Fig. 6.1
Expression of p63 in the basal epithelium of the human limbus. Representative confocal microscope images of human corneas immunolabeled with antibody specific to the putative stem cell marker p63 (green). Nuclei were counterstained with DAPI (blue). Note the detection of p63-positive signal is stronger at the basal limbal epithelium located within the palisades of Vogt and becomes weaker toward the central cornea (left panels), where this signal is no longer present. Red arrows depict P63-positive cells at the limbus (right panels). Ep corneal epithelium, St cornea stroma. Scale bar left panel: 200 μm and right panel: 100 μm
The discovery of robust markers of LSCs would greatly help in the isolation and expansion techniques for more efficient cell-based therapies. Despite a growing list of phenotypic markers [18, 72, 86], the search for a unique marker to identify LSCs is an ongoing challenge [6, 18]. In 2001, Pellegrini et al. highlighted a nuclear protein called p63 [63], originally described with epidermal development [88], and depicted in Fig. 6.1 in a representative image from a human cornea. Specifically, positive staining for an isoform of p63, ΔNp63, was found in human basal epithelium and not in the central epithelium of human corneas [63]. Later, evidence further supported ΔNp63α as the main p63 isoform located within the basal to intermediate layers of the human limbal and conjunctival epithelia [37]. The presence of several isoforms of ΔNp63 was noted during corneal wounding, in correlation with limbal cell migration and corneal regeneration and differentiation [21]. Another group reported the expression of an ATP-binding cassette transporter group 2 protein, ABCG2, expressed by stem cells from a wide variety of sources [96]. This protein was associated with a group of cells called “side population” cells that facilitated the transport of the DNA-binding dye Hoechst. Additional work by other research groups located the expression of ABCG2 to a side population of corneal limbal epithelial cells; therefore, identifying ABCG2 as a new putative marker for LSCs [5, 84]. Beside p63 and ABCG2, a potential candidate for LSC marker was reported by Di Girolamo et al., who showed selective expression of the low-affinity nerve growth factor receptor p75 to the limbal basal epithelium [20]. In addition to p75, previous groups have noted that limbal basal epithelial cells are positive for cytokeratin 19 [36], and integrin α9 [77]. Negative indirect markers associated with terminal differentiation, such as cytokeratins 3 [71] and 12 [8, 41], involucrin [9], and connexin 43 [53] have been described that help distinguish the LSC phenotype.
The described functional characteristics of LSCs include high proliferative capacity and slow-cycling [12], aspects shared with epidermal stem cells [43] that might signify a strategy for DNA protection [61] and to maintain stemness and quiescence until signaling toward their activation and proliferation is triggered [18].
Although LSCs are widely believed to actively support the renewal of continuously shedding epithelial cells at the surface of the cornea, questions remain as to whether this area is the exclusive source of stem cells for the cornea [25, 82]. Work by Majo et al., introduced the controversial concept that in addition to the limbus, the cornea itself can harbor “stemness” [51]. Specifically, this study showed that serially transplanted mouse corneal epithelium could be self-maintained, and contained oligopotent stem cells. It was noted that these cells were also present throughout the whole cornea surface of the pig [51]. In addition to their findings, the authors proposed that the limbus is a zone of equilibrium where the expanding conjunctiva and corneal epithelium meet. A disequilibrium that can result from, for example, extensive corneal wound would elicit the migration of LSCs into the cornea.
6.2.2 Stromal Stem Cells
Funderburgh et al. [25] first isolated stromal progenitor cells from bovine corneas under the rationale that stem and progenitor cells exhibit clonal growth. Although such clones did not show multipotency, they expressed markers common to mesenchymal stem cells (MSCs), non-hematopoietic cells with the ability for self-renewal and the potential for differentiation into a variety of cell lineages of mesenchymal origin [66]. Markers expressed by stromal progenitor cells included Bmi1, CD90 (Thy1), CD73, CD166, ABCG2, Fhl1, stem cell factor (kit ligand), and Notch1 [25]. The presence of multipotent cells in the stroma was evidenced by several cornea stem cell researchers. A side population of cells from mouse and rabbit stroma were expanded clonally in attachment-free cultures as floating “neurospheres” [2, 91, 92] resembling characteristics of neural stem cells (NSCs) in vitro. Consistent with the rodent findings, work by Du et al. identified stem cells in the stroma of the human cornea [23]. The stromal side population cells termed as corneal stromal stem cells (CSSCs) could be clonally expanded and expressed astrocyte/neuronal-specific markers.
Polisetty et al. [67] used a model of stromal cell culture from human limbal explants to propose that the limbal stroma supporting the limbal epithelium contains a unique population of spindle-shaped cells similar to bone-marrow-derived MSC relative to colony forming efficiency, population doubling capacity, and low immunogenicity. Recent work consolidated the MSC-nature of stem cells in the human stromal limbus [4, 26] and showed that these cells were able to form colonies [26]. Moreover, these cells exhibited immunosuppressant features including inhibition of T cell proliferation in a TGFβ-dependent manner [26].
Similar to what has been found for LSCs, no consensus has been reached regarding a bonafide marker to identify stromal stem cells. As an example, the cell surface glycoprotein CD34 is a controversial marker for stem cells in the stroma [65]. In human cornea, CD34 was reported to be expressed by most keratocytes [35, 76, 80], and also by MSCs as they differentiate into keratocytes after transplantation into the corneas of mice [49]. Although keratocytes no longer express the eye-specific protein PAX6, the corneal stromal stem cells conserve this protein. As a consequence, the expression was reported to be unique for stem cells in the stroma [23, 25].
6.2.3 Maintenance of the Limbal Stem Cell Niche
As introduced earlier, stem cells in the cornea reside within a LSC niche. In the last few years, there has been a concerted effort to understand how the LSC niche is maintained in order to facilitate corneal integrity [7, 56, 60, 87]. As an example, in an effort to elucidate how adult lineage-committed epithelial cells are regulated, Xie et al. [87] isolated both LSCs/limbal progenitor cells and their native niche cells and allowed both cell types to reunite in culture to generate sphere growth. In their model, blocking CXCR4 receptor either with an antagonist or neutralizing antibody, the investigators found a lower capacity of LSCs to form growth spheres as a result of a commitment toward a more differentiated state. These findings suggested the limbal location of corneal LSCs within the LSC niche is critical for the maintenance of their stemness and function, which is mediated by the stromal cell-derived factor-1 (SDF-1) and its receptor CXCR4.
6.3 Stem Cell Deficiencies in the Cornea
Most of the current knowledge about stem cell deficiencies involves the LSC pool which can be partially or totally depleted due to genetic defects, injury or infectious disease [16, 64, 74]. By definition, limbal stem cell deficiency (LSCD) refers to a heterogenous group of pathologies with deleterious effects on corneal integrity and wound healing [70]. As a consequence of stem cell depletion, an invasion of peripheral and central cornea by conjunctival epithelium may take place [61]. Such abnormal tissue localization induces neovascularization of the normally avascular ocular area and corneal opacification leading to impaired visual acuity.
The main conditions that determine LSCD can be grouped according to their hereditary or acquired origins. One of the most common forms of hereditary LSCD, called aniridia is characterized by mutations in the PAX6 gene, a highly evolutionary conserved transcription factor that controls the morphogenesis of all tissues in the eye [34]. In aniridia, there is a “miscommunication” between the developing corneal epithelium and the microenvironment of the anterior compartment of the eye that results in the development of a significant keratopathy not apparent at birth, but progressive from late childhood [11]. The role of PAX6 as a regulator for cell proliferation at the limbus was highlighted in recent work [32]. In this study, PAX 6, along with other putative transcription factors, was able to bind the promoter site of ΔNp63 leading to increased cell proliferation of limbal epithelial cells.
Among the common causes of acquired LSCD are chemical burns (i.e. alkali and acid), thermal burns, contact lens misuse, and direct instilled topical drugs [32, 48, 64]. In addition, acquired forms of LSCD include immune diseases, such as Steven-Johnson syndrome (SJS), characterized by cell apoptosis and necrosis resulting in epithelial detachment, greatly due to adverse drug reactions [64].
6.4 Management of Limbal Stem Cell Deficiency
Eye diseases that result from LSCD translate into a difficult management problem. From the patient’s perspective, the vision loss is partial or complete in either one or both eyes, and often cosmetically unsatisfactory, uncomfortable, or painful [75]. Clinical consequences of LSCD are recurrent or persistent ulceration of the corneal surface with the risk of thinning, vascularization, or infection. Under such circumstances, an effective treatment would offer a significant improvement in quality of life [19, 39, 52]. Conservative treatment options for patients with LSCD include the use of anti-inflammatory drugs, intensive lubrication with non-preserved artificial drops, the use of contact lenses to protect the corneal surface and provide pain relief, and the use of 10–20 % autologous serum eye drops to reduce inflammation and promote epithelial healing [27, 50, 68, 75, 93].
Regarding surgical options, earlier work by Kenyon and Tseng recommended limbal autograft transplantation for treatment of widespread ocular surface damage with loss of limbal epithelial stem cells and, specifically, for ocular surface defects such as those derived from chemical or thermal burns, and contact lens-induced keratopathy [38]. This method is currently in use and offers advantages such as the maintenance of the transplanted LSC in their natural niche, alternative sources of the limbal tissue (deceased donor, autologous tissue from the fellow healthy eye or limbal tissue donated by a living relative), and low cost [75]. An alternative technique, the transplantation of ex vivo expanded limbal epithelial sheets, is a widely used therapy for LSC deficiency [62]. Briefly, this therapeutic approach consists of placing a small limbal biopsy removed from either the patient or a donor onto transplantable carriers such as denuded human amniotic membrane (AM) to support the migration of limbal cells to form a limbal-like epithelial sheet [62, 81]. The success of this type of LSC transplantation is obscured by the depletion of LSC in culture, and requires the optimization of surgical procedures, control of the microenvironment onto which cells are supposed to engraft, and proper pharmacological support [62, 64].
Whether using allotransplantation or autotransplantation, the availability of LSCs seems to always be the limiting factor. For this reason, emphasis has been applied on the identification of potential sources of stem cells from other organs that might serve as autologous sources of replacement in ocular surface diseases, as has been shown for example, in the case of MSCs [50], adult epidermal [10, 54, 55], or oral mucosal [3, 13, 20] stem cells. In the next section of this chapter, we will discuss the use of MSCs for the development of cell-based therapies in the eye.
6.5 Therapeutic Potential of Mesenchymal Stem Cells in Treating LSCD
MSCs, originally isolated from bone marrow, are a type of multipotent cell population that now are shown to reside in almost every type of connective tissue [15] including adipose tissue [94], cord blood [59], and oral tissue [78, 95]. MSCs are characterized as a heterogenous population of cells that proliferate in vitro as plastic adherent cells with spindle-shaped morphology [66]. Based on surface markers, these cells are distinguished from hematopoietic cells by being negative for CD11b, CD14, CD34, CD45, and human leukocyte antigen (HLA)-DR, but expressing CD73, CD90, and CD105. Along with phenotypic markers, the capacity to differentiate into multiple mesenchymal lineages including osteocytes, chondrocytes, and adipocytes is used as criteria to define MSCs [22].
Due to their immunosuppressive properties, MSCs are extensively studied as new therapeutic tools for a variety of clinical applications, such as cases of graft-versus-host disease [46, 45] and autoimmune disorders [79]. In the last few years, encouraging results have highlighted the potential of MSCs in the treatment of ocular surface defects in animal models [33, 50, 57, 90]. However, the potential of these cells to differentiate into epithelial cells as needed to treat LSCD in human patients requires to be further examined.
Since chemical burns are a common cause of LSCD, animal models using this type of injury have been developed in order to test the beneficial effects of bone-marrow-derived adult MSCs on corneal surface repair. For instance, Ma et al. presented a study comparing the therapeutic efficacy of transplantation of human MSCs and LSCs in a rat model [50]. In their work, transplantation of human MSCs on amniotic membrane, like LSCs in amniotic membrane, could reconstruct severely damaged rat corneal surface suggesting a new method to treat corneal disorders. Specifically, it is tempting to speculate based on these results that one could use the patient’s own MSCs to treat their unique corneal disorder without the complications due to allotransplantation-induced immunorejection. Experimentally, the engraftment of systemically transplanted MSCs into locally injured rabbit cornea was shown by Ye et al. [90]. According to this study, MSCs could be home to the alkali-burned cornea, differentiate in response to the local microenvironment, and stimulate local repair response, promoting wound healing upon injury. Along with their repair effect, the presence of MSCs stimulated the proliferation of LSCs which we interpret to suggest that the MSCs that migrated into the wounded limbal and corneal areas exerted a positive influence on the native cornea cell proliferation.
Regarding the mechanism/s of action of MSCs, one group reported the topical application of either MSCs or MSC-derived conditioned media restored the stem cell niche in the chemically burned rat cornea through anti-angiogenic and anti-inflammatory activities of MSCs [57]. When analyzing the levels of soluble factors related to angiogenesis and inflammation, the authors found thrombospondin-1 (TSP-1), a powerful anti-angiogenic factor, was elevated while metalloproteinase-2 (MMP-2), a pro-angiogenic factor, was decreased upon MSC treatment. In addition, MSCs increased the levels of IL-10 and TGFβ resulting in reduced infiltration of inflammatory cells in the chemically burned tissue. Consistent with the restoration effect mediated by soluble factors, further studies revealed other potential therapeutic factors as part of the mechanism(s) of action of MSCs in the damaged cornea, such as the multipotent anti-inflammatory protein tumor necrosis factor (TNF)-α-stimulated gene/protein 6 (TSG-6) [58, 69]. Of interest, and controversial with the idea that MSCs require engraftment into the injured cornea to promote wound repair, another group reported that intraperitoneal and intravenous administration of human MSCs suppressed the development of corneal inflammation and opacity after chemical injury without engraftment of the cells in the cornea [69]. In support of the anti-inflammatory and angiostatic effects of MSCs, downregulation of the macrophage inflammatory protein-1 (MIP-1α), tumor necrosis factor α (TNFα), and vascular endothelial growth factor (VEGF) mRNA expression was found upon subconjunctival injection of MSCs into wounded corneas [89].
The impact of corneal injury on the homeostasis of endogenous MSCs is poorly understood. A recent report presented evidence that as a result of corneal injury, an increase in the frequency of circulating endogenous MSCs and elevated levels of the stem cell-specific chemoattractants SDF-1 and substance P was found in the peripheral blood [42]. These results implied that one or more of the soluble factors such as SDF-1 or substance P may be key to mobilize MSCs to home specifically to the injured cornea. An important question to address when considering the therapeutic application of MSCs in treating LSCD is whether the transplanted cells are able to differentiate into corneal epithelial cells. In this regard, a study found MSCs could be induced to transdifferentiate into corneal epithelial cells by corneal stromal cells in a transwell system and furthermore, such transdifferentiated MSC could then be seeded on a human amniotic membrane in an LSCD rat model [33]. The outcome of this approach was the reconstruction of the corneal epithelium with improved corneal opacity and neovascularization [33].
Beside the use of MSCs originated from the bone marrow to treat damaged corneas, recent work demonstrated intrastromal transplantation of human umbilical MSCs (UMSCs) was successful to treat ocular defects of mucopolysaccharidosis VII mice, a model of human corneal congenital metabolic disease [14]. The findings included human UMSCs survived rejection and differentiated into resident stromal cells, ameliorating the corneal clouding due to abnormal accumulation of glycosaminoglycans in this disease model.
Unlike animal models, evidence based on the efficacy of MSCs to treat human corneal disease is limited. A recent case report described the topical application of autologous adipose-derived MSCs to promote corneal epithelial healing in a patient with post-traumatic persistent corneal epithelial defect refractory to other treatment options [1]. Although these results suggest MSC transfer might be a feasible approach for corneal regeneration, further studies are required to establish the therapeutic efficacy and safety of MSC application in the treatment of corneal disorders.
6.6 Concluding Remarks
Despite some controversial findings it is widely accepted the cornea does not contain stem cells and its epithelial cells are renewed by transient amplifying cells generated by asymmetric divisions of LSCs, residing at the limbus of the eye [30]. Failure to maintain a functional pool of LSCs due to injury, genetic, or infectious disease has detrimental effects on vision by causing LSCD, characterized by invasion of conjunctival cells onto the cornea, and resulting neovascularization and inflammation of the normally avascular ocular surface. Surgical interventions to treat such disease include the successful use of limbal grafts and ex vivo expanded epithelial sheets for transplantation. However, whether using allotransplantation or autotransplantation, the caveat these procedures face is the limited availability of LSCs. The use of stem cells from different sources other than the limbus, such as MSCs, has emerged as a promising therapeutic alternative that in the last decade has been tested beneficial in a variety of animal models of LSCD. Further studies are needed to expand our knowledge on the mechanisms of action of MSCs, and their potential and efficacy as stem cell sources of replacement in ocular surface diseases (see Fig. 6.2 for summary).
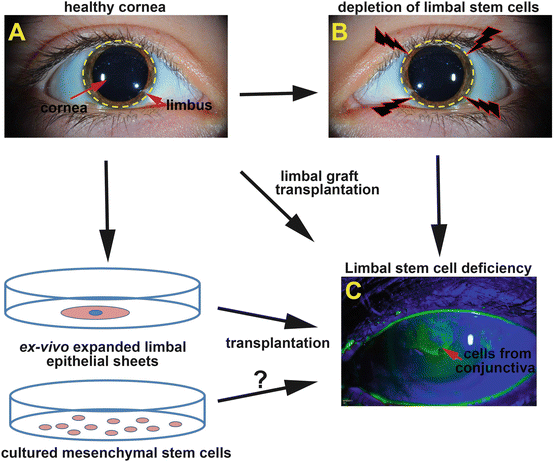
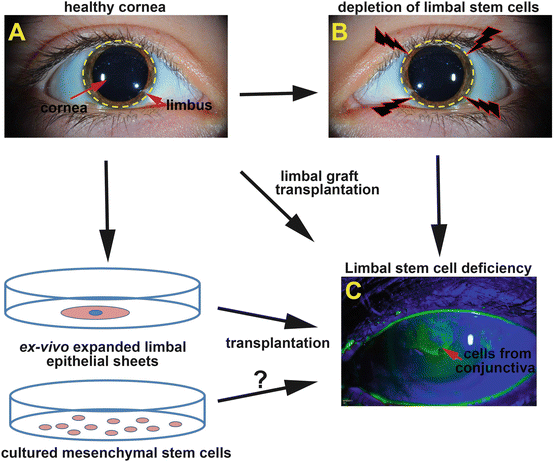
Fig. 6.2
Summary of surgical treatments for LSCD. (a) In a healthy cornea the renewal of central epithelial cells is sustained by LSCs at the limbus (depicted with yellow discontinued lines). Upon depletion of the LSC pool at the limbus (b) LSCD can develop, with clinical features that develop following the migration of cells of conjunctival origin onto the cornea. Such invading conjunctival cells onto the cornea are permeable to the dye fluorescein and can be observed under cobalt blue light by slit lamp examination (green staining) (c). Widely used surgical procedures to treat LSCD are limbal graft transplantations (from healthy fellow eye from the patient or donor eye) and the transplantation of ex vivo expanded limbal epithelial sheets. Ongoing studies are exploring the potential of MSCs as sources of stem cells to treat this disease
Acknowledgment
This work was supported by a grant from the Oklahoma Center for Adult Stem Cell Research through the Oklahoma Tobacco Settlement Endowment Trust.
References
1.
2.
3.
Blazejewska EA, Schlotzer-Schrehardt U, Zenkel M, Bachmann B, Chankiewitz E, Jacobi C, Kruse FE. Corneal limbal microenvironment can induce transdifferentiation of hair follicle stem cells into corneal epithelial-like cells. Stem Cells. 2009;27:642–52.PubMedCentralPubMedCrossRef
4.