Fig. 4.1
Uptake of radiolabeled (a) l-Glu, (b) d-Asp, and (c) l-Cys by Na+-dependent and Na+-independent transport systems in HCEC. Each point represents the mean CPM/culture and SEM of three experiments
Cellular distribution of immunoreactive EAATs and xCT (light chain of Glu/Cys exchanger) [32] in HCEC. All Na+-dependent EAATs and xCT were detected in HCEC cultures (Fig. 4.2). The relative amount and location of the EAAT1-5 varied from undetectable to highly concentrated in HCEC within each monolayer. Notably, EAAT1 was expressed weakly in some cells, but was highly concentrated in the cytoplasm on one side of the nucleus in many positive HCEC (Fig. 4.2a). EAAT2 was absent in some cells, but was detected in the cytoplasm and nuclei of other HCEC (Fig. 4.2b). EAAT3 signal was detected in the plasma membrane, was highly concentrated in the cytoplasm of many cells, and was absent or very weakly expressed in some cells (Fig. 4.2c). EAAT4 was detected weakly in all HCEC, but was concentrated in the cytoplasm of a few cells (Fig. 4.2d). A weak EAAT5 signal was detected in the perinuclear cytoplasm of most cells (Fig. 4.2e). The immunoreactive signal for the light chain xCT of the Xc− antiporter was detected on the membranes and cytoplasm of most cells (Fig. 4.2f). Thus, these results support the results of the Na-dependent uptake studies and suggest the relative levels of expression in HCEC cultures was EAAT3 > EAAT2 = xCT > EAAT1 > EAAT4 > EAAT5.
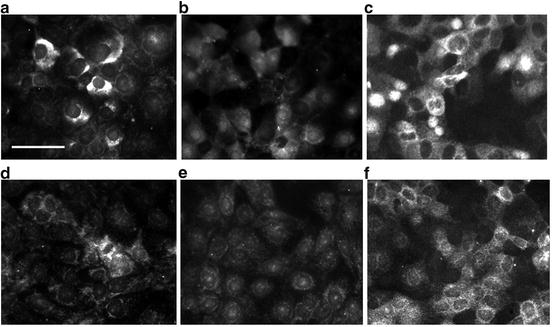
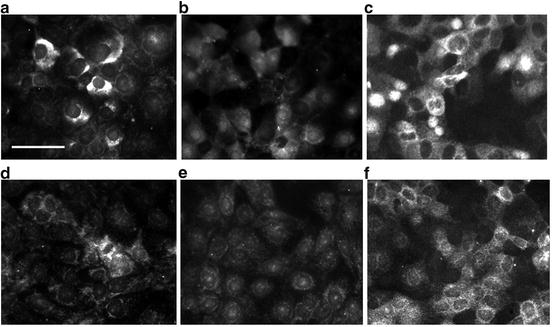
Fig. 4.2
EAATs and Xc− antiporter (xCT) in HCEC cultures. Cellular localization of immunoreactive (a) EAAT1, (b) EAAT2, (c) EAAT3, (d) EAAT4, (e) EAAT5, and (f) xCT peptides in HCEC. Note the predominance of cytoplasmic EAAT1 and EAAT3 labeling, the weaker EAAT2, EAAT4, and EAAT5 labeling, and the surface labeling of xCT in HCEC. Bar = 50 μm
Concentration–dependent substrate inhibition of EAAT. To characterize the Na+-dependent EAAT activity in HCEC, [3H]-d-Asp uptake in the presence of different EAAT competitive inhibitor and blocker concentrations was determined. l-Glu, d-Asp, THA, and TBOA inhibited the uptake of [3H]-d-Asp in a concentration-dependent manner (Fig. 4.3). The IC50 of the EAAT blocker TBOA (3.5 μM) was 2 to 8-fold lower than the IC50s of the competitive EAAT inhibitors l-Glu, d-Asp, and THA (~16 μM, 10 μM, and 20 μM, respectively). In contrast, the IC50 of KA was >100 μM. The weak inhibition of KA and d-Glu suggests that the uptake of [3H]-d-Asp in HCEC was not due to EAAT2. The strong inhibition of d-Asp uptake by d-Asp, THA, and TBOA suggests EAAT1 and/or EAAT3 activity [22]. It should be noted that the substrate specificity in a HCEC culture likely represents the transporter(s) expressed by the majority of metabolically active cells. Further, the EAAT1-5 and xCT (Xc− antiporter light chain) expression levels and distribution within cells of a culture suggests that more than one EAAT may be expressed by a HCEC and that different EAATs may be expressed by Xc− antiporter positive and negative cells.
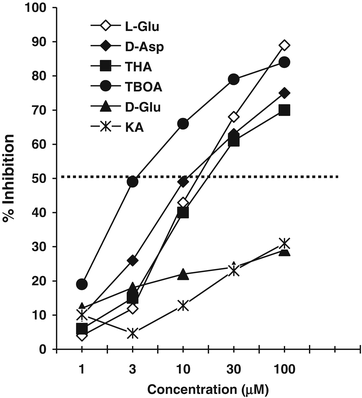
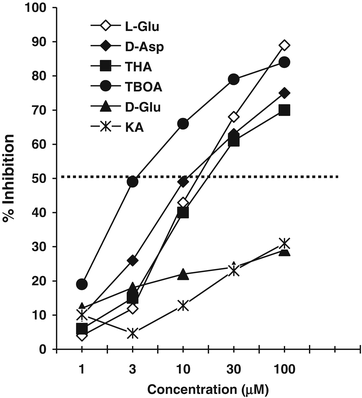
Fig. 4.3
Concentration-dependent inhibition of [3H]-d-Asp uptake in HCEC. Each point represents mean and SEM of three experiments ran in triplicate
Substrate specificity of EAAT and Xc − antiporter activities in HCEC. Na-dependent EAAT and Na-independent Xc− exchanger activities in HCEC were determined by the uptake of radiolabeled l-Glu, d-Asp, and l-Cys in the presence of 100 μM substrate-specific amino acid transporter inhibitors (Table 4.1). The Na-dependent uptake of [14C]-l-Glu by HCEC was inhibited strongly by Xag system substrate inhibitors of l-Glu (76.7 ± 2 %), d-Asp (78.3 ± 5.3 %), and THA (55.5 ± 2 %), but was inhibited weakly by d-Glu (20.6 ± 10.1 %) and KA (33.5 ± 8 %). Inhibition of [14C]-l-Glu uptake by AAA or NAC was not detected (<10 %). In comparison, Na+-dependent [3H]-d-Asp uptake was inhibited strongly by l-Glu (79 ± 0.4 %), d-Asp (79.4 ± 3.4 %), THA (74.2 ± 5.9 %), and TBOA (91.6 ± 4.1 %), but was weakly inhibited by d-Glu (47 ± 10.2 %), and KA (46 ± 8.5 %) consistent with the results of the concentration-dependent inhibition of d-Asp uptake (Fig. 4.3). The weak inhibition Glu or Asp by d-Glu and KA suggests low or not EAAT2 uptake activity in HCEC. Taken together, the results of the Glu/Asp inhibition studies support EAAT1/3 uptake of Glu/Asp in HCEC, but cannot rule out Glu uptake by EAAT2, EAAT4, and EAAT5.
Table 4.1
Substrate-specific inhibition of radiolabeled Glu, d-Asp and Cys uptake in HCEC
Inhibitors | Percent uptake inhibition by 100 μM of: | ||
---|---|---|---|
[14C]-l-Glu | [3H]-d-Asp | [14C]-l-Cys | |
l-Glu | 76.7 ± 2.0 | 79.0 ± 0.4 | 64.6 ± 0.7 |
d-Glu | 20.6 ± 10.1 | 47.0 ± 10.2 | 78.6 ± 2.9 |
d-Asp | 78.3 ± 5.3 | 79.4 ± 3.4 | |
THA | 55.4 ± 2.0 | 74.2 ± 5.9 | |
TBOA | 91.6 ± 4.1 | ||
KA | 33.5 ± 8.0 | 46.0 ± 8.5 | |
AAA | <10.0 | 54.3 ± 5.8 | |
NAC | <10.0 | 86.3 ± 2.6 | |
Cys | 90.7 ± 3.7 |
To define the substrate specificity of Cys transport by HCEC, the uptake of radiolabeled l-Glu and l-Cys was determined in the presence of inhibitors. Cys (90.7 ± 3.7 %), NAC (86.3 ± 2.6 %), and d-Glu (78.6 ± 2.9 %) inhibited Na+-dependent [14C]-l-Cys uptake more than l-Glu (64.6 ± 0.7 %) and AAA (54.3 ± 5.8 %). These results support Na+-independent Cys uptake and suggest that [14C]-l-Cys uptake via Na+-independent Xc− exchanger, but do not rule out Na+-dependent EAAT transport of Cys [33, 34].
Glu and Cys inhibition reduced GSH. Based upon the results of the substrate inhibition studies above, we next determined the effect of Glu and Cys transport inhibitors on GSH in HCEC. GSH levels were reduced most significantly by 6 h incubation in media containing l-Glu (24.3 ± 21.6 %), d-Asp (21 ± 11.7 %), and AAA (24 ± 5.3 %) (Fig. 4.4). GSH levels were reduced less by THA (12 ± 5.7 %) and KA (3 ± 8 %). These results are consistent with the idea that EAAT and Xc− antiporter uptake of Glu and Cys plays a significant role in maintaining GSH in HCEC.


Fig. 4.4
Effect of Glu and Cys transport inhibitors on GSH in HCEC. Each point represents mean and SEM of three experiments ran in triplicate
Cellular distribution of mitochondria, Glu, GGT, and GS in HCEC. Mitochondria (MitoTracker Green dye positive) were concentrated in the cytoplasm around the nucleus of almost all HCEC in a monolayer (Fig. 4.5a). Glu was detected in all HCEC, but most cells contained highly concentrated Glu in the mitochondria-rich perinuclear cytoplasm (Fig. 4.5b). Some HCEC had concentrated Glu over the nucleus suggestive of apical localization. Punctate packets of highly concentrated GGT were detected between most HCEC and GGT was detected weakly on the nuclear membrane of some cells (Fig. 4.5c). Immunoreactive GS was detected in the perinuclear cytoplasm of many cells in the monolayer, but GS signal was absent or uniquely expressed in the cytoplasm of some HCEC (Fig. 4.5d). The results are consistent with high levels of Glu in mitochondria-rich perinuclear cytoplasm. The variable GGT and GS expression by HCEC may suggest metabolic differences consistent with corneal cell differentiation from columnar to the wing epithelia cell phenotype.
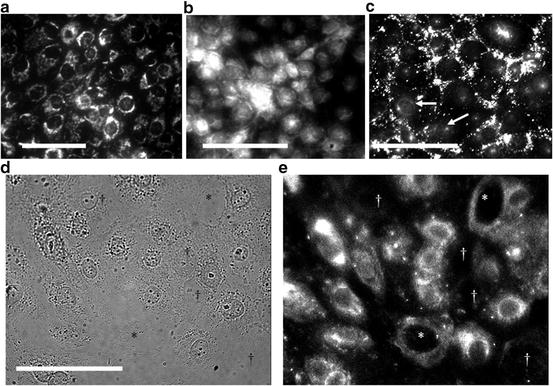
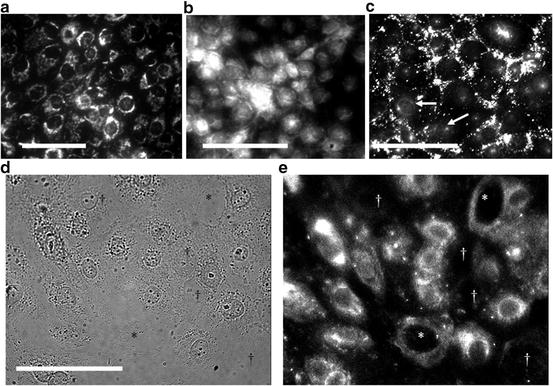
Fig. 4.5
Distribution of (a) mitochondria, (b) Glu, (c) GGT, and (d, e) GS in HCEC. Note the punctate signals of highly concentrated GGT between HCEC and weak signal on nuclear membranes (arrows) of a few HCEC. Also, note GS signals were strong, absent (†), or weak in some putative nucleophagic HCECs. Bar = 50 μm
Effect of GGT and GS inhibition on GSH levels in HCEC. To determine the relative importance of GGT and GS to GSH levels, HCEC cultures were treated with AT125 (5 μg/mL; inhibits >95 % GGT activity; data not shown) [25], 5 mM MSO (inhibitor of GS conversion of Glu to Gln) [26], or BSO (5 mM; inhibitor of GSH synthesis) [27]. The mean percent GSH reduction (±SEM) was determined from three experiments (Fig. 4.6). The GSH concentration in HCEC cultures was reduced by AT125 (19 ± 6.4 %) and MSO (32.7 ± 26.2 %), but was reduced about twofold more by BSO (54 ± 21.4 %). These results suggest the high GSH concentration in HCEC (39.7 ± 2.4 mM) is dependent predominantly on GSH synthesis. Moreover, the results suggest GGT and GS metabolic activities play significant roles in maintaining the GSH levels in HCEC.
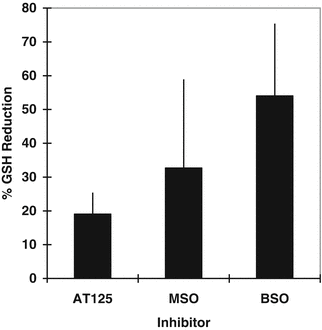
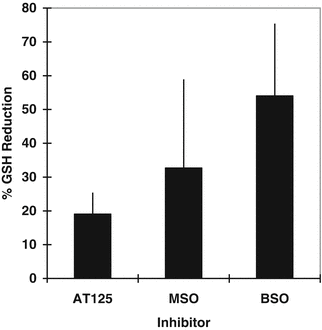
Fig. 4.6
Percent GSH reduction in HCEC treated with AT125, MSO, and BSO for 6 h. Mean and SEM of three experiments ran in duplicate
4.4 Discussion
All EAATs were detected in HCEC as in human cornea [20], but HCEC transport of Glu and d-Asp was via EAAT1/3 activity based upon the Na+-dependent uptake, substrate specificity of d-Asp and Glu uptake, and predominant expression. However, low levels of Glu/Asp uptake, export or intracellular transport by EAAT2, EAAT4, and EAAT5 cannot be ruled out. GSH depletion following EAAT inhibition is consistent with the idea that GSH levels are dependent on Glu transporter activity in HCEC as in retinal cells [33, 34]. Recently, EAAT3 was shown to transport Cys [35, 36] suggesting an alternate pathway for Cys uptake. Moreover, EAAT3 knockout mice are GSH-deficient and susceptible to oxidative stress that is sensitive to antioxidant treatment [37–39]. Thus, the detection of EAAT3 activity in HCEC, as well as in human corneal endothelial and epithelial cells [20], suggests EAAT3 may contribute to GSH synthesis by transporting Cys and Glu in columnar epithelium and endothelium, but additional experiments are needed to confirm the role of EAAT3 Cys transport and protection from oxidative stress in corneal epithelium. Concomitantly, Cys uptake in HCEC was consistent with Xc− antiporter activity based upon Na+-independent uptake activity, sensitivity to Xc− antiporter inhibitors, and xCT (Xc− antiporter light chain) expression on most HCEC in the monolayer. The ≈25 % reduction in GSH following incubation of HCEC with inhibitors of Cys uptake (Glu and AAA) supports the importance of Xc− antiporter in supplying Cys for GSH synthesis [40, 41]. That is, extracellular Glu taken up by EAAT can be utilized by the cell for metabolism and GSH synthesis or exchanged for an extracellular Cys by the Xc− antiporter; providing the essential Cys for GSH synthesis. The coupling of EAAT and Xc− antiporter activities has been shown to protect against GSH loss and oxidative stress [41–43]. The results also suggest that the coupling of EAAT and Xc− antiporter activities in HCEC may protect against oxytosis; cell death due to Glu inhibition of Xc− antiporter Cys uptake leading to a depletion of GSH and susceptibility to oxidative stress [42, 43]. In this regard, high levels of Glu and EAAT dysfunction have been noted in hypertensive and diabetic eyes [44–47]. Our results are consistent with the idea that Glu uptake by EAAT and exchange for Cys by the Xc− antiporter are critical to HCEC Glu, Cys, and GSH homeostasis and protection against oxidative stress.
The expression of GGT on cell and nuclear membranes of HCEC was similar to that in human corneal tissue [13, 20]. The detection of GGT supports GSH recapture via the γ-glutamyl cycle [48]. That is, the catalytic cleavage of extracellular GSH by the ectoenzyme GGT generates free precursor amino acids that can then be taken up by HCEC. The function of intracellular and nuclear membrane-bound GGT in mitochondria-rich HCEC and corneal columnar epithelial cells is unknown, but suggests the presence of the glutaminase-GGT pathway [49]. The ≈20 % reduction in GSH following incubation of HCEC with acivicin (AT125; GGT inhibitor) [24] was similar to the reduction in GSH by Glu and Cys uptake inhibition and was consistent with the idea that GGT activity contributes to the GSH levels. The >2-fold greater reduction in GSH levels by BSO than AT125 suggests that cytosolic/mitochondrial GSH synthesis [50] in HCEC cultures plays a greater role in maintaining the cellular GSH levels than GSH transport. The importance of GGT to GSH and protecting against cell death due to oxidative stress has been shown in vitro and in vivo [51–55]. Thus, loss of corneal GGT due to aging [13], diabetes [14] and other factors would reduce the capacity of the cornea epithelium to maintain GSH via the γ-glutamyl cycle and lessen the antioxidant potential of the cornea.
GS was detected in many of the HCECs in a 48 h culture, but was absent or weakly expressed in ≈25 % of the cells. GS converts intracellular Glu in the presence of ammonia to Gln [26]. The ≈30 % reduction in GSH caused by the GS inhibitor MSO was greater than the reduction caused by EAAT, Xc− antiporter, and GGT inhibitors, but was less than by BSO (inhibitor of GSH synthesis). The reduction in GSH by MSO suggests inhibition of GS activity leads to intracellular Glu accumulation which inhibits Cys uptake by Xc− antiporter (and/or EAAT3) and predisposes the cell to Glu-mediated oxidative stress. This scenario is supported by previous studies in neuronal cells showing GS and Xc− antiporter activities protect neuronal tissue from the toxic effects of Glu and ammonia by generating Gln and exporting Glu [41, 42]. It is not clear why GS is elevated in some HCECs in culture or predominantly expressed in Xc− antiporter positive wing cells in human cornea [20]. However, GS has been detected in ciliary body epithelium [17–19] and retinal pigmented cells [26] where it likely functions to efflux Gln. In addition, GS activity has been shown to regulate Glu-mediated cell swelling [56], enhance clearance of extracellular Glu [57] and protect against neuronal cell death [58]. If GS exerts similar modulatory activities in the corneal epithelium then GS activity could help maintain GSH, protect against endogenous and exogenous oxidative stress, clear the high Glu concentration in columnar cells [20], provide Gln for other corneal cells, reduce Glu produced by dysfunctional mitochondria, and play a role in wing cell morphological changes. Other possibilities cannot be ruled out. Parallel studies using inhibitors of GSH, Glu, Cys, and Gln biogenesis in the corneal epithelium in vivo and in the HCEC model are needed to provide insights into the role of GS in corneal protection against oxidative stress due to endogenous and exogenous factors.
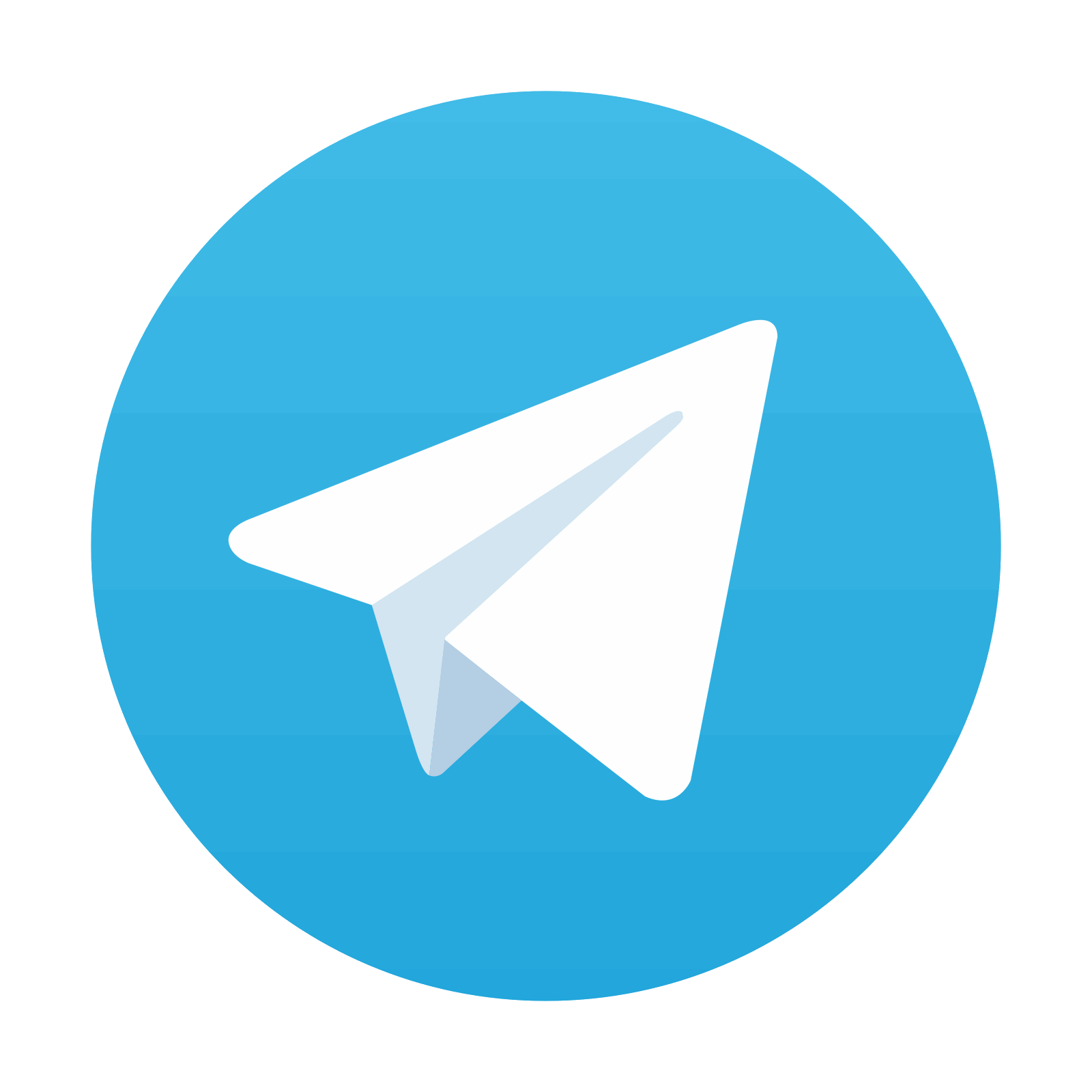
Stay updated, free articles. Join our Telegram channel

Full access? Get Clinical Tree
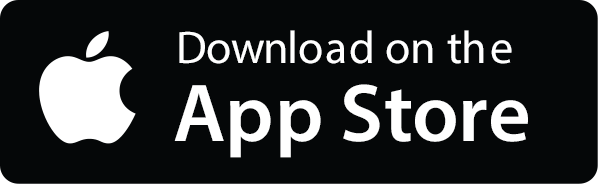
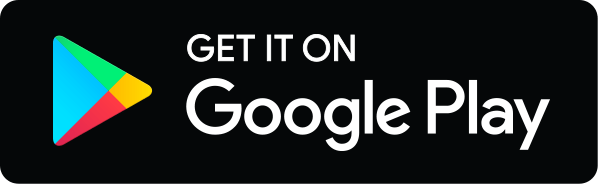