Chapter 16 Cell Biology of the Retinal Pigment Epithelium
For additional online content visit http://www.expertconsult.com
Embryology
At day 27 postconception, the optic vesicles invaginate to form the optic cup and the neuroepithelium undergoes early differentiation and thickening. By day 30, the basic cellular relationship of the outer retina is established with the prospective RPE layer found closely apposed to the prospective neural retina. Several transcription factors have been found to play a critical role in the determination and specification of RPE, among which are microphthalmia-associated transcription factor (Mitf), orthodenticle homeobox (Otx)1/2 and paired box (Pax) 6.1 The Sonic hedgehog (Shh) signaling pathway is also critical; the distinct expression pattern of its members in central and peripheral RPE suggests multiple regulatory roles in the process of RPE differentiation.2 By day 35, melanin pigment granules are identified in the presumptive RPE; this is the earliest site of pigmentation in the body. By the 6th week of gestation, RPE cells elaborate basement membrane material that participates in the formation of the first recognizable Bruch’s membrane. Between 4 weeks and 6 months of gestation, RPE cells exhibit a high rate of proliferation that peaks at 4 months of gestation.3 Meanwhile, most of the RPE cells have developed polarized characteristics with apical microvillous projections that extend into the subretinal space.4 The RPE’s consequent interaction with neurosensory retina coordinates the differentiation of primordial photoreceptors. While RPE fate may be reversible for several days following the initial activation of differentiation,5 recent studies suggest that, the Shh pathway,6 retinoic acid,7 bone morphogenetic protein (BMP) signaling,8 Notch9 and Wnt/β-catenin10 pathways all play a role in the maintenance of RPE differentiation. In addition, ezrin, a member of the ezrin/radixin/moesin (ERM) family, is important for morphogenesis of apical microvilli and basal infoldings in RPE cells.11 Polarization of the differentiated RPE monolayer is required for the normal development and function of the photoreceptors and choroid. A role for RPE in the induction and modulation of scleral development has also been described.12 Readers are referred to Chapter 13, Development of the retina, for a more comprehensive review of the development of the RPE and retina.
Anatomy and histology
There are approximately 3.5 × 106 RPE in the adult human eye, and this population remains relatively stable during young adult life in the absence of diseases in which RPE are induced to undergo proliferation or cell death.13 Studies evaluating cell cycle and cell proliferation markers show that a very low level of mature RPE are retained in the cell cycle and proliferate in vivo in both rats and an aged human sample.14 The RPE layer extends from the optic nerve to the ora serrata and continues as the pigment epithelium of the ciliary body. The foveal area contains the highest density of RPE cells, while the cell density exhibits in-gradient decrease to the periphery. These cobblestone-like cells with highly polarized structure form a monolayer between the neurosensory retina and the choroid. The apical microvilli of the RPE cells interdigitate with the OS of the photoreceptors, while the RPE basal side is attached firmly to the underlying Bruch’s membrane; the basement membrane of the RPE represents the innermost layer of Bruch’s membrane. Readers are referred to Chapter 20, Structure and function of Bruch’s membrane, for a detailed description of Bruch’s membrane physiology and interaction with the RPE.
The brown color of the RPE is imparted by its melanin granules and the typical patterned appearance of the fundus results from variations in the pigmentation of the RPE layer. In a healthy retina, the highest concentration of pigment is found in the peripheral retina, the lowest in the macular area.15 With age, RPE gradually lose melanin granules, possibly related to effects of photooxidation.16 The retina and the RPE are separated by a potential space known as the subretinal space. Although the retina conforms to the shape of the adjacent pigment epithelium and underlying sclera, it is not firmly attached to the pigment epithelium except at the optic disc and ora serrata; attachments elsewhere are weak and can be disrupted by relatively weak forces. Readers are referred to Chapter 19, Retinal adhesion and fluid physiology of the subretinal space, for a complete discussion of retinal adhesion.
Heterogeneity and polarity of the RPE
RPE cells exhibit regional heterogeneity in morphology and function.17 The cuboidal RPE cells that form the RPE monolayer appear polygonal in shape when viewed en face, a pattern that is maintained during early passage in culture when grown to confluence (Fig. 16.1). In situ, cell shape varies throughout the fundus; in the macular area the cells are tall and narrow, whereas in the periphery they are flatter, more spread out, and may be binucleated.13 Perhaps due to regional differences in requirements for RPE function, RPE cells vary regionally in growth potential,18 level of expression of vimentin and phosphotyrosine,17 distribution of Na/K adenosine triphosphatase (ATPase) pumps,19 and kinetics of rod outer-segment binding and ingestion.20 Regionally heterogeneous age-related alterations in expression of lysosomal enzymes, Mn-superoxide dismutase, and accumulation of lipofuscin have been identified in RPE,21–23 suggesting that this heterogeneity is probably involved in pathological changes in RPE. Quantification of RPE phenotypic variation can be achieved using laser scanning cytometry.22
The polarity of RPE in the monolayer is characterized by distinct ultrastructural features and specialized functions in the apical and basolateral domains (Fig. 16.2). The apical cell membrane elaborates numerous microvilli (3–7 µm in length) that interdigitate with and ensheath the OS of the retinal photoreceptors.24 (Figs 16.3 and 16.4). These interdigitations, in conjunction with the extracellular matrix (ECM), and neural cell adhesion molecule (N-CAM) expressed on the RPE apical surface, allow some degree of adhesion between the retina and the RPE. Between 30 and 45 photoreceptors are in contact with each RPE cell.25 RPE cells engulf and degrade shed rod OS each day such that one human RPE cell will ingest and degrade hundreds of millions of discs during its lifetime.26
The functional polarity of RPE cells is expressed in the differential distribution of membrane proteins along the apical–basal axis (Table 16.1). Sharing many common characteristics with other transporting epithelia, RPE exhibit “reversed polarity” of certain proteins, such as the Na/K-ATPase pump, ECM metalloproteinase inducer (EMMPRIN), and N-CAM. In RPE these proteins are found at the apical surface, rather than at the basolateral surface where they are found in other epithelia.27 In a proteomic study of RPE apical microvilla,27 283 proteins were identified, which could be divided into different functional categories, including retinoid-metabolizing, cytoskeletal, enzymes, ECM components, membrane proteins, and transporters. αvβ5 integrin (Fig. 16.5), mannose receptors, and CD36 are localized to the apical membrane domain, specifically in the microvilli, where they play critical roles in rod outer-segment phagocytosis.28–30 Na/K-ATPase is mainly expressed at the apical RPE cell membrane where it is critical for transepithelial ion transport in the RPE.31 A newly identified membrane protein chloride intracellular channel 4 (CLIC4) has been shown to be enriched in apical RPE microvilli, possibly for modulating the activity of cell surface channels/transporters.32 The RPE basal membrane domain is characterized by infoldings that are approximately 1 µm in length (Fig. 16.6). The basal membrane expresses α3β1, α6β1, and αvβ3 integrins and mediates attachment to Bruch’s membrane (Fig. 16.5). The basal infolding also express Bestrophin-1, which is a chloride anion channel that regulates voltage-dependent L-type Ca2+ channels by interacting with the beta subunits of the Ca2+ channels.33 Ezrin is found in both apical and basolateral membranes where it promotes morphogenesis of apical microvilli and basal infoldings.34 A number of transporters work together in apical and basal domains. For example, glucose transport from the choroid to the photoreceptors is mediated by glucose transporter (GLUT) 1 located both basally and apically.35 In contrast, elimination of lactic acid from the subretinal space is mediated by different monocarboxylate transporters in the apical (MCT1) and basolateral (MCT3) membranes.36,37 The lateral membrane domain of the RPE cell demonstrates specialized junctions important for cell–cell attachment and communication, which will be discussed below.
Table 16.1 Polarized cell surface molecule expression in retinal pigment epithelium
Location | Protein | Function |
---|---|---|
Apical membrane | Na/K-ATPase | Na flux |
N-CAM | Adhesion to retina, phagocytosis | |
αvβ5 Integrin | Phagocytosis | |
CD36 | Phagocytosis | |
Ezrin | Apical microvilli | |
CLIC4 | Channels/transporters | |
GLUT1 | Glucose transport | |
MCT1 | Monocarboxylate transporter | |
Lateral membrane | Occludin | Tight junction |
Cadherin | Adherens junction | |
Connexin | Gap junction | |
Basolateral membrane | α3β1, α6β1, ανβ3 integrin | Attachment to Bruch’s membrane |
Ezrin | Basal infoldings | |
GLUT1 | Glucose transport | |
MCT3 | Monocarboxylate transporter | |
Bestrophin-1 | Chloride anion channel |
Na/K-ATPase, Na/K adenosine triphosphatase; N-CAM, neural cell adhesion molecule; CLIC4, chloride intracellular channel 4; GLUT1, glucose transporter 1; MCT1, monocarboxylate transporter 1.
The intracellular distribution of cell organelles in RPE also exhibits heterogeneity and polarization (Figs 16.2 and 16.6).38 Melanin granules, which are ovoid or spherical in shape, 2–3 µm in length, and 1 µm in diameter, are localized at the apical region of the cell, as is the endoplasmic reticulum, the site of protein synthesis. At the ora serrata, the RPE contain many dense round melanin granules throughout the cell; at the equator and macula, melanin granules are more apical, less frequent, and often elongated. The nucleus, with a diameter of 8–12 µm, is located in the basal part of the cell. The localization of mitochondria in the basal half of the RPE results from the oxygen pressure which is highest in this cell region and is most apparent at the macula.39 Other cytoplasmic elements, such as microperoxisomes, lysosomes, and phagosomes, do not appear to have a distinct distribution. However, inhibition of lysosomal degradation in RPE cells could induce exocytosis of phagocytic residual material at the basolateral plasma membrane.40
Cellular junctions
The outer blood–retinal barrier is formed by the RPE monolayer, in which the lateral domains of adjacent RPE cells connected by apical zonulae occludens (tight junctions) and adjacent zonulae adherentes (adherens junctions) enable the epithelium to form a barrier by joining neighboring cells together and regulating transepithelial diffusion through the paracellular spaces (Fig. 16.6).41 These junctions seal off the subretinal space where the exchange of macromolecules with the choriocapillaris takes place, and form the so-called Verhoeff’s membrane. Two major groups of proteins of zonulae occludens are the claudins and the occludins. The interaction between the extracellular domains of adjacent occludin molecules leads to high transepithelial resistance and an intact blood–retinal barrier. They are responsible for maintaining the polarity of cells by preventing the lateral diffusion of integral membrane proteins between the apical and lateral/basal surfaces, allowing the specialized functions (such as molecule transport ) of each to be preserved.42 The cytoplasmic domain of occludin interacts with several other proteins, including zonula occludens (ZO)-1 and ZO-2, to form a complex that interacts with the actin cytoskeleton and components of various signal transduction pathways (Fig. 16.7). ZO-1 regulates cell proliferation and gene expression by inhibiting the activity of the Y-box transcription factor ZONAB in cultured epithelial cells, indicating they are critical for the differentiation and homeostasis of the RPE monolayer.43 Early expression of the assembly proteins, including junctional adhesion molecule-A (JAM-A), AF-6, PAR-3, and PAR-6, corresponds to the initial establishment of the adherens and tight junctions,44 whereas diffusible factors secreted by the neural retina act synergistically with basolateral stimulation to regulate the structure and function of RPE tight junctions.45 Claudin 19 has been shown to be dominantly expressed in human fetal RPE cells.46 JAM-C localizes specifically in the tight junctions of human fetal RPE and adult native RPE, and functions to regulate the recruitment of N-cadherin and ZO-1 to the cell–cell contacts.47 In pathological conditions, oxidative stress,48 inhibition of Na/K-ATPase,49 matrix metalloproteinase (MMP)-9, interferon (IFN)-γ, tumor necrosis factor,50,51 and amyloid-beta(1–42)52 could decrease tight junction protein expression, resulting in the disruption of the integrity of the outer blood–retina barrier; in contrast, nitric oxide is involved in the maintenance of blood–retina barrier integrity.51
The zonulae adherentes (adherens junction) form a junction with a separation of 200 Å and are associated with circumferential microfilament bundles.53–55 The transmembrane cadherin molecules of the adherens junction require calcium for ensuring that cells within tissues are bound together. Their cytoplasmic domains interact with catenins, which in turn form a complex with proteins such as α-actinin and vinculin. The adherens junctions play a role in maintenance of the polygonal shape of the RPE cell and in the organization of the actin cytoskeleton.56 Gap junctions, which are also present in the lateral cell membranes, are associated with the expression of connexin and are important for the exchange of ions and metabolites between cells. The gap junction protein connexin 43-mediated communication between the retina and RPE is essential for the correct pacing of retinal organogenesis.57 In addition, ATP released from RPE hemichannels via connexin 43 speeds both cell division and proliferation in the neural retina.58 The basal cell membrane expresses various integrins and shows focal adhesion points with the ECM.59 The presence of desmosomes between RPE cells varies among species; they are often difficult to detect, and they do not appear to be necessary for establishment of a polar, functioning RPE layer.60 Occasional desmosomal–mitochondrial complexes have been described between RPE cells in human specimens, similar in appearance to those observed in nonpigmented ciliary epithelia.61 While the desmosome-associated protein desmoplakin is found in cultured bovine RPE, it has not been identified in human RPE cultures.
Cytoskeleton
Apart from the general role of the cytoskeleton in all nucleated cells, the RPE cytoskeleton is highly associated with its distinct functions such as melanosome transport and phagocytosis.62,63 These functions are disrupted in some diseases like PVR, caused by RPE cell proliferation and myofibroblastic transdifferentiation, where there are prominent rearrangements of the cytoskeleton.64 The cytoskeleton is composed of three major elements: the actin microfilaments (diameter 7 nm), microtubules (diameter 25 nm), and intermediate filaments (diameter 10 nm). Microfilaments and microtubules are dynamic structures that undergo polymerization and depolymerization and are critical for intracellular transport. Microtubules play a role in mitosis, and the movement of subcellular organelles and pigment granules. Actin microfilaments are located in the microvilli and throughout the cytoplasm where they are arranged in loose arrays or bundles. They play an important role in the generation and maintenance of cellular shape and cell migration.65 In fish RPE, actin is involved in melanosome transport within apical projections.66 Myosins are cytoskeletal motors critical for generating the forces necessary for establishing cell structure and mediating actin-dependent cell motility. The expression of multiple myosin family members has been shown in fish RPE.67 In addition, myosins are proposed to play a role in the movement and biogenesis of the melanosomes in RPE.68 Intermediate filaments provide a structural framework for the cell linking the nucleus with the cell membrane and to microfilaments and microtubules. In human RPE cells, intermediate filaments of type I (acidic keratins), type II (basic/neutral keratins), and type V (lamins) have been identified. In RPE, the pattern of cytokeratin filament expression varies with cellular differentiation, changes in cellular polarity, intercellular association, and conditions of cell culture.69,70 Human RPE cells in vivo express keratins 8 and 18.71 Under conditions such as cell culture, keratins 7 and 19 are also coexpressed. The presence of keratin 18/19 has been correlated with migration in cultured RPE cells.71
Role of RPE in Bruch’s membrane synthesis and remodeling
The basal surface of the RPE monolayer has elaborate basal infoldings that attach to Bruch’s basement membrane, an acellular layer separating the RPE from the choriocapillaris.72 Bruch’s membrane is a complex structure that not only serves as the attachment site for the RPE cells, but also serves as a selective conduit for nutrients transported to the retina from the choroidal vasculature and for metabolic wastes transported from the retina to the circulation. Bruch’s membrane consists of five distinct layers.73 From the RPE to the choroid the following layers can be distinguished: the basement membrane of the RPE, the inner collagenous layer, the elastic layer, the outer collagenous layer, and the choriocapillaris endothelium basement membrane. In normal mice the RPE basement membrane and the choroid basement membrane are synthesized first, followed by the deposition of a collagenous layer between the two basement membranes. Later the elastic layer is synthesized and deposited within the collagenous layer, eventually separating it into an inner and outer collagenous layer.74
The basement membrane of the RPE and choroid (1.4–1.5 µm thick) are similar to other basement membranes in composition; they contain collagens, laminin, fibronectin, and sulfated polysaccharides75–77 and serve the same functions, including anchoring subjacent cells, acting as a barrier and a filter, and stabilizing the structure of the tissue.78 The choriocapillaris basement membrane also contains collagen type VI, which is not present in the RPE basement membrane, and may be involved in capillary endothelial cell stabilization. The inner collagenous (1.4 µm thick) and outer collagenous (0.7 µm thick) layers are composed of collagens type I, II, and V organized in a lattice-like network embedded in an amorphous collection of glycosaminoglycans.79
Distributed throughout all the layers of Bruch’s membrane is collagen XVIII,80 which gives rise to the endostatin, an inhibitor of choroidal neovascularization.81 Extensive gene expression studies by Booij et al.82,83 have shown that both the RPE and choroid express most of the genes necessary for the formation and maintenance of Bruch’s membrane. Since both the RPE and choroid are able to synthesize the major components of Bruch’s membrane, it appears that the basal lamina of each RPE would be maintained by RPE cells, the choroid basement membrane by choroidal cells, whereas the inner, outer collagenous layer and the elastic layer would be maintained cooperatively by both tissues. Age-related biochemical alterations in the composition and three-dimensional organization of ECM molecules in Bruch’s membrane may affect this function and are described in detail in Chapter 20, Structure and function of Bruch’s membrane.
The apical domain of the RPE cells is embedded in the interphotoreceptor matrix (IPM), which is produced by the RPE and the inner segments of the photoreceptors. Major protein components of IPM that are involved in retinoid transport between the photoreceptors and the RPE include the interphotoreceptor-binding protein (IRBP), retinol-binding protein (RBP), and transthyretin (TTR).84–87 Retinal adhesion to the RPE is mediated in part by RPE transport of water and ions from the IPM toward the choriocapillaris. It has been identified that RPE predominantly secrete the neurotrophic pigment epithelial-derived factor (PEDF) from the apical side into the IPM, which is about 1000-fold greater than that for vascular endothelial growth factor (VEGF), which is mainly from the basolateral side.88 In addition, proteomic analysis of the porcine IPM indicated that a set of potentially neuroprotective proteins could be extracted, including phosphoprotein enriched in astrocytes (PEA)-15, peroxiredoxin 5, αB crystallin, macrophage migration inhibitory factor, 78-kDa glucose-regulated protein (GRP78), protein disulfide isomerase (PDI), and PEP-19.89 Notably, αB crystallin has been shown to be secreted by RPE and plays an important role in maintaining and facilitating a neuroprotective outer retinal microenvironment.90
Degradation of ECM is regulated, in part, by both MMPs and the urokinase-type plasminogen activator (uPA) cascade,91 which are activated by multiple types of cells including leukocytes, endothelial cells, and RPE. MMPs are a family of zinc-binding, Ca+-dependent endopeptidases that can degrade both collagen and proteoglycans in ECM during physiological and pathological ECM remodeling. The MMPs are tightly regulated by its tissue inhibitors of metalloproteinases (TIMPs), whose impairment may lead to progressive alterations in Bruch’s membrane.92 Normal RPE express the membrane-bound type 1 (MT1 MMP) as well as type 2 (MMP-2) metalloproteinase,93 as well as the metalloproteinase inhibitors TIMP-1 and TIMP-3.94 Degradation of the ECM is also promoted by uPA, a serine protease that catalyzes conversion of inactive plasminogen to active plasmin.95 Plasmin is a broad-spectrum protease that degrades fibrin, fibronectin, laminin, and other ECM components. uPA activity can be blocked by two endogenous inhibitors, plasminogen activator inhibitor-1 and -2 (PAI-1 and PAI-2),96 which belong to the serpin protease superfamily. Cultured human RPE cells produce immunoreactive uPA and a protein similar to PAI-1.97
Cell culture models of RPE
Cell culture models of RPE play a critical role in gaining basic knowledge about native tissue, evaluating polarized RPE function, facilitating drug discovery and toxicity, and offering potential to researchers working in the field of tissue engineering and cell transplantation. Culture of well-differentiated and characterized RPE cells from various sources may be a future cellular therapy for several retinal diseases.98 Methods to generate RPE with varying degrees of polarization have been reported for immortalized human RPE cell lines, such as ARPE19 and D407, and fetal human RPE99,100 (reviewed by Sonoda et al.101 and Maminishkis and Miller102). Polarized RPE monolayers derived from human fetal RPE develop high transepithelial resistance, and show polarized membrane specialization, including prominent apical microvilli and apical expression of Na/Ka-ATPase.101 More recently, cells from the ciliary margin progenitor zone,103 retinal stem cells,104 human embryonic stem cells,105,106 and human induced pluripotent stem cells107 have also been shown to have the potential to be directed into RPE cells. The reader is referred to Chapters 35, Stem cells and cellular therapy, and 125, Transplantation frontiers, for an indepth discussion of RPE cellular therapies.
specialized functions of the RPE
Absorption of light
The first step in the visual process is absorption of light by the opsins of rod and cone photoreceptors and by the melanopsin of retina ganglion cells. Melanopsin is involved in the regulation of circadian rhythms, pupillary light reflex, and other nonvisual responses to light,108 although recently it has been shown that melanopsin in ganglion cells may contribute directly to pattern vision.109 The light-absorbing chromophore of opsins is 11-cis retinaldehyde (11-cis-RAL), which is delivered to the rod photoreceptors by the RPE cells. The RPE cells possess the enzymatic mechanism to convert vitamin A to 11-cis-RAL as well as the mechanism to deliver it to the photoreceptors. An important function of the RPE is the absorption of light that passes beyond the OS of the photoreceptors. The melanin pigment within the pigment granules (Fig. 16.6) of the RPE absorbs stray photons of light which minimizes light scatter within the retina and protects from excess light.15
Phagocytosis of rod outer segments
In all vertebrates examined, nocturnal and diurnal, cold-blooded and warm-blooded, rod OS shedding occurs maximally at or shortly after light onset, a daily rhythm which is established early after birth, irrespective of the lighting conditions during development. In fact, in rats the daily rod OS shedding rhythm is established by 2 weeks postnatally and it is maintained even after optic nerve section, indicating that this rhythmic process of OS shedding is controlled by intrinsic signals within the eye.110,111The temporal pattern of cone OS shedding is much more variable since in some species it occurs at night,112,113 whereas in others cones and rod OS are shed just after light onset.114,115
The disposal of the continuously shed OS is accomplished by phagocytosis of the OS at the expense of elevated metabolic activity and energy expenditure.116,117 It has been estimated that, during an 80-year span, one RPE cell has internalized and degraded about 200 million discs.118 The phagocytosis of ROS disc material can be visualized in normal rat eyes using confocal microscopy to visualize rhodopsin+ phagolysosomes by enucleating the eye 2 hours after onset of light (Fig. 16.8). The mechanisms involved in rod OS phagocytosis have recently been reviewed.119 Rod OS phagocytosis is a highly specialized receptor-mediated, multistep process that comprises recognition, attachment (receptor–ligand interactions), internalization (transmembrane signaling and contractile proteins), and degradation of the ingested OS.119 The first step in the phagocytic pathway is recognition. It appears the recognition is a receptor-mediated event that requires soluble molecules to bridge the receptor on the RPE cell and some functional molecules on the rod OS. Studies have shown that the receptor on the RPE cell is αvβ5 and the molecule on the rod OS is phosphatidylserine, which has been described on the OS plasma membranes.120 Although αvβ3 and αvβ5 have been demonstrated to be instrumental for substrate binding, they do not bind substrate directly. Rather they bind an opsonin that recognizes the “eat-me” signal on their phagocytic targets. A number of soluble molecules have been shown to be possible bridging elements, such as Gas6, protein S, and milk fat globule epidermal growth factor (MFG)-E8.121 Recently Tubby and tubby-like protein (Tulp) 1, which are secreted by photoreceptors, have been identified as playing a role in RPE phagocytosis. Tubby and Tulp1 are bridging molecules that bind to MerTk (a member of the TAM receptor tyrosine kinase subfamily) at their N-terminal region and likely to the rod OS at their C-terminal region.122
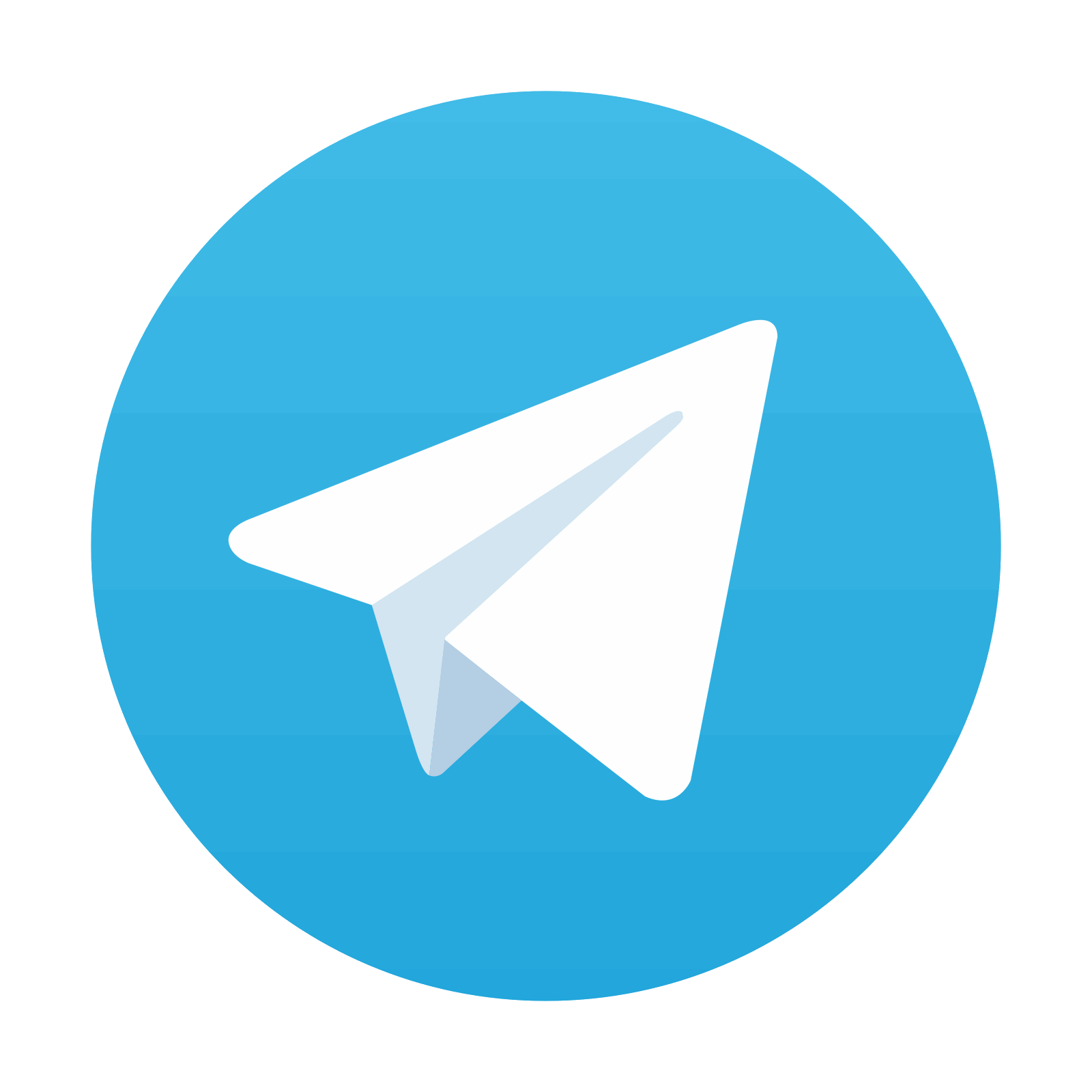
Stay updated, free articles. Join our Telegram channel

Full access? Get Clinical Tree
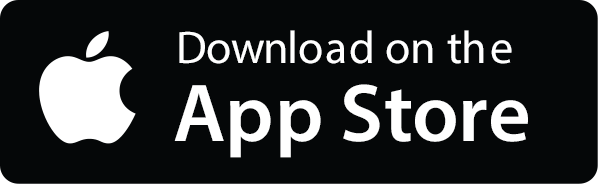
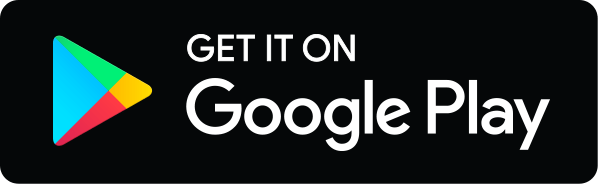