Pathologic Principles of Ophthalmic Disease
J. Douglas Cameron
An understanding of the underlying basic principles of fundamental disease processes can be extremely helpful to the ophthalmologist in dealing with novel clinical problems.
Pathologic processes are those processes that deviate from the normal function of cells and tissues, all of which (both normal and abnormal) are controlled at the molecular level by protein messengers (cytokines) or electrical signals. The clinical relevance of deviations in basic control systems is best appreciated by gathering relevant information at the tissue and cellular levels.
At the tissue level, change can be observed clinically either by gross inspection or by using optical illuminating and magnifying tools (e.g., biomicroscopy, and indirect microscope). The cellular level can be appreciated from tissue sampling and processing by utilizing high-magnification instruments, such as light and electron microscopy. Thus, tissue abnormalities can be identified with clinical methods and understood at the cellular level with the ultimate goal of altering pathological processes surgically at the tissue level or pharmacologically at the molecular level.
Embryology: Developing the Ocular System
After conception, the embryo implants in the uterine wall and creates a cellular plate between the yolk sac and the amniotic sac. The cells of the embryonic plate begin specialized function by dividing into three distinct cell lines: the ectoderm, the mesoderm, and the endoderm. The ectoderm and the mesoderm form the entire eye and supportive tissue. The ectoderm further divides into surface ectoderm, neuroectoderm, and neural crest. The neuroectoderm forms all of the light-sensitive structures of the eye; the neural crest forms the majority of the supportive structures of the eye and orbit. The surface ectoderm forms the primary, light-focusing elements of the eye, the anterior cornea, and the crystalline lens. The posterior corneal stroma, the anterior iris, and the filtration apparatus of the anterior chamber angle are formed by neural crest tissue (Table 1).
TABLE 1-1. Germ Layers, Structures Formed, and Abnormalities of Differentiation | |||||||||||||||||||||||||||||
---|---|---|---|---|---|---|---|---|---|---|---|---|---|---|---|---|---|---|---|---|---|---|---|---|---|---|---|---|---|
|
Migration and differentiation of cells are two of the critical processes bringing about the development of the eye. Abnormalities of migration and differentiation can lead to specific clinical entities.
Failure of cells to differentiate at critical times during development (e.g., such as at uterine implantation) may cause loss of the entire embryo (spontaneous abortion). Failure of differentiation of cells of the primary eye fields in the neuroectoderm may result in primary anophthalmia. Differentiation of neural crest-derived cells proceeds normally in anophthalmia; thus, orbital structures, such as the rectus muscles and orbital bones, are represented.
Dendritic melanocytes normally originate in the neural crest and migrate to their final functional site in the basal layer of the surface ectoderm. If migration is impeded in the deep orbital tissues, abnormal melanocytes form a congenital “blue nevus.” If migrating melanocytes fall just short of the surface epithelium, usually in the skin adnexal units, a junctional nevus is formed (Fig. 1). Similarly, a limbal dermoid results from abnormal proliferation and differentiation of neural crest-derived cells in an abnormal location. Although the cells and their cell products are normal, the tissue has no functional role in the area.
Peters syndrome is a condition resulting from faulty separation of the lens vesicle (an ectodermally derived tissue) from the posterior cornea (a neural crest-derived tissue). Clinically, the hallmark is central, posterior corneal opacity; histologically, it is a defect of variable extent in Descemet’s membrane and posterior corneal stroma (see Table 1).
Defects of cell cycle control mechanism located at 13q14 may lead to uncontrolled proliferation of cells or cancer. If both alleles of the gene at this location (the Rb gene) are defective or absent primitive retinal cells may proliferate to form retinoblastoma, a potentially fatal tumor.
Trauma: Threats to the Ocular System
Trauma usually involves disruption of cells or disruption of cell structural support, which translates into disruption of tissue and loss of function. Entire organ systems may be lost because of extensive degrees of injury. Disruption of cell membranes may be either physical or biochemical.
The cell membranes of all cells are composed of phospholipids. The phospholipid membrane is the interface at which the cells responds to and influences its environment. Phospholipids are soluble at alkaline pH.
Alkali injuries are particularly destructive, because alkali dissolves all cell membranes with which it comes in contact. After the surface cells have been destroyed, alkali is free to diffuse deeper into the tissue where it can lyse both surface and vascular endothelial cells (Fig. 2). Involvement of vascular endothelial cells compounds the injury by adding downstream ischemia. Diffusion continues until the pH is neutralized.
Acid injuries are usually more limited, because acid causes denaturation and precipitation of cellular proteins. A diffusion barrier is, thus, created to limit damage to deeper tissues (Fig. 2).
Mechanical trauma may be focused, which occurs from a concentrated application of pressure (e.g., a steel knife cutting through tissue) or unfocused, which occurs from blunt trauma. Cell death is present on both sides of even the sharpest knife wound because of the transection of cell processes. The death of corneal keratocytes, which have extensively arborizing cellular processes, may extend several microns on either side of a corneal incision. Corneal opacification occurs in the area normally maintained by damaged or dead keratocytes.
Blunt trauma may cause simultaneous injury to cells in a large area, which occurs with a corneal abrasion; it may also deform large segments of tissue. The vulnerability of specific ocular tissues varies greatly (Table 2). Elastic tissue, such as Descemet’s membrane, the crystalline lens capsule, the zonular fibers of the lens, and Bruch’s membrane have a limited range of deformability; beyond which they will rupture. Tightly adherent structures, such as the iris root and the longitudinal ciliary muscle inserted into the scleral spur, concentrate pressure and may also rupture. Transparent structures, such as the cornea, crystalline lens, and retina, must maintain homogeneity to maintain transparency. Trauma frequently results in loss of homogeneity sufficient to cause opacification. When hydrostatic pressure is increased suddenly, pressure is transmitted equally throughout the globe, seeking sites of relative weakness. These sites include the sclera at the limbus, which is relatively thin to accommodate the structures of the trabecular meshwork; the point of insertion of the rectus muscles, and the optic disc (see Table 2).
TABLE 1-2. Ocular Tissue Vulnerable to Mechanical Trauma | |||||||||||||||||||||||||||||||||||
---|---|---|---|---|---|---|---|---|---|---|---|---|---|---|---|---|---|---|---|---|---|---|---|---|---|---|---|---|---|---|---|---|---|---|---|
|
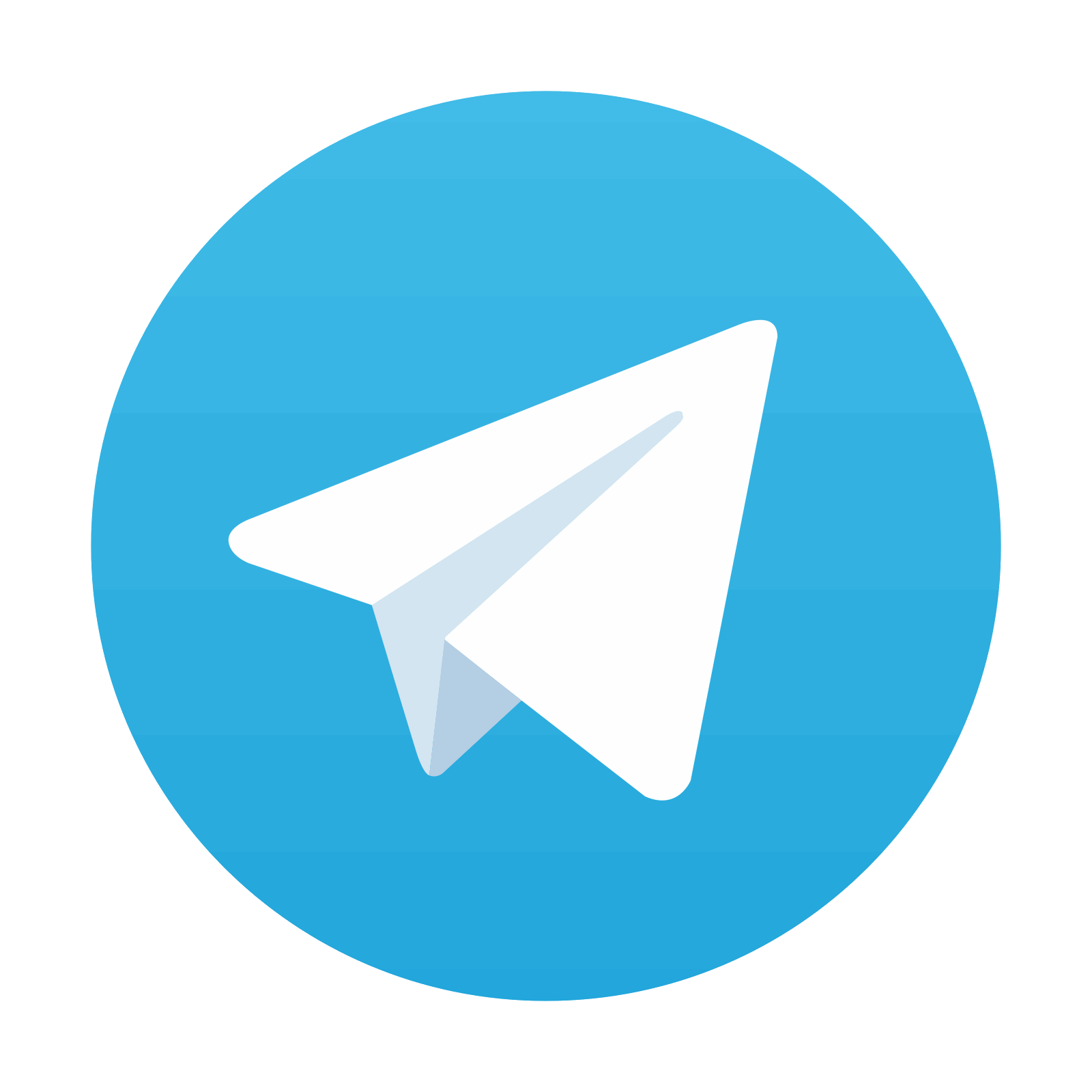
Stay updated, free articles. Join our Telegram channel

Full access? Get Clinical Tree
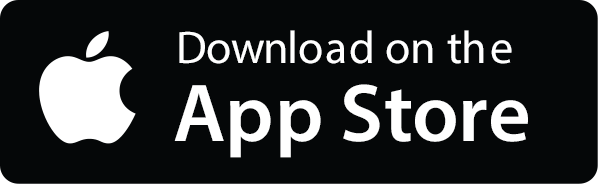
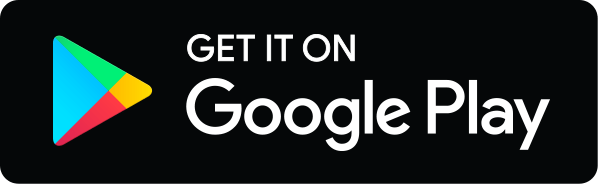