22 Retinal Manifestations of Metabolic Disease
22.1 Introduction
While the genetic basis and systemic manifestations of many metabolic diseases are quite diverse, their range of retinal expression can be divided into two main categories. Those causing cherry-red spot (Fig. 22-1) are listed in List 1; those resulting in pigmentary retinopathy (Fig. 22-2) are given in List 2. Pigmentary changes in the temporal macula at the insertion of the long ciliary nerve can be seen in infants and young children and are sometimes confused for an “eccentric cherry-red spot” (Fig. 22-3). This is a normal finding and typically is not seen as the fundus pigmentation increases with age. A cherry-red spot is always at the fovea. A summary of the genetic etiologies of these metabolic diseases can be found in Table 22-1.



Diseases Causing Cherry-Red Spots
Storage diseases
GM1 gangliosidosis
GM2 gangliosidosis (Tay–Sachs and Sandhoff)
Niemann–Pick types A and B
Sialidoses
Farber’s lipogranulomatosis
Other etiologies
Central retinal artery occlusion
Traumatic retinal edema
Macular hole with surrounding cuff of subretinal fluid
Metabolic Diseases Causing Pigmentary Retinopathy
Lysosomal storage diseases
Mucopolysaccharidoses
Hurler–Scheie syndrome
Hunter’s syndrome
Sanfilippo’s disease
Peroxisomal diseases
Zellweger’s spectrum disorders (Zellweger, neonatal adrenoleukodystrophy, infantile Refsum)
Refsum’s disease
Primary hyperoxaluria
Disorders of amino acid and lipoprotein metabolism
Cystinosis
Gyrate atrophy
Abetalipoproteinemia
Hypobetalipoproteinemia
Others
Mucolipidosis IV
Ceroid lipofuscinoses
22.2 Lipidoses
The sphingolipidoses are a type of lysosomal storage disease in which glycolipids with a sphingosine base are not degraded properly, leading to an accumulation in cytoplasmic vesicles.
22.2.1 GM2 Gangliosidoses (Tay–Sachs disease and Sandhoff’s disease)
Tay–Sachs and Sandhoff’s diseases are characterized by storage of GM2 ganglioside in the lysosomes of neuronal tissue, which results in progressive loss of neurologic function. Tay–Sachs disease is most prevalent in Ashkenazi Jews, with a carrier rate estimated to be 1 in 30 to 1 in 40 among European Jews living in the United States, compared with 1 in 300 among non-Jews. 1 It is also more common in individuals of Pennsylvania Dutch, Southern Louisiana Cajun, or Eastern Quebec French Canadian descent. 1 No single ethnic group has an increased rate of Sandhoff’s disease. Instead, several geographically or demographically isolated populations have higher incidences, such as in the town of Cordoba in Argentina, where 36 cases in 15 families have been detected in 3 years. 2
Clinical Features
Infantile Tay–Sachs is the archetype of the gangliosidoses. It is characterized by early onset, at age 3 to 5 months; hyperirritability; and advancing weakness. These infants rapidly lose motor and intellectual skills after the age of 1 year and may crawl but never walk. The natural course leads to a decerebrate, vegetative state by the second to fourth year of life. Visual inattentiveness is frequently the presenting complaint, and the ocular stigma, a macular cherry-red spot, is found early in all patients. Additionally, infants are commonly macrocephalic from accumulation of storage material in the brain. A juvenile form of Tay–Sachs exists; this has a later onset and slower course but a similar constellation of signs and symptoms. The mildest form of the disease, the adult subtype, has its onset in the 20s or 30s. Presenting manifestations include ataxia, dysarthria, muscle weakness, and psychosis. One final variant, dubbed chronic, presents between ages 2 and 5 years and has a more gradual course, with patients surviving well into adulthood. The vast majority of patients have the infantile form. 3 , 4
Pearls
In Tay–Sachs disease, the genetic deficiency of ß -hexosaminidase A results in abnormal accumulation of GM2 gangliosides in the lysosomes of neuronal cells. The macular cherry-red spot, a consistent and early finding, is caused by swelling and opacification of the ganglion cells around the fovea.
Although biochemically distinct, Sandhoff’s disease has a similar range of presentations and clinical features. However, patients with Sandhoff’s disease may also have organomegaly and occasional bony abnormalities. 2
Diagnosis
Diagnosis of the GM2 gangliosidoses is accomplished by assaying for the activity of individual ß-hexosaminidase isoenzymes in serum or cultured cells from affected individuals. Deficiency in the ß-hexosaminidase A isoenzyme is found in Tay–Sachs disease, whereas deficiency in ß -hexosaminidase A and B isoenzymes is detected in Sandhoff’s disease. Heterozygous carriers can be detected by a reduced level of activity for ß-hexosaminidase. 1 In addition to biochemical testing, carrier state testing can be performed by DNA analysis. This is especially true in the Ashkenazi Jewish population, in which three mutations appear to be responsible for the vast majority of cases. Testing identifies 99.9% of carriers in the Ashkenazi Jewish population, with a false-negative rate of less than 2%. 5
Pathogenesis
ß-Hexosaminidase is a lysosomal acid hydrolase composed of two subunits, a and ß, which can pair to create three isomeric forms: a/a, a/ß, and ß/ß. Only the a/ß isoenzyme, ß-hexosaminidase A, can degrade GM2 gangliosides. Thus, deficiency of either a or ß subunits results in accumulation of GM2 gangliosides. 3 Loss of ß subunit activity also causes deficiency of ß-hexosaminidase B (ß/ß) and accumulation of globoside in the viscera. 6 Tay–Sachs disease is caused by mutations in the a subunit gene on chromosome 15, and Sandhoff’s disease results from mutations in the ß subunit gene on chromosome 5. The onset and severity of the disease process correlate directly with the amount of residual enzymatic activity in the mutant subunit. Any mutations that cause complete loss of enzymatic activity in the homozygous state, such as a frameshift, splicing, or premature stop codon mutation, lead to the severe, rapidly progressing, infantile phenotype. Individuals with point mutations resulting in single amino acid substitutions often retain some enzymatic activity and, therefore, have a later onset and more gradual course of the juvenile or adult phenotype. Intermediate expressions may also occur in patients who are heterozygous for two mutant alleles, one severe and one mild. 3
Gangliosides are most plentiful in the gray matter; it is not surprising, then, that most of the clinical manifestations and pathology center on the nervous system. Meganeurites, neurons distended with storage material, are the pathologic hallmark of the GM2 gangliosidoses and are found throughout the nervous system. In the retina, ganglion cells and amacrine cells are swollen with storage material. The concentration of enlarged, opacified ganglion cells encircling the fovea results in the cherry-red spot. The storage material in Tay–Sachs disease is composed ultrastructurally of membranous cytoplasmic bodies with concentrically arranged layers. 2 In contrast, the storage material in Sandhoff’s disease is more pleomorphic, containing fibrogranular vacuoles, membranous cytoplasmic bodies, and zebra bodies with stacked lamellae. 6
Management and Course
Management is mostly supportive. It is unknown whether bone marrow transplantation would alter the neurologic consequences of these diseases significantly.
22.2.2 GM1 Gangliosidosis
GM1 gangliosidosis is a rare, autosomal recessive disorder with an unknown incidence. 7
Clinical Features
GM1 gangliosidosis has a broad range of clinical expressions, which are divided by age of onset into infantile, juvenile, and adult forms. The infantile form is the most common and severe phenotype. Afflicted infants appear normal at birth but suffer developmental arrest and then regression by 3 to 6 months of age. Failure to thrive, hypotonia, hyperirritability, and seizures are prevalent manifestations. Hepatosplenomegaly, dysmorphic facies, and dysostosis multiplex are found less consistently. Death ensues by the age of 2 years. The juvenile form has a later onset and more gradual course but similar clinical features. The chronic adult form is dominated by spastic ataxia; the dysmorphic and visceral expressions are less severe. In terms of ocular findings, a macular cherry-red spot is found in about 50% of affected infants and has been reported in some juvenile cases. 4 , 7 Additional ocular findings include visual inattentiveness, corneal clouding, tortuous retinal vessels, retinal hemorrhages, and optic atrophy. 8
Morquio type B disease shares the same biochemical error but is clinically distinct from GM1 gangliosidosis. Patients are normal at birth, but growth retardation and progressive, generalized skeletal dysplasia develop in the first decade of life. The nervous system is not involved, and patients are of normal intelligence. Corneal clouding is the only ocular expression of the disease. 7
Diagnosis
Diagnosis is based primarily on markedly decreased or absent ß-galactosidase activity in cultured leukocytes or fibroblasts. Tears may also be used for enzyme assays. Prenatal diagnosis can be made by assaying enzyme activity in cultured amniocytes or chorionic villus cells. Morquio type B is differentiated from GM1 gangliosidosis by the predominance of skeletal abnormalities in the absence of neurologic involvement and the high concentrations of keratan sulfate in the urine. 7
Pathophysiology and Histopathology
Mutations in the ß-galactosidase 1 (GLB1) gene on chromosome 3 result in accumulation of ganglioside GM1. The gangliosides are the major storage compounds in GM1 gangliosidosis, whereas keratan sulfate is the dominant storage substance in Morquio B disease. Thus, the phenotypic differences between GM1 gangliosidosis and Morquio B may be the result of altered enzymatic substrate specificity. Higher levels of residual enzyme activity correlate roughly with the later onset and milder phenotype.
By light microscopy, meganeurites similar to those seen in Tay–Sachs disease are found throughout the central nervous system. The ultrastructural characteristics of the storage material are also similar. 7 In the retina, there is extensive ganglion cell loss, and the remaining cells are greatly distended with storage vesicles. Electron microscopy demonstrates numerous clear vacuoles in the cell bodies of rods and cones and membranous cytoplasmic bodies in the bipolar and ganglion cells. 9
Management and Course
No therapy has proved effective in GM1 gangliosidosis. Bone marrow transplants in a canine model failed to alter the course of the disease. 7
22.2.3 Gaucher’s Disease
Gaucher’s disease is the most common of the lysosomal storage diseases, with an incidence of 1 in 10,000 to 20,000 Americans. Type 1 is the most prevalent (90% of cases) and occurs with greater frequency in the Ashkenazi Jewish population, while types 2 and 3 are less common and occur across all ethnic groups. 10 , 11
Clinical Features
Affected patients are divided into three categories: type I, nonneuropathic (adult); type II, acute neuropathic (infantile); and type III, subacute neuropathic (juvenile). As in GM2 gangliosidoses, an earlier onset coincides with more severe disease and rapid course. These categories are actually arbitrary divisions in a continuous clinical spectrum; thus, there is considerable variation within each group. Type I patients may be completely asymptomatic or may display some or all of the following: anemia, thrombocytopenia, hepatosplenomegaly, and bone lesions. The adult-onset type is most common among the Ashkenazi Jewish population. The infantile, neuropathic form, type II, is much rarer and occurs panethnically. Infants with type II disease present in the early months of life with hepatosplenomegaly and neurologic deterioration. Death usually occurs before the age of 2 years. Patients with the juvenile type of disease demonstrate neuronal and visceral involvement; the neurologic complaints develop later in life and progress more slowly than in the infantile form. 3 , 9 , 12 The most consistent ocular finding reported is that of scattered, superficial, retinal white spots concentrated in the posterior pole, especially along the inferior vascular arcade (Fig. 22-4). In older patients, a peculiar, pigmented, brown, triangular pingueculum may develop that contains Gaucher’s cells, which are characteristic macrophages with foamy, lipid-laden cytoplasm. 13

Pearls
Gaucher’s disease is the most common lysosomal storage disorder. The most common fundus finding is that of multiple, superficial white dots in the posterior retina, particularly around the inferior vascular arcade.
Diagnosis
Diagnosis is made by analyzing leukocyte acid ß-glucosidase glucocerebrosidase activity. Although heterozygotes have, on average, 50% of the activity of normal subjects, the range of normal is so wide that the usefulness of enzyme assays to detect heterozygotes is limited. The finding of large numbers of Gaucher’s cells on bone marrow aspirate is characteristic. 12 Mutation analysis can also confirm the diagnosis, predict clinical manifestations, and identify undiagnosed family members and carriers. 14
Pathogenesis and Histopathology
Gaucher’s disease is caused by mutations in the glucocerebrosidase (also called acid ß-glucosidase, GBA) gene on chromosome 1q21. More than 200 different mutations have been identified, with greater than 80% of them single-nucleotide substitutions. 15 Mutations in this lysosomal enzyme glucocerebrosidase lead to an accumulation of glucocerebroside within macrophage lysosomes. 16 Clinical manifestations result in the accumulation of lipid-laden macrophages in the spleen, liver, bone marrow, and bone, among other tissues/organs. 17 Gaucher’s disease is a spectrum of disease manifestations, related to specific mutations rather than a disorder with separate subtypes. Recombination and insertion or deletion mutations cause neuropathic disease, whereas point mutations may cause either neuropathic or nonneuropathic disease. 12 This makes sense conceptually, because point mutations are more likely to result in enzymes with residual activity. In fact, considerable phenotypic variability can be found in siblings with identical genotypes, implying that environmental or other associated genetic factors can modify phenotypic expression. 9 Afflicted individuals may also be heterozygous, possessing two different mutant alleles for Gaucher’s disease. These patients usually have an intermediate age of onset and rate of progression compared with what is predicted for homozygotes of each allele. 3
Although the glucocerebrosidase deficiency exists in all cells, the phenotype is expressed only in the macrophage/monocyte system in the nonneuropathic forms, and also in the neurons in the neuropathic form. 12 By light microscopy, abnormal macrophages, called Gaucher’s cells, which are filled with foamy, lipid-laden cytoplasm, are found in bone marrow, spleen, and liver. Histologically and ultrastructurally, the white spots in the fundus represent Gaucher’s cells. 10 , 18
Management and Course
Nonneuropathic Gaucher’s disease is an ideal candidate for a variety of treatment approaches because it affects mainly macrophages, which are derived from bone marrow stem cells and are in constant contact with the bloodstream. Enzyme-replacement therapy (ERT) with recombinant glucocerebrosidase (imiglucerase, velaglucerase alfa, and taliglucerase alfa) is recommended for symptomatic children and those with severe manifestations of nonneuropathic (type 1) Gaucher’s disease. It is also recommended for those with neuropathic type 3 disease with severe visceral symptoms and those at risk for type 3 disease (genotype or family history). It is not recommended for type 2 disease because it unfortunately does not alter the fatal neurologic outcomes. 19 , 20 , 21 , 22 , 23 , 24 , 25 , 26 Overall, ERT is individualized to each patient. Its high cost (> $400,000 per year) also makes it necessary to determine lowest effective initial and maintenance dose. 9 Hematopoietic stem cell transplantation was a treatment option previously but, given the associated morbidity and mortality, has been largely replaced by ERT. 27 , 28
22.3 Sphingomyelin Lipidoses
22.3.1 Niemann-Pick Disease
All the sphingomyelin lipidoses, types A through F, are characterized biochemically by accumulation of sphingomyelin, particularly in the reticuloendothelial system. Only types A and B consistently have macular findings. The disease is panethnic but has the highest frequency among the Ashkenazi Jewish population, in which the incidences of types A and B disease are 1 in 40,000 and 1 in 80,000, respectively. 29 , 30 , 31
Clinical Features
Type A, the acute neuropathic form, has its onset in early infancy. Affected infants exhibit progressive psychomotor retardation with massive hepatosplenomegaly and usually die by 3 years of age. The nonneuropathic type B has a slightly later onset in infancy than type A, and affected individuals manifest only visceral symptoms. Pancytopenia may occur secondary to hypersplenism, and pulmonary compromise can result from alveolar involvement. 30 , 31
The ocular stigmata of the acute neuropathic form include corneal stromal opacities, cataracts, and cherry-red spots. 32 Macular cherry-red spots are eventually present in all affected individuals with type A, although they may not be observed initially. Macular halos, the mildest expression of a macular cherry-red spot, may be present in children with type B disease 33 (Fig. 22-5).

Pearls
In Niemann–Pick disease, only types A and B have clinically evident retinal changes. Cherry-red spots and macular halos are found in the neuropathic and nonneuropathic types, respectively.
Diagnosis
The diagnosis is based on the clinical findings, course of the disease, and testing for deficiency of sphingomyelinase activity in leukocytes. 3 The presence of Niemann–Pick cells (described later) in bone marrow aspirates supports the diagnosis of Niemann–Pick disease, but the diagnosis should be confirmed by enzyme assays. Definitive diagnosis of types A and B requires residual acid sphingomyelinase activity that is less than 10% of controls. 34 Prenatal diagnosis can be made by enzymatic or molecular analysis of cultured amniocytes or cells from chorionic villi. 35
Pathogenesis and Histopathology
Niemann–Pick types A and B are caused by mutations in the acid sphingomyelinase gene (SMPD1) on chromosome 11p15. Mutations associated with type A disease result in complete absence of acid sphingomyelinase activity, leading to lysosomal accumulation of sphingomyelin. 36 , 37 , 38 Mutations associated with type B disease result in acid sphingomyelinase deficiency with some residual activity of the enzyme. 37 , 38 , 39 Comparison of enzymatic activity found that type B had 4% of normal acid sphingomyelinase activity while type A had undetectable activity. 37 , 39
Distinctive foam cells, labeled Niemann–Pick cells, can be found in all affected tissues. However, their presence is not pathognomonic because similar cells can be observed in Tay–Sachs and several other metabolic diseases. Niemann–Pick cells are formed by lipid accumulating in bone marrow progenitor cells. They can be distinguished from Gaucher’s cells by the smaller size, positive test for cholesterol, and weak staining for acid phosphatase. Ultrastructurally, Niemann–Pick cells contain granular lipid inclusions that may appear lamellar, like the membranous cytoplasmic bodies found in the neurons of patients with Tay–Sachs disease. In neuropathic disease, ganglion and glial cells of the central nervous system are distended, pale, and filled with large vacuoles. The glial cells may resemble foam cells. 35 In type A disease, membranous cytoplasmic bodies have been found in numerous ocular tissues, including keratocytes; endothelial cells; lens; retinal pigment epithelium (RPE); and fibroblasts of choroid, sclera, and extraocular muscles. 40
Special Considerations
The foam cells of Niemann–Pick disease are not pathognomonic for the condition, as similar cells can be observed histopathologically in Tay–Sachs and other metabolic diseases.
Management and Course
Unfortunately, the management of all forms of Niemann–Pick disease is supportive.
22.4 Mucopolysaccharidoses
The mucopolysaccharide (MPS) storage disorders are lysosomal storage disorders caused by a deficiency of enzymes required for the breakdown of glycosaminoglycans (GAGs) leading to the accumulation of partially degraded GAGs intracellularly and extracellularly in many tissues and in the urine. 41 , 42 , 43 All are progressive disorders and are inherited as an autosomal recessive trait except for Hunter’s syndrome, which is an X-linked recessive trait. Of the six types, MPS I, II, and III exhibit retinal degeneration, whereas MPS I, IV, VI, and VII display corneal clouding.
22.4.1 Mucopolysaccharidosis Type I-H (MPS I-H, Hurler’s Syndrome) and Type I-S (MPS I-S, Scheie’s Syndrome)
Mucopolysaccharidosis type I (MPS I) is caused by a deficiency of the lysosomal hydrolase, alpha-L-iduronidase (IDUA gene), which is needed for the degradation of heparin sulfate and dermatan sulfate. 44 MPS I includes Hurler (MPS I-H), Hurler–Scheie (MPS I-H/S), and Scheie (MPS I-S) that represent a spectrum of severity. 45 The incidence of Hurler’s syndromes in British Columbia is estimated to be 1 in 10,000 births. 46 Scheie’s syndrome is very rare, with an incidence of 1 in 600,000 in British Columbia. 41
Clinical Features
The Hurler’s and Scheie’s syndromes are now recognized to share the same biochemical defect and represent the extremes of a continuous spectrum of disease. In early infancy, patients with Hurler’s syndromes often appear normal, but within the first year of life they manifest abnormalities such as coarse facies, large head, thick lips, enlarged tongue, airway problems, cardiac disease, hydrocephalus, hepatosplenomegaly, stiff joints, dysostosis multiplex, umbilical hernias, deafness, and mental retardation. Short stature becomes apparent by 2 to 3 years of age. Premature death usually ensues by the age of 10 years from cardiorespiratory failure. 41 , 47
Corneal clouding associated with photophobia is often the first ocular manifestation of the MPS I-H and may progress to a point at which the fundus is obscured from view. It is caused by structural changes in the corneal stroma, including abnormalities of size, spacing, and arrangement of collagen fibrils. 48 Glaucoma has also been reported. 49 , 50 A retinal dystrophy with bone spicules develops, and the electroretinogram (ERG) is reduced or extinct. 50 , 51 Optic nerve head edema and subsequent optic atrophy are frequently observed. Enlargement of the optic nerve is caused by accumulation of acid mucopolysaccharides and leads to compression of the optic nerve at the lamina cribrosa or optic canal. 52
The systemic manifestations of Scheie’s syndrome are similar to those of Hurler’s syndrome but less severe. Patients with Scheie’s disease have a later onset of symptoms, which appear by 5 to 7 years of age. In one series of 10 patients, the most common presenting features were joint stiffness and corneal clouding. 45 Patients with Scheie’s syndrome have normal stature, intelligence, and life expectancy. The diagnosis is made most commonly in the second decade of life. In a milder form, they share with Hurler’s patients coarse facies, stiff joints, dysostosis multiplex, hernia, and deafness. Because of their longer life span, they also frequently are affected by carpal tunnel syndrome and aortic valvular disease, which result from accumulation of acid mucopolysaccharides at these sites. Orthopedic complications often dominate the long-term clinical picture. Crippling loss of hand function occurs secondary to carpal tunnel syndrome and progressive joint stiffness. Spondylolisthesis can lead to chronic back pain and occasionally results in spinal cord compression. 47
The ocular features in Scheie’s syndrome, like the systemic features, have a delayed but similar presentation to those of Hurler’s syndrome. Corneal clouding becomes apparent in the second decade of life. It is most prominent peripherally and tends to be slowly progressive. Glaucoma frequently develops in the fourth and fifth decades of life. The retinal degeneration manifests as nyctalopia by age 20 to 30 years, and the fundus changes mimic those of retinitis pigmentosa. 50
Diagnosis
Measurement of urinary GAG concentration, fractionation of GAG by electrophoresis or chromatography, and analysis of oligosaccharides can identify the type of MPS, oligosaccharidosis, or other storage disorders. Definitive diagnosis requires assay of alpha-L-iduronidase enzyme activity, usually in peripheral blood leukocytes. Differentiation between MPS type I Hurler and Scheie is based on the differences in clinical features and course (i.e., later onset, and lack of neurologic impairment in Scheie’s disease). Carriers can also be detected by this method, as they have 50% or less of the a-L-iduronidase activity of normal controls. Prenatal diagnosis can be made by assaying cultured amniotic fluid cells by similar methods. 41 , 53 Conjunctival biopsy with ultrastructural study can assist in the diagnosis of storage diseases, but the changes are not specific to any one entity. 54 Mutation analysis is limited by the high frequency of “private” mutations, although analysis can be performed when the suspected mutation is known. 55 , 56 , 57 , 58
Pathogenesis and Histopathology
The loss of a-L-iduronidase activity results in the buildup of intracellular heparin sulfate and dermatan sulfate, which are acid mucopolysaccharides that cannot be degraded and are deposited in swollen lysosomes. 49 Although the correlation between a-L-iduronidase activity and clinical severity is not absolute, most patients with severe disease have no detectable enzyme activity. The a-L-iduronidase gene (IDUA) has been cloned, and several mutant alleles have been analyzed. As expected, the more serious mutations resulting in frameshifts or premature stop codons are associated with little or no active enzyme and result in the more severe Hurler’s phenotype. Point mutations frequently are associated with enzyme production and residual activity and result in the milder Scheie’s phenotype. 3
Chan and colleagues found abnormalities by electron microscopy in all ocular tissues of two patients without clinically detectable retinal degeneration. 54 They observed multimembranous inclusions in retinal ganglion cells and optic nerve astrocytes as well as fine fibrogranular inclusions in the RPE.
Management and Course
Currently, the only available therapy for MPS I is an allogenic bone marrow transplant. Patients with Hurler’s disease receiving such transplants have improved visceral symptoms but show no improvement in skeletal abnormalities. The neurologic and intelligence outcomes have been variable, but a small number of patients who received early bone marrow transplants, before the age of 2 years, have developed normally, even though their genotypes would predict a severe Hurler’s phenotype. 41 HLA-matched bone marrow transplants from siblings have the best chance of success, but when engraftment is achieved, unrelated donor transplants have been equally efficacious for visceral and neurologic symptoms. 59 Short of bone marrow transplant, treatment is limited to management of the disabling systemic and ocular manifestations, such as orthopedic problems, glaucoma, and corneal clouding.
22.4.2 Mucopolysaccharidosis Type II (MPS II, Hunter’s Syndrome)
The incidence of Hunter’s syndrome is estimated to be 1 in 150,000 in British Columbia and 1 in 67,500 in Israel. 46 , 60 Hunter’s syndrome and adrenoleukodystrophy are the only two X-linked traits discussed in this chapter.
Clinical Features
Hunter’s syndrome shares many features with the MPS type I syndromes. It presents in two forms: a severe, infantile form in which symptoms develop by 1 year of age and a less severe, childhood form with an older age of onset, at about 4 years. The infantile form is predominant. Affected individuals are generally mentally retarded and die before 15 years of age. Those with the childhood form have near-normal intelligence and life span. In both forms, coarse facies, immobile joints, short stature, airway problems, and hoarse voice gradually develop. The skeletal involvement tends to be milder in Hunter’s syndrome than in Hurler’s syndrome. One distinctive but rare feature of Hunter’s syndrome is ivory-colored, nodular skin lesions affecting the neck, back, pectoral regions, upper arms, and thighs. The mildly afflicted patients often have cervical myelopathy in later life resulting from dural hyperplasia and thickening of the ligamenta flava. 41 , 47
A progressive retinal degeneration with reduced ERG responses is the major ocular manifestation of Hunter’s syndrome. The corneal clouding characteristic of the MPS type I syndromes is absent. 50 Chronic optic nerve head edema has been observed in the childhood form of Hunter. 41
Diagnosis
Diagnosis is made by assaying for activity of iduronate sulfatase in cultured leukocytes. 41
Pathogenesis and Histopathology
Hunter’s syndrome is caused by a deficiency of iduronate 2-sulfatase, the gene of which (IDS gene) is located on chromosome Xq28, and results in lysosomal accumulation of heparin and dermatan sulfate. 61 Deletion of contiguous genes has been reported in patients with severe Hunter’s syndrome. 62 Histologically, the retinal findings are indistinguishable from those of retinitis pigmentosa, with loss of photoreceptors and thinning of inner nuclear, outer nuclear, and ganglion cell layers. The RPE demonstrates pigmentary loss and migration. Fibrillogranular and multimembranous vacuoles are found in all ocular tissues examined by electron microscopy. 54
Management and Course
No specific treatment has been proven effective in Hunter’s syndrome.
22.4.3 Mucopolysaccharidosis Type III (MPS III, Sanfilippo’s Syndrome)
MPS III is the most common mucopolysaccharidosis and is estimated to have a frequency of 1 in 24,000 in the Netherlands. Although it has less phenotypic variation than other mucopolysaccharidoses, it is biochemically heterogeneous. Defects in any one of four different enzymes may cause clinically similar disease. 41
Clinical Features
The onset occurs between the ages of 2 and 6 years; affected children present with behavioral disorders and mental regression. The neurologic deterioration is profound and associated with progressive loss of developmental milestones, gait disturbances, deafness, and occasionally seizures. By contrast, the somatic stigmata of coarse facies, moderately short stature, hepatosplenomegaly, stiff joints, and hirsutism are less severe. Some mildly affected patients may even have normal features as adults. Death commonly occurs before the age of 20 years.
Corneal clouding is absent, but retinal degeneration closely resembling that of retinitis pigmentosa, with nyctalopia, bone spicules, vascular attenuation, and optic atrophy, is characteristic. 50 The ERG typically becomes extinct. 51
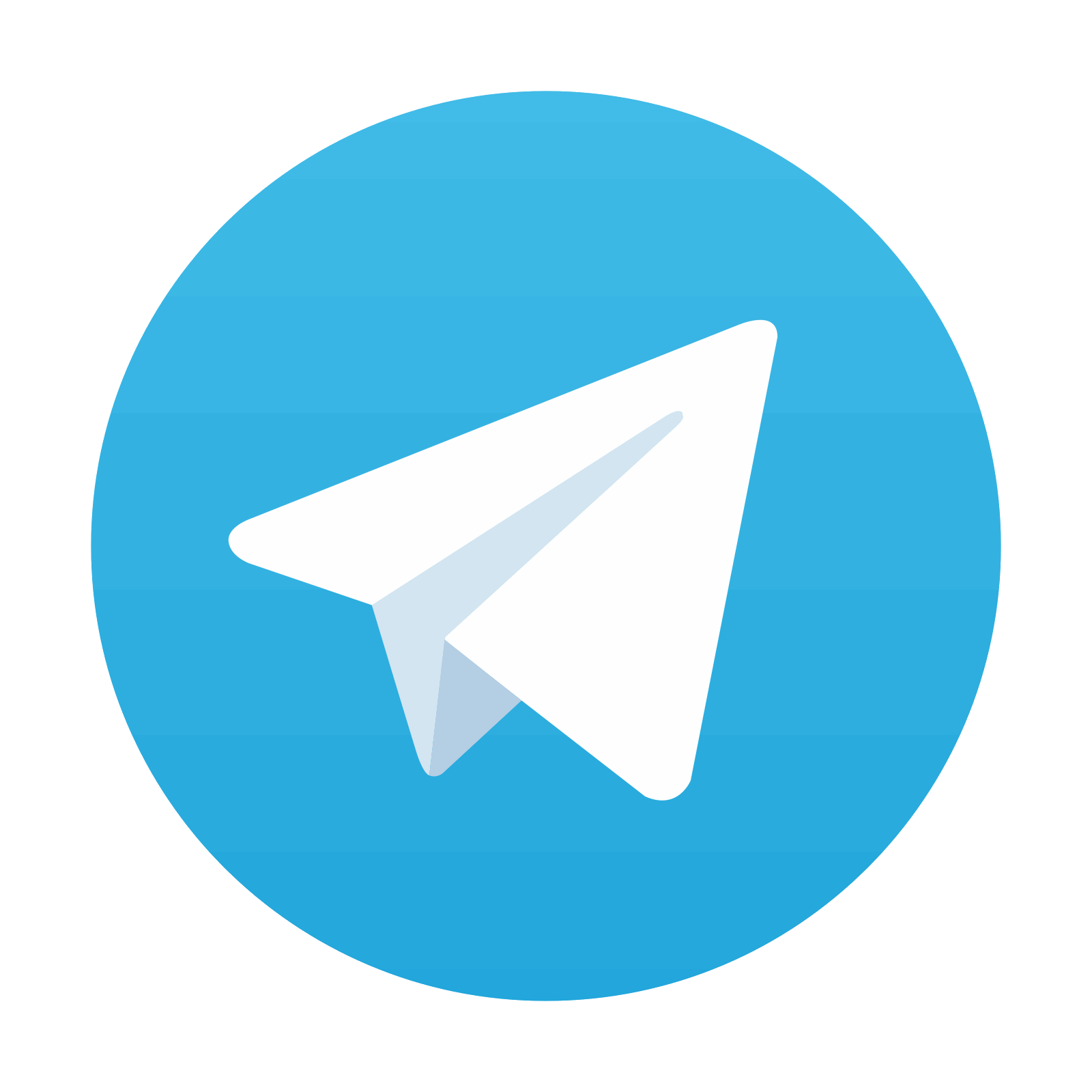
Stay updated, free articles. Join our Telegram channel

Full access? Get Clinical Tree
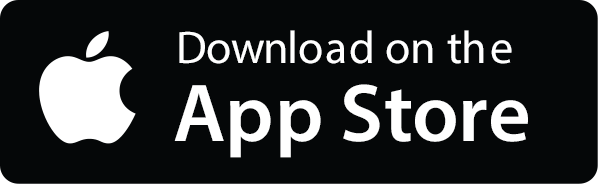
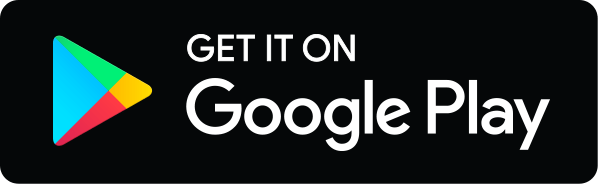
