Figure 6-1 An example of meridional (tangential, left) and axial (right) maps of a normal cornea. (Used with permission from Roberts C. Corneal topography. In: Azar DT, ed. Gatinel D, Hoang-Xuan T, associate eds. Refractive Surgery. 2nd ed. St Louis: Elsevier-Mosby; 2007:103–116.)
Following keratorefractive surgery for the treatment of myopia, the cornea becomes less prolate and has a shape resembling that of an egg lying on its side. The central cornea becomes flatter than the periphery. This flattening results in a change in the spherical aberration of the treated zone.
To demonstrate this change, consider the point spread function produced by all rays that traverse the pupil from a single object point. Generally, keratorefractive surgery for myopia reduces spherical refractive error and regular astigmatism, but it does so at the expense of increasing spherical aberration and irregular astigmatism (Fig 6-2). Keratorefractive surgery moves the location of the best focus closer to the retina but, at the same time, makes the focus less stigmatic. Such irregular astigmatism is what underlies many visual complaints after refractive surgery.
Figure 6-2 Examples of the effects of (A) coma, (B) spherical aberration, and (C) trefoil on the point spread functions of a light source and a Snellen letter E. (Courtesy of Ming Wang, MD.)
A basic premise of refractive surgery is that the cornea’s optical properties are intimately related to its shape. Consequently, manipulation of the corneal shape changes the eye’s refractive status. Although this assumption is true, the relationship between corneal shape and the cornea’s optical properties is more complex than is generally appreciated.
Ablative procedures, incisional procedures, and intracorneal rings change the natural shape of the cornea to reduce refractive error. Keratometry readings in eyes conducted before they undergo keratorefractive surgery typically range from 38.0 D to 48.0 D. When refractive surgical procedures are being considered, it is important to avoid changes that may result in excessively flat (<33.0 D) or excessively steep (>50.0 D) corneal powers. A 0.8 D change in keratometry value (K) corresponds to approximately a 1.00 D change of refraction. The following equation is often used to predict corneal curvature after keratorefractive surgery:
Kpostop = Kpreop + (0.8 × RE)
where Kpreop and Kpostop are preoperative and postoperative K readings, respectively, and RE is the refractive error to be corrected at the corneal plane. For example, if a patient’s preoperative keratometry readings are 45.0 D (steepest meridian) and 43.0 D (flattest meridian), then the average K value is 44.0 D. If the amount of refractive correction at the corneal plane is –8.50 D, then the predicted average postoperative K reading is 44.0 + (0.8 × –8.50 ) = 37.2 D, which is acceptable.
The ratio of dioptric change in refractive error to dioptric change in keratometry approximates 0.8:1 owing to the change in posterior corneal surface power after excimer ablation. The anterior corneal surface produces most of the eye’s refractive power. In the Gullstrand model eye (see Table 2-1), the anterior corneal surface has a power of +48.8 D and the posterior corneal surface has a power of –5.8 D, so the overall corneal refractive power is +43.0 D. Importantly, standard corneal topography instruments and keratometers do not measure corneal power precisely because they do not assess the posterior corneal surface. Instead, these instruments estimate total corneal power by assuming a constant relationship between the anterior and posterior corneal surfaces. This constancy is disrupted by keratorefractive surgery. For example, after myopic excimer surgery, the anterior corneal curvature is flattened. At the same time, the posterior corneal surface remains unchanged or, owing to the reduction in corneal pachymetry and weakening of the cornea, the posterior corneal surface may become slightly steeper than the preoperative posterior corneal curvature, increasing its negative power. The decrease in positive anterior corneal power and the (minimal) increase in negative posterior corneal power cause an increase in the relative contribution to the overall corneal refractive power of the posterior surface.
The removal of even a small amount of tissue (eg, a few micrometers) during keratorefractive surgery may cause a substantial change in refraction (Fig 6-3). The Munnerlyn formula approximates these 2 parameters:
where t is the depth of the central ablation in micrometers, S is the diameter of the optical zone in millimeters, and D is the degree of refractive correction in diopters.
Figure 6-3 Comparison of a 43 D cornea with a 45 D cornea. Numbers below the vertical arrows indicate distance from the optical axis in millimeters; numbers to the right of the horizontal arrows indicate the separation between the corneas in micrometers. A typical pupil size of 3.0 mm is indicated. A typical red blood cell has a diameter of 7 µm. Within the pupillary space (ie, the optical zone of the cornea), the separation between the corneas is less than the diameter of a red blood cell. (Courtesy of Edmond H. Thall, MD. Modified by C. H. Wooley.)
An ideal LASIK ablation or PRK removes a convex positive meniscus in corrections of myopia (Fig 6-4A) and a concave positive meniscus in simple corrections of hyperopia (Fig 6-4B). A toric positive meniscus is removed in corrections of astigmatism. In toric corrections, the specific shape of the ablation depends on the spherical component of the refractive error.
Figure 6-4 A, Schematic illustration of myopic photorefractive keratectomy. The shaded area refers to the location of tissue subtraction. More stromal tissue is removed in the central than in the paracentral region. B, Schematic illustration of hyperopic laser in situ keratomileusis. A superficial corneal flap is raised. The shaded area refers to the location of tissue subtraction under the thin flap. After treatment, the flap is repositioned. (Used with permission from Poothullil AM, Azar DT. Terminology, classification, and history of refractive surgery. In: Azar DT, ed. Gatinel D, Hoang-Xuan T, associate eds. Refractive Surgery. 2nd ed. St Louis: Elsevier-Mosby; 2007:5–6. Figs 1-4, 1-5.)
Azar DT, ed. Gatinel D, Hoang-Xuan T, associate eds. Refractive Surgery. 2nd ed. St Louis: Elsevier-Mosby; 2007.
Azar DT, Primack JD. Theoretical analysis of ablation depths and profiles in laser in situ keratomileusis for compound hyperopic and mixed astigmatism. J Cataract Refract Surg. 2000;26(8):1123–1136.
Klyce S. Night vision after LASIK: the pupil proclaims innocence. Ophthalmology. 2004;111(1):1–2.
Munnerlyn CR, Koons SJ, Marshall J. Photorefractive keratectomy: a technique for laser refractive surgery. J Cataract Refract Surg. 1988;14(1):46–52.
Angle Kappa
As discussed in Chapter 2, the pupillary axis is the imaginary line that is perpendicular to the corneal surface and passes through the midpoint of the entrance pupil. The visual axis is the imaginary line that connects the point of fixation to the fovea. The angle kappa (κ) is defined as the angle between the pupillary axis and the visual axis. A large angle kappa results from a significant difference between the pupillary axis and the central corneal apex. If the angle kappa is large, centering an excimer ablation over the geometric center of the cornea will effectively result in a decentered ablation. This can be particularly problematic in a hyperopic correction, in which a large angle kappa can result in a refractively significant “second corneal apex,” causing monocular diplopia and decreased quality of vision. A large angle kappa must be identified before surgery to reduce the likelihood of a poor visual outcome.
Freedman KA, Brown SM, Mathews SM, Young RS. Pupil size and the ablation zone in laser refractive surgery: considerations based on geometric optics. J Cataract Refract Surg. 2003;29(10):1924–1931.
Pupil Size
Pupil size measurement is becoming the standard of care in preoperative evaluations, prompted by observations that some patients with large pupils (>8 mm) reported difficulties with night vision after undergoing keratorefractive surgery. Typical symptoms included the appearance of glare, starbursts, and halos; decreased contrast sensitivity; and poor overall quality of vision. Night-vision problems tended to occur in patients with both large pupils and small treatment zones (≤6 mm). The algorithms used in third-generation lasers, however, incorporate larger optical and transition zones, enabling surgeons to perform refractive procedures on patients with larger pupils. Use of these algorithms has dramatically decreased the incidence and severity of night-vision problems.
Many surgeons use default ablation zones during excimer procedures. The accepted standard transition zone between ablated and unablated cornea is 0.5–1.0 mm larger than the pupil; use of this zone helps minimize night-vision problems. To conserve corneal tissue, smaller optical zones are typically used in higher myopic corrections. In patients who require such corrections, the incidence of night-vision problems increases in part because of the mismatch between the size of the pupil and that of the optical zone.
Although pupil size does not affect surgical outcome as significantly as it once did, pupil size measurement continues to be the standard of care in preoperative evaluation. Patients with extremely large pupils (≥8 mm) should be identified and counseled about the potential for increased risk of complications. Spherical aberration may be increased in these patients. Clinical management of postoperative night-vision problems includes the use of a miotic such as brimonidine (0.2%) or pilocarpine (0.5%–1%).
Freedman KA, Brown SM, Mathews SM, Young RS. Pupil size and the ablation zone in laser refractive surgery: considerations based on geometric optics. J Cataract Refract Surg. 2003;29(10):1924–1931.
Klyce S. Night vision after LASIK: the pupil proclaims innocence. Ophthalmology. 2004;111(1):1–2.
Lee YC, Hu FR, Wang IJ. Quality of vision after laser in situ keratomileusis: influence of dioptric correction and pupil size on visual function. J Cataract Refract Surg. 2003;29(4):769–777.
Schallhorn SC, Kaupp SE, Tanzer DJ, Tidwell J, Laurent J, Bourque LB. Pupil size and quality of vision after LASIK. Ophthalmology. 2003;110(8):1606–1614.
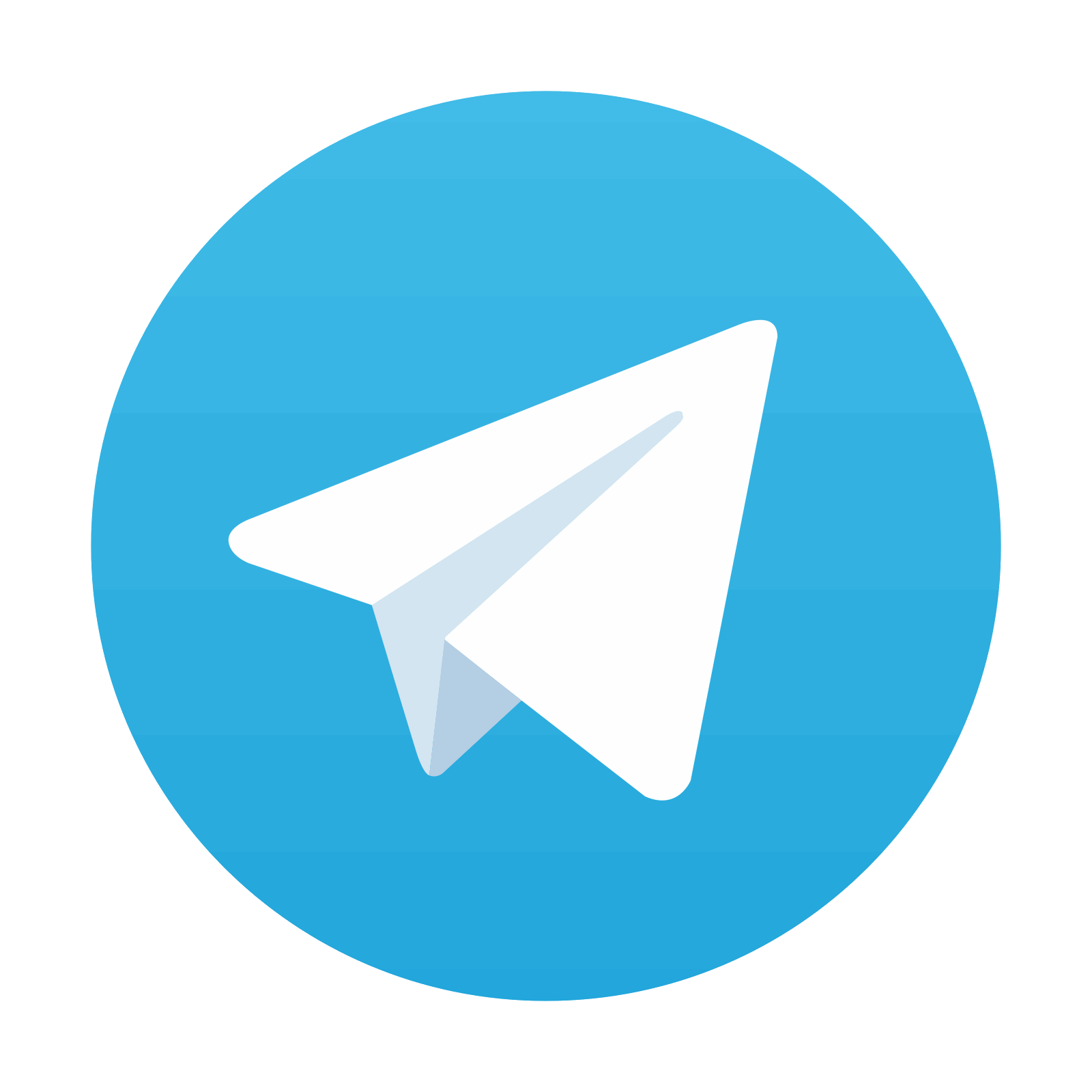
Stay updated, free articles. Join our Telegram channel

Full access? Get Clinical Tree
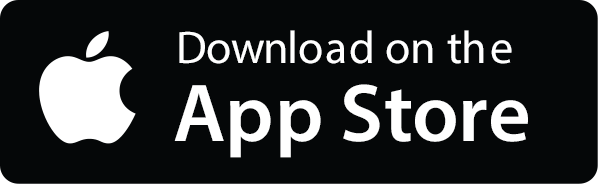
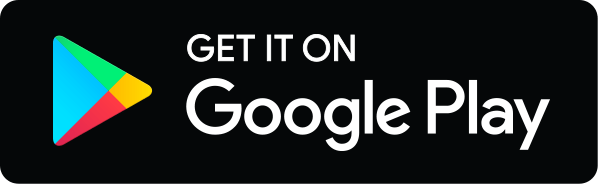