CHAPTER 145 Management of Temporal Bone Trauma
Epidemiology
Motor vehicle accidents commonly result in head trauma of varying degrees of severity. In the past, 75% of motor vehicle accidents resulted in a head trauma; however, the increased use of seatbelts and the advent of airbags may alter these statistics in the future. When the head trauma is of sufficient magnitude to fracture the skull, 14% to 22% of injured patients sustain a temporal bone fracture.1,2 In the largest series of temporal bone fractures reported to date, 31% of the fractures resulted from motor vehicle accidents (Fig. 145-1).3
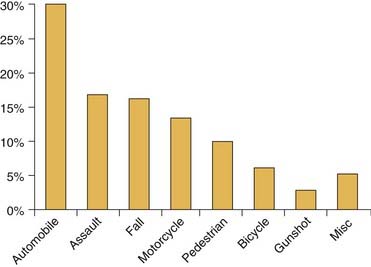
Figure 145-1. Type of injury.
(Data from Brodie HA, Thompson TC, Management of complications from 820 temporal bone fractures. Am J Otol. 1997;18:188.)
Temporal bone fractures are reported to occur across all age groups, with more than 70% of fractures occurring during the second, third, and fourth decades of life.3 These fractures occur predominantly in men in a ratio of 3 : 1,3 which is attributed to involvement in riskier activities rather than to structural weaknesses in the male skull.
Pathophysiology
The temporal bones are pyramidal structures in the thick bone of the skull base and consequently require a great force to fracture. The force of lateral impact required to fracture the temporal bones of fresh cadavers is estimated at 1875 pounds.4 The fractures take the path of least resistance, which is along the structurally weakened points, such as the various foramina that perforate the skull base.
The temporal bone houses or encapsulates many important structures, including the facial nerve, cranial nerves IX through XI, the cochlea, the labyrinth, the ossicles, the tympanic membrane, the carotid artery, and the jugular vein. Any of these structures can be injured with a fracture of the bone. Fracture of the temporal bone can violate dura, resulting in cerebrospinal fluid (CSF) fistula, meningitis, and brain herniation. In addition to neurotologic manifestations of involved structures within the temporal bone, associated intracranial injuries, such as epidural or subdural hematomas, cerebral edema, post-traumatic encephalopathy, and elevated intracranial pressure, may occur. Neurotologic symptoms can also result from shearing strain within the brain tissue with disruption of vessels, axons, synapses, and dendrites.5
Sixty percent of temporal bone fractures are categorized as open fractures, presenting with bloody otorrhea, brain herniation, or CSF draining from the ear canal, eustachian tube, or penetrating wound site.3 Eight percent to 29% of temporal bone fractures occur bilaterally.3,6,7
Classification
Temporal bone fractures have traditionally been divided into transverse and longitudinal categories on the basis of the relationship of the fracture line to the axis of the petrous ridge.8 This classification has given way to a new scheme that classifies fractures by whether they disrupt or spare the otic capsule (the bone that houses the cochlea and the semicircular canals) (Figs. 145-2 through 145-4).3,9
Otic capsule–disrupting fractures pass through the otic capsule, generally proceeding from the foramen magnum across the petrous pyramid and the otic capsule. The fracture often passes through the jugular foramen, the internal auditory canal, and the foramen lacerum; these fractures do not typically affect the ossicular chain or the external auditory canal.10 Otic capsule–disrupting fractures generally result from blows to the occipital region.
Longitudinal fractures reportedly make up 70% to 90% of temporal bone fractures, with the remaining 10% to 30% categorized as transverse.8–14 In two large series using the newer classification scheme, only 2.5% to 5.8% of fractures disrupted the otic capsule,3,15 suggesting that many fractures that are oriented perpendicular to the petrous ridge do not actually cross the otic capsule. Many of the otic capsule–disrupting fractures are actually oriented in the longitudinal plane.15
The rationale for changing the classification scheme is to focus on the functional sequelae and complications of temporal bone fractures as opposed to merely describing the anatomic orientation of the fracture. Fractures that disrupt the otic capsule almost always result in a sensorineural hearing loss, although there are reported exceptions.16 Otic capsule–sparing fractures tend to have conductive or mixed hearing loss,3 whereas otic capsule–disrupting fractures have a much higher incidence of facial nerve paralysis (30% to 50% vs. 6% to 14%).3,15 In addition, Fisch17 reported a much higher incidence of nerve disruption in fractures involving the otic capsule. There is a twofold to fourfold increase in CSF fistula in otic capsule–disrupting fractures as well as a much greater risk of intracranial injuries.3,15 These fractures may also carry a higher risk of delayed meningitis due to an inability of the otic capsule enchondral bone to remodel and heal.18,19 Pollak and colleagues19 reported the case of a 51-year-old man who died of meningitis after having suffered an otic capsule–disrupting fracture during childhood. The histopathology of his temporal bone revealed pus in the middle ear extending through an unhealed fracture line across the otic capsule, and the fracture line contained a loose fibrous tissue. The membranous bone along the tegmen has the capability to heal, whereas the enchondral bone of the otic capsule does not. Fractures through the otic capsule generally partially fill with fibrous tissue and potentially seal with periosteal bone reaction on the surface of the otic capsule (Fig. 145-5).19
Temporal bone fractures in the pediatric population differ somewhat from those in adults. There is a higher incidence of intracranial complications (58%) and a lower incidence of facial nerve paralysis (3%) in children.20
Evaluation
The ear canal is inspected for fractures along the roof, CSF otorrhea, the degree of hemorrhage, and the presence of brain herniation. The ear is examined as aseptically as possible. Blood and cerumen in the ear canal should never be débrided with irrigation. After stabilization and transfer, the ear can be carefully examined with the aid of an operating microscope. Typical findings include fractures along the scutum and roof of the external auditory canal and tympanic membrane perforations. Hemotympanum or bloody otorrhea is almost invariably present (Figs. 145-6 to 145-8).
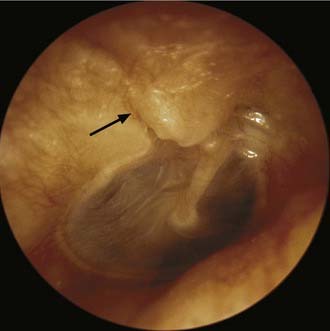
Figure 145-7. Otoscopic image that demonstrates a displaced fracture along the scutum (black arrow).
The neurotologic examination should also note the presence or absence of nystagmus and the type of nystagmus. Peripheral vertigo generally manifests with horizontal or rotatory nystagmus and is suppressible with visual fixation. Central vertigo may present with vertical or direction-changing nystagmus that fails to suppress and may even enhance with fixation. The most common type of vertigo after head trauma is benign paroxysmal position vertigo, which manifests with transient rotatory nystagmus with the Halpike maneuver.21 The nystagmus occurs with the affected ear down. There is a 2- to 10-second latency followed by 10 to 20 seconds of rotatory nystagmus; the nystagmus is fixed in direction and is fatigable. Central vertigo induces direction-changing nystagmus, which has no latency and is not fatigable. Interestingly, the incidence of vertigo does not closely correlate with the severity of the trauma.22
Patients with severe head trauma of the magnitude required for a temporal bone fracture generally already have had a computed tomography (CT) scan of the head to assess for intracranial hemorrhage. Additional imaging of the temporal bone with axial and coronal high-resolution CT scanning (HRCT) is indicated in the presence of facial paralysis, CSF fistula, disruption of the superior wall of the external auditory canal or scutum with potential trapping of epithelium (Fig. 145-9), or suspected vascular injury. HRCT of the temporal bone is also indicated if surgical intervention is required for the management of an otologic complication. Hearing loss (conductive or sensorineural) in the absence of other complications does not warrant additional temporal bone imaging. Demonstrating a transverse fracture through the otic capsule in a profound sensorineural hearing loss does not alter the treatment plan. Preoperative assessment with CT scanning in patients with a conductive hearing loss of sufficient magnitude to warrant exploration and ossicular reconstruction may provide useful information that may influence the surgical approach.
The role of HRCT in the evaluation of potential carotid artery injuries is unclear. Resnick and colleagues23 reported a 5% incidence of carotid injury in basilar skull fractures if the fracture spares the carotid canal and an 18% incidence of carotid injury in patients with fractures through the carotid canal seen on HRCT. However, Kahn and colleagues24 argues that, in an asymptomatic patient, HRCT demonstration of a fracture through the carotid canal yields no valuable additional information. Subsequent angiography performed in cases of asymptomatic carotid canal fractures yielded no evidence of carotid artery injury and provided no clinical utility.24 Consequently, in completely asymptomatic patients who have sustained temporal bone fractures and who are neurologically intact, HRCT and angiography are not required. On the other hand, if there are any transient or persistent neurologic deficits in patients with basilar skull fractures, HRCT of the temporal bone and CT angiography are indicated (Fig. 145-10).
Management of Complications
Facial Nerve Injury
Facial paralysis is a severely disfiguring complication of temporal bone fractures. Seven percent of temporal bone fractures result in facial paralysis; 25% of such cases involve complete paralysis.3 The reported incidence in the literature of up to 30% is exaggerated by sampling error due to omitting simple uncomplicated temporal bone fractures that are not referred for otolaryngologic consult. The incidence of facial paralysis is also overestimated by including patients in retrospective reviews who have been referred to the tertiary care center for the management of complications of temporal bone fractures (e.g., facial paresis). Because the entire pool of temporal bone fracture patients is not included in the statistics, the incidence of complications becomes quite biased.
If head trauma patients are carefully evaluated in the emergency department upon admission (before the administration of muscle relaxants), 27% of the facial nerve injuries will present with immediate-onset facial paralysis. Seventy-three percent will have facial motion in the initial examination and subsequently deteriorate.3 The latency of delay in onset of facial palsy ranges from 1 to 16 days. It is crucial to differentiate “delayed onset” from “delayed diagnosis.” Delayed onset of facial paralysis is defined as documented facial function in the emergency department that subsequently deteriorates; delayed diagnosis of facial paralysis occurs when the patient is given a paralytic agent and is intubated before the examination of facial function. In this situation, an assessment of facial function is delayed until extubation. These patients should be categorized as unestablished onset and treated in a manner that is similar to the immediate onset patients. In one large series, 10% of patients fell into this unestablished onset category.25
The delay of onset of paralysis after temporal bone fracture is the most important of the predictive factors. In one series of 37 delayed-onset facial palsies, five were lost to follow-up, and the remainder recovered to a House-Brackmann grade I or II.3 McKennan and Chole26 described their experience with 17 patients with immediate-onset facial paralysis and 19 patients with delayed-onset paralysis. Complete spontaneous recovery of facial function occurred in 94% of the patients with delayed-onset paralysis; the one remaining patient had a House-Brackmann grade II recovery. By contrast, 8 of the 17 immediate-onset paralysis patients had facial nerve transsections.
Turner7 reviewed a large series of traumatic facial paralysis patients who were treated conservatively. His paper included 36 immediate-onset and 34 delayed-onset facial palsies. Complete recovery occurred in 94% of the delayed-onset cases and 75% of immediate-onset cases. The one patient with delayed-onset paralysis who had no recovery of function developed the facial palsy coincident with acute otitis media. By contrast, Maiman and colleagues27 found no correlation between immediate- or delayed-onset facial paralysis (treated nonsurgically) and outcome. However, 44 of 45 of their patients (including both immediate- and delayed-onset patients) had satisfactory recovery. Review of the literature argues strongly against surgical exploration and decompression of delayed post-traumatic and facial paralysis. The natural course of delayed facial paralysis is almost always the recovery of facial function to a House-Brackmann grade I or II. There are no convincing data in the literature that demonstrate that surgical intervention in delayed-onset paralysis will increase the probability of complete recovery of function.
May28 describes the difficulty in differentiating immediate-onset from delayed-onset facial paralysis. He explored reported delayed-onset palsies and found, on occasion, a severed nerve; this observation highlights the necessity of careful examination in the emergency department. Even in comatose patients, painful stimuli generally induce a grimace. Admittedly information about the immediate facial nerve function is not always available. Sometimes the examination is omitted because attention is given to other life-threatening complications or because the patient has already received muscle relaxants with intubation. Some patients are not responsive to pain, and a grimace cannot be induced; the critical issue is whether or not any facial function has been identified. If facial function is present in the emergency department and subsequently deteriorates, our experience is that the patient will recover without surgical treatment. When reliable information from the emergency department is unavailable, the patient is never documented to have facial function, and the diagnosis of facial palsy is delayed by a few days, these cases must be categorized as “unknown onset” and considered as part of the immediate-onset group.
The degree of facial nerve injury is also a critical factor for guiding the management algorithm. Incomplete paresis rarely fails to resolve spontaneously unless an additional insult to the nerve, such as infection, occurs.3 Consequently, only patients with complete paralysis of immediate or unknown onset are considered for surgical exploration.
The degree of the injury can be assessed not only clinically with facial motion but also with electrodiagnostic testing using the Hilger facial nerve stimulator, evoked electromyography (EEMG), and standard electromyography (EMG). The role of electrodiagnostic testing is to assist the clinician in differentiating a neuropraxic injury from a neural degenerative injury and to assess the proportion of degenerated axons. Nerves that have sustained a neuropraxic injury proximal to the stimulated portion of the nerve maintain electrical stimulability. Partial or complete disruption of the nerve results in wallerian degeneration and consequent decrease or loss of stimulability. However, the distal segment of the nerves maintains electrical stimulability for 3 to 5 days29; consequently, electrodiagnostic testing cannot reliably differentiate a neuropraxic injury from a laceration of the nerve for up to 3 to 5 days.
Sunderland30 classified nerve fiber injuries into five categories. The first-degree injury is anatomically intact, with a conduction blockade (neuropraxia); these lesions tend to recover completely. The second-degree injury transects the axons but maintains an intact endoneurium (axonotmesis); these lesions also tend to resolve without subsequent deficits. The third-degree injury transacts the axon and endoneurium but maintains an intact perineurium (neurotmesis); aberrant regeneration can occur with third-degree injuries and leave patients with some weakness and synkinesis. Fourth-degree injuries transect the entire nerve trunk but maintain an intact epineural sheath (neurotmesis). Loss of the conduit of the epineural sheath allows regenerating axons to cross into adjacent fascicles, thereby resulting in a loss of topographic organization. A proportion of the regenerating fibers are also lost as a result of the healing process and scarring. These lesions result in a high incidence of residual weakness, synkinesis, and hyperkinesis. Complete transection of the entire nerve trunk and epineurium is classified as a fifth-degree injury (neurotmesis) and is associated with poor (if any) likelihood of spontaneous recovery, depending on the degree of diastasis of the nerve ends.
May and colleagues31 argue that the MST provides a more reliable estimation of the degree of degeneration. In the MST, stimulation is performed similar to the way it is for NET, but the intensity of the stimulus is increased until the amount of facial contraction plateaus or is limited by patient intolerance. The degree of contraction is subjectively assessed by the physician and compared with electrical stimulation of the unaffected side of the face. The difference in contraction is expressed as equal, mildly decreased, markedly decreased, or no response; the latter two categories are associated with poorer prognosis. Much like NET, MST is most useful between days 3 and 14 after injury in patients with dense facial paralysis.
EEMG has been popularized by Fisch32 in a version called electroneuronography (ENoG). ENoG differs from EEMG only in the use of bipolar stimulating and recording electrodes. Both techniques measure the evoked compound muscle action potential (CAP) and provide similar information to the MST, but in an objective fashion. The stimulating electrode is placed adjacent to be stylomastoid foramen, and the recording electrode is placed in the nasolabial crease. The diminution in the amplitude of the CAP is indicative of the percentage of degenerated nerve fibers. EEMG has been demonstrated to be the most accurate electrodiagnostic test for prognostic information.33 Fisch34 reported that patients in whom the degeneration on EEMG reaches 90% within 6 days of onset of traumatic facial paralysis have a poorer outcome and consequently should undergo facial nerve decompression. Sillman and colleagues35 demonstrated a significant association between a CAP decline of more than 90% and poor recovery of function for idiopathic paralysis, but they demonstrated no significant association between a CAP decline of more than 90% and clinical outcome in traumatic paralysis.
The value of EMG for the acute management of traumatic facial paralysis remains controversial. Standard monopolar EMG is performed with the insertion of an EMG electrode into the muscle, and the spontaneous electrical activity is then recorded. Two types of information are obtainable: voluntary activity and fibrillation potentials. If voluntary activity is present during the acute postinjury period, the patient has a very high probability of good recovery.35
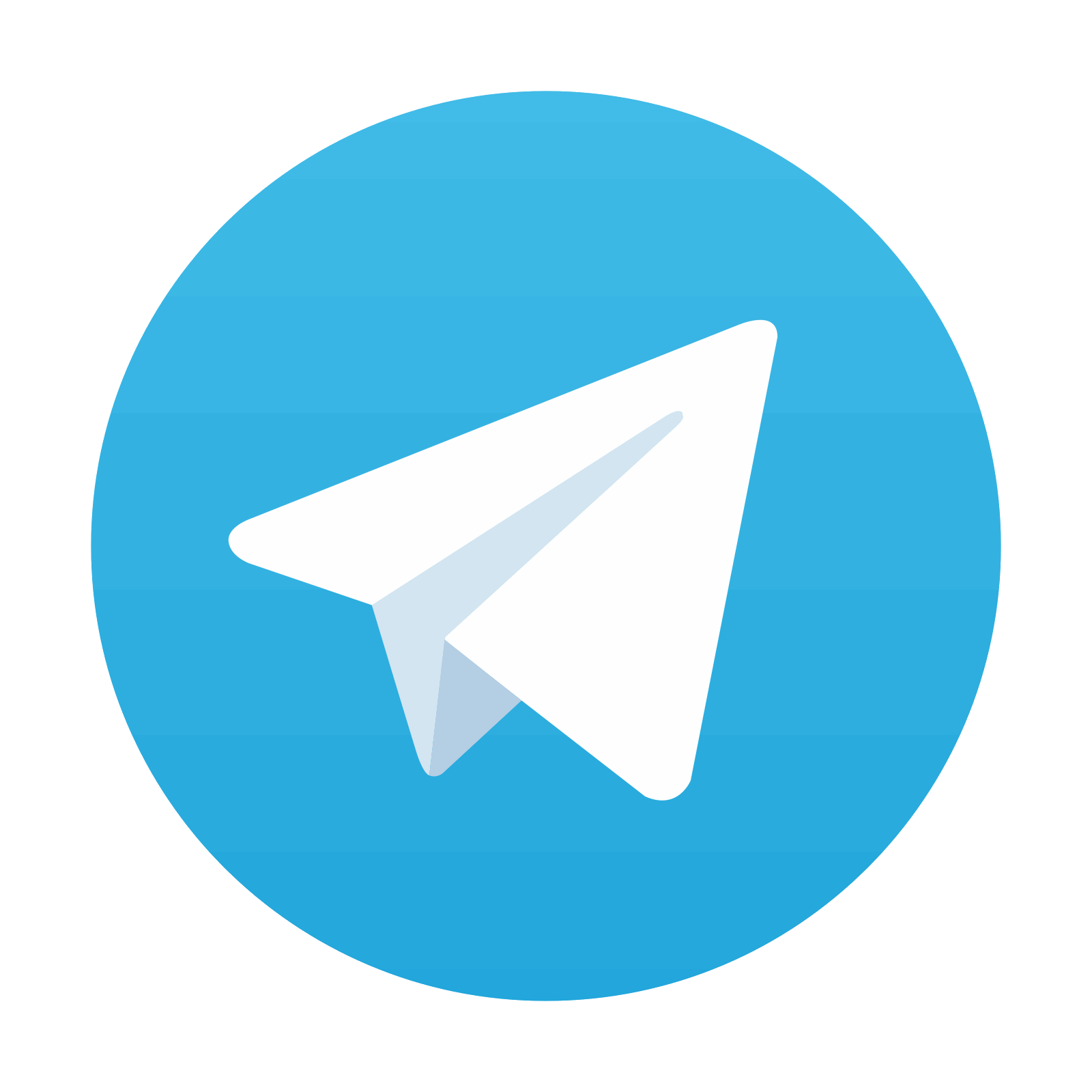
Stay updated, free articles. Join our Telegram channel

Full access? Get Clinical Tree
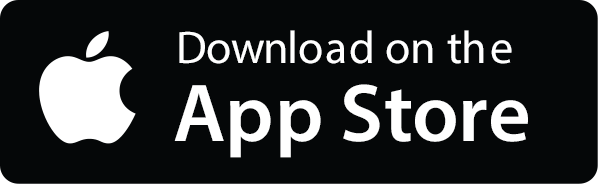
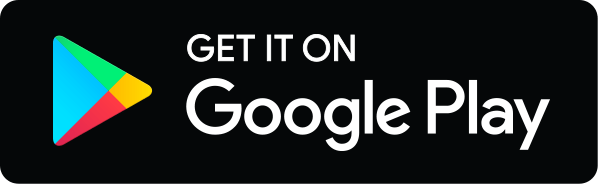