Chapter 65 Stereotactic Radiosurgery of Skull Base Tumors
Gamma knife surgery, similar to microsurgery, has advantages and disadvantages which must be thoroughly discussed with the patient.1,2 For the patient it is alluring to undergo an outpatient procedure rather than microsurgical management that requires a much longer period of care. Further, gamma knife outcomes show excellent tumor control and, with current methods, low cranial nerve morbidity. Gamma knife surgery is a viable treatment modality for the appropriate patient as defined by age, medical history, tumor characteristics and physical findings. As such, many neurotologists now offer gamma knife surgery as part of their armamentarium for managing vestibular schwannomas and glomus tumors.3
PREOPERATIVE COUNSELING
Informed consent for gamma knife surgery requires the surgeon to discuss alternative options such as observation and microsurgical resection.2 The risks and benefits of these alternatives should be frankly described and compared to gamma knife treatment. Many patients have received information from the Internet or from physicians with limited experience with gamma knife and may have erroneous information. Common misconceptions include the expectation that gamma knife surgery completely removes the tumor and that hearing will improve, or conversely that cranial nerve morbidities are significant. These need to be addressed with evidence-based reports and information.
One statistic, which is particularly alarming to patients considering gamma knife surgery is that there have been eight cases of malignancy within vestibular schwannomas (as of 2002).4 Four of these patients had been previously treated with radiosurgery. While it remains possible that these four malignancies developed after the radiation treatment, it is more likely that these malignant tumors were misdiagnosed as benign at the outset of evaluation and treatment.
Delayed development of radiation-induced neoplasms was addressed by Pollock and colleagues in 1998.5 They reviewed more than 20,000 patients treated with radiosurgery worldwide and found no increased incidence of new neoplasm development (i.e., benign or malignant). A retrospective cohort study comparing the Sheffield, England radiosurgery patient database with the national mortality and cancer registries identified a single new astrocytoma among those treated.6 Based on their national incidence figures, 2.47 cases would have been predicted. The risk of radiosurgery induced malignancy in patients with neurofibromatosis type 2 (NF2) and von Hippel-Lindau disease was similarly studied.7 Of 118 NF2 and 19 von Hippel-Lindau disease patients, totaling 906 and 62 patient-years of follow-up data, respectively, only two cases of intracranial malignancy were found. Both of these were in NF2 patients. One was thought to have arisen before the radiosurgery; the other was a glioblastoma diagnosed three years after radiosurgery. Gliomas may occur in as many as 4% of NF2 patients and the single case may not represent an increased risk. It was suggested that the late risk of malignancy arising after irradiation must be put in the context of the condition being treated, the treatment options available to these individuals, and their life expectancy.
Despite the findings of the studies just reviewed, it is important to counsel patients about the possibility of malignant transformation or induction. A handful of tumors suggestive of radiation induced malignancy have been reported among the tens of thousands who have undergone gamma knife treatment. Lustig and colleagues reported the development of a squamous cell carcinoma following radiation treatment of vestibular schwannoma.8 Hanabusa and colleagues reported the malignant transformation of a vestibular schwannoma following gamma knife surgery.9 There was histologic evidence of vestibular schwannoma following a retrosigmoid resection. Four years after this resection, recidivistic tumor was identified, and the patient was subsequently treated with gamma knife surgery. Six months post-treatment, the tumor had grown, and the patient underwent surgical resection via a combined retrosigmoid-translabyrinthine approach. Abnormal mitotic figures were observed on histologic sections, and the diagnosis of malignancy was assigned.
SURGICAL TECHNIQUE
The Gamma Knife Unit
The basic principle of gamma knife surgery is to provide focused radiation to the tumor while minimizing radiation delivery to surrounding tissues. As such, a semicircular shield called the collimator helmet is used to generate approximately 200 individual gamma radiation “beams.” In the center of the helmet, where the beams meet, radiation delivery is maximal, but along each individual radiation tract tissue exposure is relatively low. When the collimator helmet is locked into position, the 201 openings of the collimator helmet coincide with the cobalt sources. There is a shielded chamber within which the 60Co sources are contained, and stainless steel shielding doors protect the treatment room from the 60Co sources. There is a treatment couch with an adjustable mattress that slides into the gamma knife unit together with the collimator helmet and the patient. Figure 65-1 schematically shows the orientation of the components of the gamma knife model, Leksell Gamma Knife® 4C and Figure 65-2 shows the overall appearance of the gamma knife model, Leksell Gamma Knife® 4C.
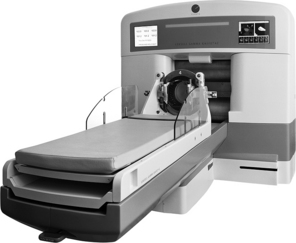
Figure 65-2 Leksell Gamma Knife 4C.
(Published with permission, copyright © 2008, Elekta Instrument AB [Stockholm, Sweden].)
Frame Attachment
The method of anesthesia used during frame placement is surgeon and patient dependent. In our program, either sedation with versed and fentanyl, or monitored anesthesia with propofol, followed by injection of local anesthetic at the pin sites is used. Figure 65-3A shows the typical array of tools used for the frame attachment. A variety of screw lengths allow the surgeon to choose those ideally suited for the individual location of the posts and tumor. The placement of the frame should begin with an accurate orientation of the location of the target within the patient’s head. Ideally, the target should be located within the fiducial range and placed centrally within the frame thereby avoiding later collisions with the collimator helmet and granting sufficient accuracy for the stereotactic target definition.
The stereotactic frame is assembled and preliminarily supported by using external auditory canal support pins, a Velcro band, or a stereotactic fiducial box. When using a fiducial box to facilitate frame placement, it is important to use the MRI fiducial box, rather than the CT or angiography fiducial box, since this is the smallest of the three plexiglass fiducial boxes (Figs. 65-3B and 65-3C). Asymmetric frame placements are possible and do not impair the accuracy of imaging. The frame can be shifted from side to side or can be moved as far as possible to the front or back to facilitate centering of the tumor. The frame is stabilized against the patient by an assistant and the surgeon should adjust the lengths of the posts to maintain relative tumor position. A low position of the anterior posts can help avoid anterior collisions with the collimator helmet for skull base posterior fossa tumors. In critical positions, collisions can sometimes be avoided by using the curved posts in the anterior position.
Measurements of the frame and placement are then performed to allow the computer to identify any potential collisions after the plan is formulated. These measurements are required for the frame and skull section in Leksell GammaPlan treatment planning software. Measurements include the length of the four posts and the length of the screws that protrude from the posts. Additionally, the volume of the head is measured using the plastic collimator bubble, simulating the relationship of the frame to the treatment collimator helmet (Fig. 65-3D). This concludes frame placement and the patient may proceed to imaging.
Imaging
Many centers acquire only MRI scans for treatment planning. We prefer to also acquire a non-contrast CT scan through the temporal bone to aid in planning. There is evidence of distortion of MR images and correlation with CT scans at the time of planning can aid in reducing radiation delivery to critical structures such as the cochlea and facial nerve.10 A CT fiducial box is affixed to the frame, the patient secured in the holder attached to the table, and an axial scan through the temporal bone and skull base acquired. Both CT and MR images are imported into the Gamma Knife workstation. Axial scans are defined, and coronal and sagittal reconstructions generated for each.
Treatment Planning
Dose planning using Leksell GammaPlan involves composing shots to develop a conformal isodose. By definition, this includes the whole target but spares the surrounding healthy tissue. Figure 65-4 shows an example of a vestibular schwannoma. The target is well positioned on the screen and magnified for good visibility. When the shot menu is opened, one can select the size of the collimators. The size of the collimator is selected based on the tumor shape and the gaps in coverage of the 50% isodose line displayed over the tumor. Shots are placed sequentially to cover the target as effectively as possible. Changing the position of the shots, adding additional shots, and adjusting the relative weight of shots quickly leads to a conformal dose plan.
The dose plan can be checked using Leksell GammaPlan with the three-dimensional (3D) image or the measurement tools, such as dose volume histograms. While the subject of conformity index is beyond the scope of this chapter, an excellent review of available methods has been published.11 Leksell GammaPlan indicates the point in the stereotactic space where a global maximal dose can be found. Leksell GammaPlan also calculates the individual shot times. Once the treatment plan has been determined to be appropriate by the gamma knife team (surgeon, radiation oncologist, and radiation physicist), the stereotactic coordinates and irradiation times are printed and used during the gamma knife treatment.
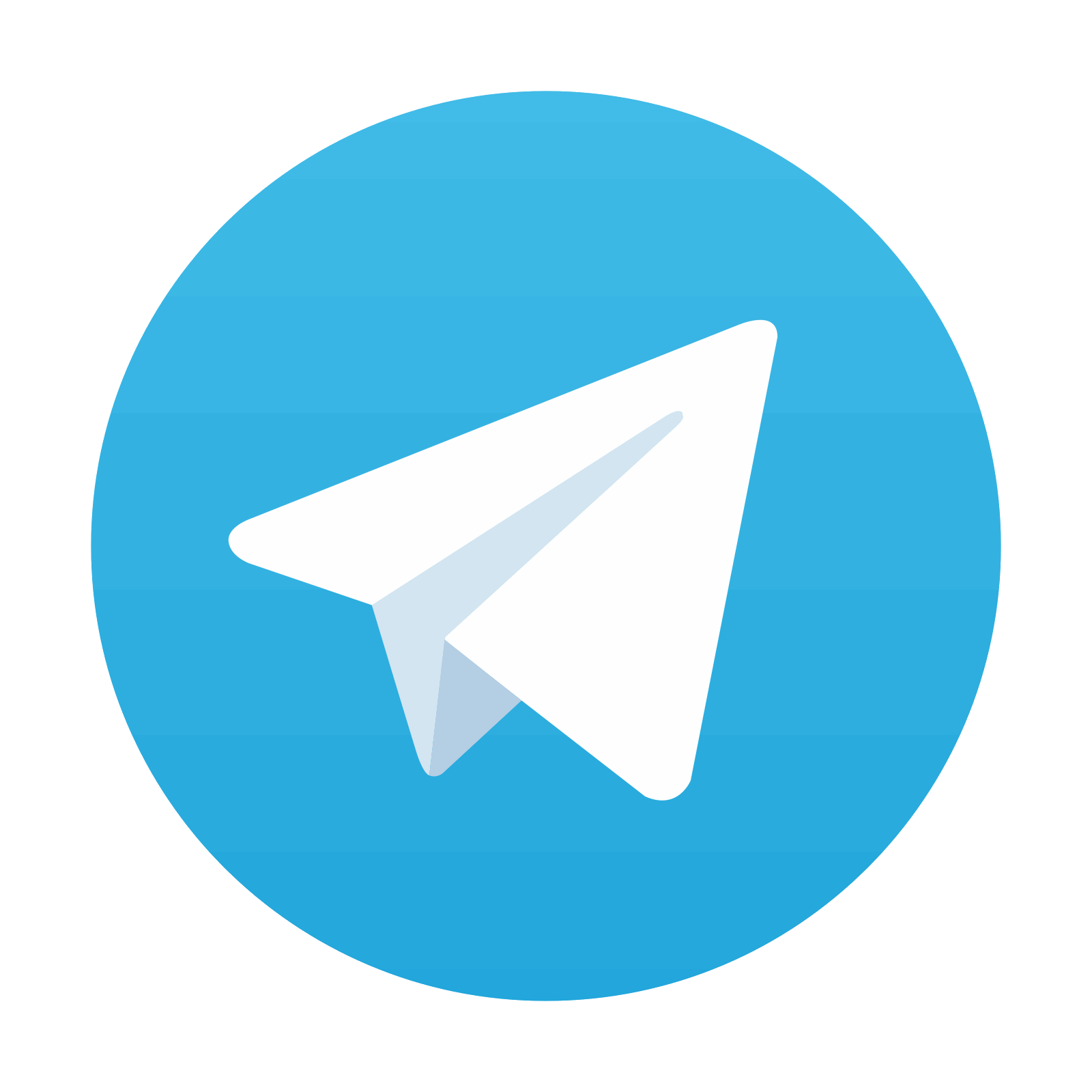
Stay updated, free articles. Join our Telegram channel

Full access? Get Clinical Tree
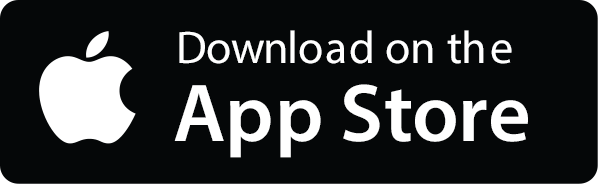
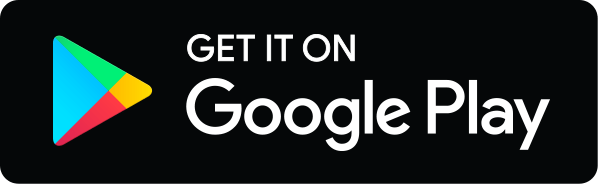