertension79 but had substantial ocular side effects when administered chronically;80 and L-650,719, another sulfonamide derivative that reduced IOP in rabbits81 but failed to reduce IOP in a clinical trial of normotensive volunteers.82
In the 1980s, a series of thienothiopyran-2-sulfonamide compounds were developed that are effective in reducing IOP when administered topically. These compounds are sulfonamide derivatives,83,84,85 as is acetazolamide, and they differ from other CAIs previously investigated for topical use in that they are water soluble, are stable in solution, have excellent corneal penetration, and inhibit CA at low concentrations, which can be achieved with topical application.
Three thienothiopyran-2-sulfonamide derivatives were identified that reduced IOP after topical administration in laboratory and clinical trials: MK-927, a racemic mixture (Fig. 1); sezolamide (MK-417), the S-enantiomer of MK-927 (Fig. 2); and dorzolamide, a compound structurally similar to MK-927 and sezolamide. During the development of dorzolamide, it was initially identified as L-671,152 and then as MK-507 (Fig. 3).
![]() Figure 2. The chemical structure of MK-927 and sezolamide. MK-927 is the racemic mixture of the compound shown. Sezolamide is the S-enantiomer. |
MK-927
Rabbit Studies
MK-927 was the first of the three thienothiopyran-2-sulfonamide derivatives to be identified and extensively evaluated. Studies in normal rabbits demonstrated that the topical application of MK-927 inhibited CA in homogenized iris ciliary body tissues84 and reduced IOP for at least 6 hours.85 IOP was reduced for a longer duration in pigmented rabbits than in albino rabbits,86 possibly because pigment binding of the drug may have caused a depot effect. A dose–response study in albino rabbits demonstrated that 2% MK-927 was at the top of the dose–response curve, producing the longest duration and the largest magnitude of effect on IOP.86 In unilateral alpha-chymotrypsinized glaucoma-induced rabbits, MK-927 reduced IOP unilaterally when applied to the normal eyes but did not affect the contralateral, untreated hypertensive eyes. These data provided support for the local rather than systemic effect of MK-927.
Primate Studies
Single-dose and multiple-dose testing of MK-927 was performed in cynomolgus monkeys in which ocular hypertension had been created by multiple sessions of high-power argon laser trabeculoplasty. A single-dose dose–response study demonstrated that 2% MK-927 was at the top of the dose–response curve.86 IOP was significantly reduced 1 to 6 hours after drug application, with a mean peak reduction of 9.6 mm Hg. Twice-daily application of 2% MK-927 for 5 days to eight glaucomatous monkeys reduced IOP 1 to 8 hours after each dose.87 Maximum reductions in IOP occurred 3 hours after dosing and increased from a mean of 10.1 mm Hg on day 1 to 13.2 mm Hg on day 5, suggesting enhancement of effect with repetitive dosing.
Clinical Studies
The first clinical trials (Table 1) in normal volunteers evaluated three doses of 2% MK-927 administered to ten volunteers and three doses of placebo administered to two volunteers.88 IOP was reduced by 30% in the MK-927 treated eyes and by 7% in the fellow eyes, and it was unchanged in the placebo-treated eyes. A similar study in patients with glaucoma or ocular hypertension demonstrated mean IOP reductions of 27% in the 2% MK-927–treated eyes and 11% in the placebo-treated eyes 6 hours after drug instillation.89 A single application of 2% MK-927 to patients with elevated IOP reduced the pressure by 33% in the drug-treated eyes and by 17% in the placebo-treated eyes at 4½ hours after dosing.90
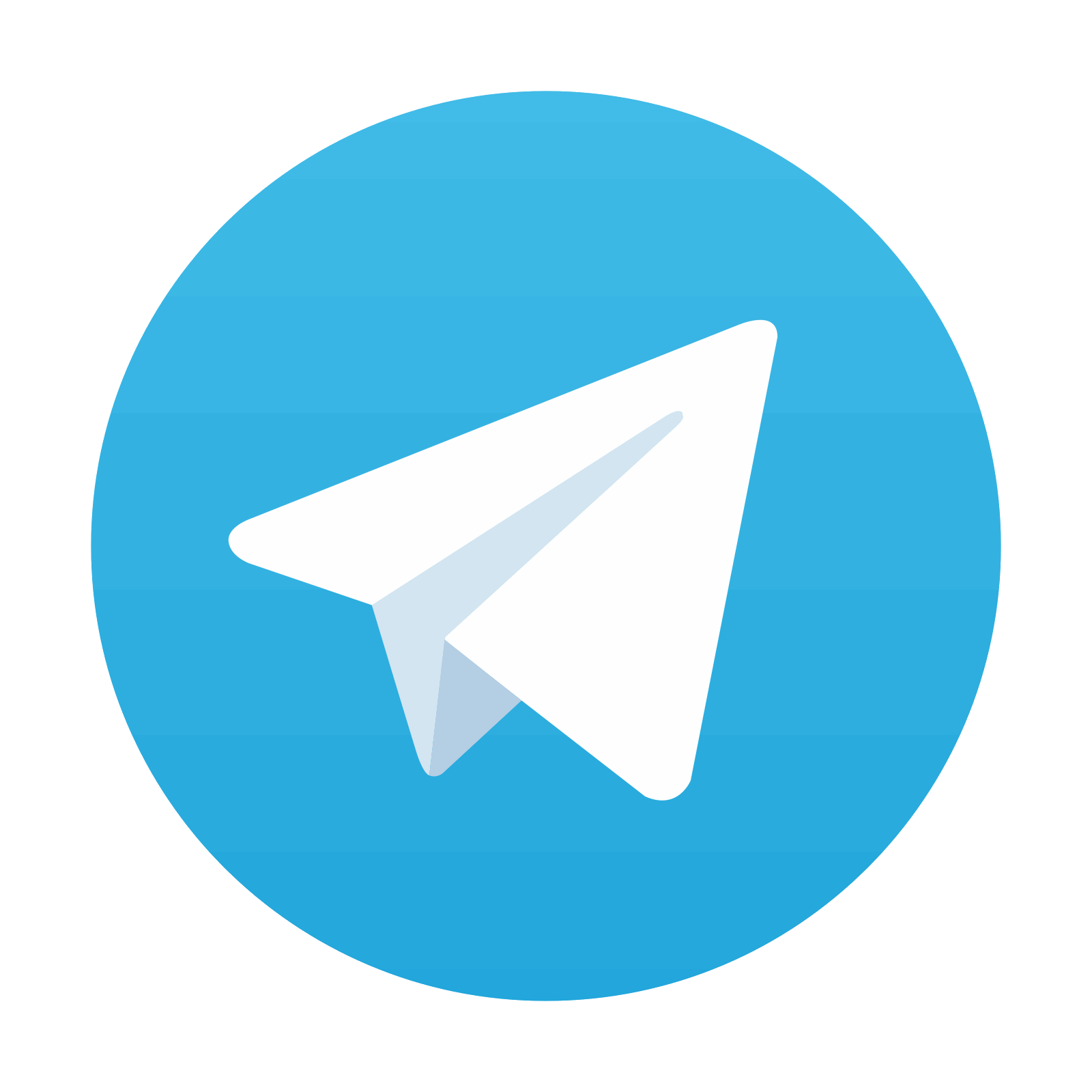
Stay updated, free articles. Join our Telegram channel

Full access? Get Clinical Tree
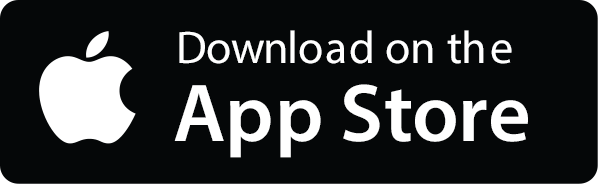
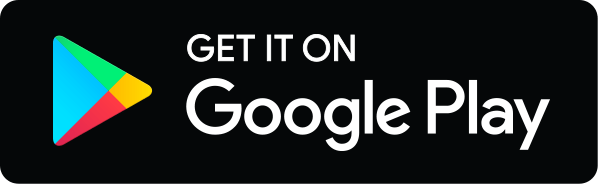