Vitreous: from Biochemistry to Clinical Relevance
J. Sebag
Kenneth M. P. Yee
Although vitreous is the largest structure within the eye, comprising 80% of its volume, our knowledge of vitreous structure and function is perhaps the least of all ocular tissues. Historically, investigations of vitreous structure have been hampered by two fundamental difficulties: first, any attempts to define vitreous morphology are attempts to visualize a tissue that is invisible by design (Fig. 1).1 Considerable barriers must be overcome to adequately study the structure of an invisible tissue. Second, the various techniques that were used previously to define vitreous structure were fraught with artifacts that biased the results of these investigations. Thus, as noted by Baurmann2 and Redslob,3 histologic studies performed during the nineteenth and early twentieth centuries were flawed by the use of tissue fixatives that caused the precipitation of what we recognize today as the glycosaminoglycan (GAG) hyaluronan (HA; formerly called hyaluronic acid).
The development of slit-lamp biomicroscopy by Gullstrand4 in 1912 generated a great deal of excitement because it was anticipated that this technique would enable investigations of vitreous structure without the introduction of artifacts. Yet as noted by Redslob,3 a varied set of descriptions resulted, mostly because of the first of the previously mentioned inherent difficulties (i.e., vitreous is largely invisible). This problem even persists in more recent investigations. Eisner5 has described membranelles; Worst,6 cisterns; Sebag and Balazs,7,8 fibers; and Kishi and Shimizu,9 pockets in the vitreous body. It is likely that the membranelles described by Eisner are composed of the collagen fibers described by Sebag and Balazs. The observation of so-called pockets by Kishi and Shimizu has been explained as the result of age-related liquefaction of vitreous gel, with little relevance to the normal macromolecular structure.10,11
A clear concept of vitreous structure can only derive from an accurate understanding of its macromolecular organization. The following describes the molecular constituents of vitreous and the macromolecular organization that results in the exquisite anatomic structure of vitreous. Aging and disease-related changes are described as they pertain to the pathophysiology of the major vitreoretinal disorders12,13 as well as the rationales of surgical intervention14 and preventative15,16 modalities.
VITREOUS BIOCHEMISTRY
MOLECULAR CONSTITUENTS
Collagens
Collagen is an important structural protein in vitreous, as in connective tissues everywhere. Gloor17 pointed out that the collagen content is highest where the vitreous is a gel. Gross18 was the first to claim that vitreous collagen fibrils are morphologically distinct from collagen in other connective tissues. Yet, Swann and co-workers19 demonstrated that the amino acid composition of the insoluble residue of vitreous is similar to that of cartilage collagen and later identified that it is most similar to cartilage collagen composed of α1 type II chains.20 Comparisons of the arthritogenic and immunologic properties of collagens from bovine articular cartilage (type II) and vitreous showed that the two were indistinguishable by these assays.21 However, subsequent studies22 demonstrated that while vitreous collagen contains an α1 type II chain similar to cartilage collagen, there is a lower alanine content. Furthermore, these studies found that vitreous collagen has additional peptides present as uncleaved extension chains containing an amino acid composition different from the alpha chain component. The investigators concluded, however, that the overall similarities in amino acid composition and in the types of cyanogen bromide cleavage peptides indicate that the fibers of the central and posterior peripheral regions of the vitreous are composed of a collagen that should be classified as type II. Schmut and associates23 used differential salt precipitation of pepsin-solubilized collagen from bovine vitreous and found that type II collagen is the major component of native vitreous fibers. Linsenmayer and collaborators24 measured in vivo synthesis of types I and II collagen in chick embryo vitreous by radioimmunoprecipitation after tritiated proline labeling and found that over 90% of the labeled material in the vitreous was type II collagen. Snowden25 provided further physicochemical evidence in support of the similarities between vitreous and cartilage collagens. Considered as a whole, this body of work may explain why certain clinical phenomena, such as inborn errors of type II collagen metabolism in arthro-ophthalmopathies, manifest phenotypic expression in each of these two tissues.
There are, however, distinct differences in the chemical composition of vitreous and cartilage collagens that are only partly due to the presence of terminal peptide constituents in vitreous collagen. Swann and Sotman26 have demonstrated that the carbohydrate content of pepsin-solubilized vitreous α chains is significantly greater than cartilage α chains, indicating that the carbohydrate side chains of vitreous collagen are largely composed of disaccharide units similar to those found in basement membrane collagen. They proposed that these distinct chemical features are related to the special structure of the mature vitreous fibrils in vivo. Liang and Chakrabarti27 have shown that there are differences between bovine cartilage and vitreous with respect to collagen fibril growth, melting temperature, and fluorescence with a hydrophobic fluorescent probe. These investigators and others28 proposed that vitreous collagen should be considered a special type II collagen. Ayad and Weiss29 demonstrated that type II is the major vitreous collagen in bovine vitreous but collagens composed of α1, α2, and α3 chains as well as C-PS disulfide-bonded collagen were present in concentrations similar to those in cartilage. In contrast to cartilage, however, vitreous type II collagen was significantly more hydroxylated in the lysine and proline residues. The α1, α2, and α3 collagen chains were interpreted by Van der Rest30 to represent type IX collagen. Eyre and colleagues31 felt that there was evidence to indicate the presence of type V collagen in vitreous. Furthermore, with respect to the disulfide-bonded collagen, vitreous had three times more C-PS1 and C-PS2 collagens than cartilage although the molar ratio of C-PS1 to C-PS2 in both was 1:1, suggesting that in both tissues these collagens are components of a larger molecule, possibly a derivative of type IX collagen. In this regard vitreous is once again similar to cartilage, insofar as both contain type IX collagen, although the two tissues differ in the sizes of type IX collagen chains.32 Hong and Davison33 have identified a procollagen in the soluble fraction of rabbit vitreous that was identified as type II by segment-long spacing-banding patterns. Detection of a propeptide extension only at the N-terminus prompted these investigators to conclude that this was a novel type II procollagen. Such distinctive characteristics are possibly related to the unique physiologic roles of vitreous, in particular, its mechanical function.12
Vitreous collagen fibrils are organized as a triple helix of three alpha chains (Fig. 2). The collagen fibrils of vitreous are heterotypic, consisting of more than one collagen type. Recent studies of pepsinized forms of collagen confirm that vitreous contains collagen type II, a hybrid of types V/XI, and type IX34,35
![]() Fig. 2. Schematic diagram of vitreous collagen fibril organization (d’après Bishop62). The central core of vitreous collagen fibrils is composed of a hybrid of collagen types V and XI molecule. Surrounding the core is a specialized form of type II collagen, which constitutes 75% of vitreous collagen. N-propeptide extensions of these two components are found on the surface of vitreous collagen fibrils and may mediate interactions with other extracellular components of vitreous. However, the presence of type IX collagen on the surface of vitreous collagen fibrils probably plays a significant role in this regard. |
TYPE II COLLAGEN.
Type II collagen, a homotrimer composed of three identical α chains designated as [α1 (II)]3, comprises 75% of the total collagen content in vitreous. When first synthesized as a procollagen and secreted into the extracellular space, type II collagen is highly soluble. The activity of N-proteinase and C-proteinase enzymes reduces the solubility and enables type II collagen molecules to cross-link covalently in a quarter-staggered array. Within this array are likely to be N-propeptides, which probably extend outward from the surface of the forming fibril.35 This may influence the interaction of the collagen fibril with other components of the extracellular matrix. Recent studies36 combined immunolocalization with Western blot analysis of macromolecules extracted from bovine vitreous collagen fibrils and found that the pN-type IIA procollagen is located on the surface of the vitreous collagen fibril. The finding37 that type IIA procollagen propeptides specifically bind transforming growth factor (TGF)-β1 and bone morphogenic protein-2 (BMP-2) supports the concept that in certain circumstances, such growth factors and cytokines interact with vitreous fibrils to promote the cell migration and proliferation that result in proliferative diabetic retinopathy and proliferative vitreoretinopathy.
TYPE IX COLLAGEN.
Type IX collagen is a heterotrimer which is disulfide-bonded with an [α 1 (IX) α 2 (IX) α 3 (IX)] configuration. This heterotrimer is orientated regularly along the surfaces of the major collagen fibrils in a D periodic distribution, where it is cross-linked onto the fibril surface. Type IX is not a typical collagen but is a member of the fibrillar-associated collagens with interrupted triple helixes (FACIT) group of collagens. It contains collagenous regions described as COL1, COL2, and COL3 interspersed between noncollagenous regions called NC1, NC2, NC3, and NC4.35,38 In vitreous, as opposed to cartilage, the NC4 domain is small and, therefore, not highly charged and not likely to exhibit extensive interaction with other extracellular matrix components.39 In vitreous, type IX collagen always contains a chondroitin sulfate glycosaminoglycan chain,35,38 which is linked covalently to the α 2 (IX) chain at the NC3 domain; this enables the molecule to assume a proteoglycan form. Electron microscopy of vitreous stained with cationic dyes enables visualization of the chondroitin sulfate chains of type IX collagen. In some of these studies, sulfated glycosaminoglycans are found distributed regularly along the surface of vitreous collagen fibrils,40 and often bridge between neighboring collagen fibrils. Duplexing of glycosaminoglycan chains from adjacent collagen fibrils may result in a ladderlike configuration.41 However, this hypothesis of vitreous organization has yet to be confirmed.
TYPE V/XI COLLAGEN.
Ten percent of vitreous collagen is a hybrid V/XI collagen, which is believed to comprise the central core of the major collagen fibrils of vitreous.42 Type V/XI is a heterotrimer that contains α 1 (XI) and α 2 (V) in two chains, while the nature of the third chain is presently not known. Along with type II collagen, type V/XI is a fibril-forming collagen. While the interaction of the fibril with other extracellular matrix components is probably influenced by a retained N-propeptide which protrudes from the surface of the fibril in cartilage,42 it is not known whether this is the case in vitreous.
TYPE VI COLLAGEN.
Although there are only small amounts of type VI collagen in vitreous, the ability of this molecule to bind both type II collagen and HA suggests that it could be important in organizing and maintaining the supramolecular structure of vitreous gel. Aggregates of type VI collagen have been found in the cortical vitreous of a patient with macular hole,43 but it is not clear whether this plays any role at all in the pathogenesis of this condition.
GLYCOSAMINOGLYCANS
GAGs are polysaccharides of repeating disaccharide units, each consisting of hexosamine (usually N-acetyl glucosamine or N-acetyl galactosamine) glycosidically-linked to either uronic (glucuronic or iduronic) acid or galactose. The nature of the predominant repeating unit is characteristic for each glycosaminoglycan and the relative amount, molecular size, and type of glycosaminoglycan are said to be tissue-specific.44 GAGs do not normally occur as free polymers in vivo but are covalently linked to a protein core, the ensemble called a proteoglycan. A sulfated group is attached to oxygen or nitrogen in all GAGs except HA. Balazs45 first documented the presence of sulfated galactosamine-containing GAGs in bovine vitreous (less than 5% of total vitreous glycosaminoglycans), and others46,47 identified these as chondroitin-4-sulfate and undersulfated heparan sulfate. Studies in the rabbit48 found a total vitreous glycosaminoglycans content of 58 ng with 13% chondroitin sulfate and 0.5% heparan sulfate.
HYALURONAN.
Although HA is present throughout the body, it was first isolated from bovine vitreous by Meyer and Palmer in 1934.49 In humans, HA first appears after birth and then becomes the major vitreous GAG. It has been proposed that hyalocytes synthesize HA, but other plausible candidates are the ciliary body and retinal Müller cells. Whereas the synthesis of HA seems to continue at a constant rate in the adult without extracellular degradation, HA levels are in a steady-state because the molecule escapes via the anterior segment of the eye.45 Laurent and Fraser50 showed that the passage of HA from the vitreous to the anterior segment is strongly molecular-weight–dependent, indicating a diffusion-controlled process. In contrast, disappearance of HA from the anterior chamber is independent of molecular weight, suggesting that this is controlled by bulk flow.
HA is a long, unbranched polymer of repeating glucuronic acid β-1,3-N,N-acetylglucosamine disaccharide moieties linked by β-1–4 bonds. It is a linear, left-handed, threefold helix51 with a rise per disaccharide on the helix axis of 0.98 nm (Fig. 3).52 Rotary shadowing electron microscopy of human and bovine vitreous detected lateral aggregates of HA that form an anastomosing three-dimensional network.52 This periodicity, however, can vary depending on whether the helix is in a compressed or extended configuration.53 Changes in the degree of extension of HA could be important in the role vitreous plays in retinal disease. Indeed, the volume of the unhydrated HA molecule is approximately 0.66 cm3/g, whereas the hydrated specific volume is 2,000 to 3,000 cm3/g.53 Thus, the degree of hydration has a significant influence on the size and configuration of the HA molecular network. Although there is no definitive evidence that adjacent HA chains bind to one another, Brewton and Mayne54 first proposed such an arrangement. Recent rotary shadowing electron microscopy studies55 of bovine and human vitreous found lateral aggregates of HA that formed three-dimensional latticelike networks. HA also interacts with the surrounding mobile ions and can undergo changes in its conformation that are induced by changes in the surrounding ionic milieu.56 A decrease in surrounding ionic strength can cause the anionic charges on the polysaccharide backbone to repel one another, resulting in an extended configuration of the macromolecule. An increase can cause contraction of the molecule and, in turn, the entire vitreous body. As a result of HA’s entanglement and immobilization within the vitreous collagen fibril matrix, this mechanical force can be transmitted by collagen fibrils to the retina, optic disc, and other structures, such as neovascular complexes. In this way, changes in the ionic milieu of vitreous may be converted into mechanical energy via extension or contraction of the HA macromolecule. This can be important in certain pathologic conditions that feature fluctuations in ionic balance and hydration, such as diabetes.57
The sodium salt of HA has a molecular weight of 3 to 4.5 × 106 in normal human vitreous.45 Laurent and Granath58 used gel chromatography and found the average molecular weight of rabbit vitreous to be 2 to 3 × 106 and of bovine vitreous to be 0.5 to 0.8 × 106. In these studies there were age-related differences in the bovine vitreous, in which HA molecular weight varied from 3 × 106 in the newborn calf to 0.5 × 106 in old cattle. Furthermore, there may be several species of HA within vitreous that have polysaccharide chains of different lengths59 with a variable distribution in different topographic regions within the vitreous body.60
An important property of HA is that of steric exclusion.61 With its flexible linear chains and random coil conformation, HA occupies a large volume and resists the penetration of this volume by other molecules to a degree dependent upon their size and shape.53 This excluded volume effect can influence equilibria between different conformational states of macromolecules and alter the compactness or extension of these molecules. Steric exclusion also causes an excess of osmotic pressure when such compounds as albumin and HA are mixed, because the resultant osmotic pressure is greater than the sum of the two components. This could be important in diabetes, in which vascular incompetence can increase vitreous concentrations of serum proteins such as albumin. These osmotic effects can induce contraction and expansion of the vitreous body similar to the foregoing description of hydration and ion-induced changes within vitreous, and can similarly play an important role in neovascularization and diabetic vitreous hemorrhage.57 An increase in the chemical activity of a compound because of steric exclusion can cause its precipitation if the solubility limit is reached. This could be important in the formation of pathologic vitreous opacities, such as asteroid hyalosis and amyloidosis (see below).
CHONDROITIN SULFATE.
Vitreous contains two types of chondroitin sulfate proteoglycans. The minor type is actually type IX collagen, which is described above. The majority of vitreous chondroitin sulfate, however, is in the form of versican.55 This large proteoglycans (molecular weight [MW] = 380 kd) has a globular N-terminal which binds HA via a 45-kd link protein. Thus, in human but not bovine vitreous, versican is believed to form complexes with HA as well as microfibrillar proteins, such as fibulin-1 and fibullin-2.62 The concentration of versican in the human vitreous is 0.06 mg/mL of vitreous gel, representing approximately 5% of the total protein content. Each versican molecule has 5 to 7 chondroitin sulfate side chains (MW = 37 kd each), which form aggregates with vitreous HA. It also binds lectins via the C-terminal region. Binding by both the C- and N-terminal domains is believed to be the manner by which versican stabilizes the macromolecular structures and organization of vitreous.63
HEPARAN SULFATE.
This sulfated proteoglycans is normally found in basement membranes and on cell surfaces throughout the body. It was first detected in bovine vitreous in 1977,64 and in chick vitreous (as agrin) in 1995.65 However, it is not clear whether heparan sulfate is a true component of vitreous or a contaminant from adjacent basement membranes, such as the internal limiting lamina of the retina.64 As pointed out by Bishop62 this may also be the case for nodogen-1, the aforementioned fibulins, and fibronectin.
Noncollagenous Structural Proteins
FIBRILLINS.
Fibrillin-containing microfibrils are more abundant in vitreous than the type VI collagen microfibrils described above. They are the found in vitreous gel as well as in the zonules of the lens. This fact explains why in Marfan’s syndrome the defects in the gene encoding fibrillin-1 (FBN1 on chromosome 15q21) result in both ectopia lentis and vitreous liquefaction.62 The latter probably plays a role in the frequent occurrence of rhegmatogenous retinal detachment in these patients.
OPTICIN.
The major noncollagenous protein of vitreous is a leucine-rich repeat (LRR) protein, which is bound to the surface of the heterotypic collagen fibrils, known as opticin.67,68,69 Formerly called vitrican, opticin is believed to be important in collagen fibril assembly and in preventing the aggregation of adjacent collagen fibrils into bundles. Thus, a breakdown in this property or activity may play a role in age-related vitreous degeneration (see below).70
VIT1.
Another novel vitreous protein is VIT1, a collagen-binding macromolecule.71 Because of its propensity to bind collagen, this highly basic protein may play an important role in maintaining vitreous gel structure.
Glycoproteins
Glycoproteins are macromolecules of the ground substance existing as heteropolysaccharides rather than homogeneous repeating disaccharide units, such as found in GAGs. They are mostly proteinaceous and contain only a minor carbohydrate component (5% to 10% by weight). According to Balazs,72 the most important difference between vitreous and serum proteins is the high content of glycoproteins in vitreous, because these constitute 20% of the total noncollagenous protein content of vitreous.
There are two types of vitreous glycoproteins: those found associated with collagen fibrils and those that freely diffuse in the interfibrillar space. Although some of the soluble glycoproteins enter vitreous from serum, many are believed to be specific to vitreous. Studies using the radiolabeled precursor3 H-fucose detected 14 fractions of labeled polypeptides.73 The same results were obtained using tritiated amino acids,74 suggesting that more than one component is synthesized within the eye, probably at the ciliary body. Rhodes and co-workers75 studied the incorporation of tritiated-fucose into rabbit vitreous and surrounding tissues, concluding that there is continuous renewal of the glycoproteins in and around the vitreous body. They suggested that this process could serve as an index of metabolic activity in normal and pathologic states. Hageman and Johnson76 documented the distribution of vitreous glycoproteins in several species, including man, and characterized these glycoproteins based on differential staining to various fluorescein isothiocyanate-conjugated lectins.
There is no clear understanding of the role of glycoproteins in vitreous. Through Western blotting techniques, an acidic glycoprotein has been identified that is unique to cartilage and vitreous.77 It is known as cartilage oligomeric matrix protein and has a characteristic five-armed structure. The function of this molecule in vitreous is unknown. Nevertheless, it represents more evidence linking vitreous with joints and may explain why both structures are often simultaneously involved in certain syndromes. Recent findings, reviewed by Bishop et al,78 have led to the concept that glycoproteins and other connective tissue macromolecules, as well as basement membrane macromolecules that are synthesized by the ciliary body are important in the biosynthesis of vitreous as well as the internal limiting lamina of the retina. The former has significant implications for development of the eye as well.
Miscellaneous Components
ANTIOXIDANTS.
High performance liquid chromatography measurements of vitreous ascorbic acid concentration showed values approximating 0.43 mmol/kg, which represent a vitreous to plasma ratio of 9:1.79 Vitreous levels this much higher than plasma concentrations are believed to be caused by active transport by the ciliary body epithelium. Such high vitreous concentrations of ascorbic acid may relate to the ability of this compound to absorb ultraviolet light80 and serve as a free-radical scavenger.81 This would protect the retina and lens from the untoward effects of metabolic and light-induced singlet oxygen generation. Indeed, a study in an animal model showed that the light-induced free radicals induced vitreous liquefaction.82 Ascorbic acid may also protect against oxidative damage due to inflammation.83 Vitreous levels of serum-derived ceruloplasmin and transferrin also increase after experimental inflammation,84 presumably to provide additional antioxidant protection.
SUPRAMOLECULAR ORGANIZATION
As described by Mayne,85 vitreous is organized as a dilute meshwork of collagen fibrils interspersed with extensive arrays of long HA molecules. The collagen fibrils provide a scaffold-like structure that is inflated by the hydrophilic HA. If collagen is removed, the remaining HA forms a viscous solution; if HA is removed, the gel shrinks56 but is not destroyed. Early physiologic observations86 suggested the existence of an interaction between HA and collagen which stabilizes collagen. Gelman and associates87 have shown that several GAGs, including HA, stabilized the helical structure of collagen, so that the melting temperature of collagen was increased from 38° C to 46°C. Hyaluronidase decreased the thermal stability of cartilage collagen and the addition of chondroitin-6-sulfate to hyaluronidase-treated collagen in turn increased the thermal stability. Snowden88 demonstrated that the shrinkage temperature of tendon collagen is dependent linearly on the concentration of chondroitin sulfate in the surrounding fluid. Biomechanical studies89 of vitreous viscoelasticity suggested that collagen similarly affects HA. The exact nature of the interaction between vitreous macromolecules that results in the aforementioned phenomena has yet to be determined, although there are several possibilities: interactions on a chemical or electrostatic level, or via an intermediary molecule. Concerning a chemical interaction, Balazs45 hypothesized that the hydroxylysine amino acids of collagen mediate polysaccharide binding to the collagen chain via O-glycosidic linkages. These polar amino acids are present in clusters along the collagen molecule, which may explain why proteoglycans attach to collagen in a periodic pattern.40
Mathews90 observed the reversible formation of complexes of an electrostatic nature between solubilized collagen and various GAG. He suggested that collagen-HA interaction occurs on a physicochemical rather than chemical level. Podrazky and co-workers91 demonstrated that the sulfate group of a GAG was largely responsible for interactions with the guanidine groups of arginine and ε-amino groups of lysine in collagen. When these highly charged groups on the collagen fibrils are bound by advanced glycation end products, such as occurs in diabetes57 and aging,92 destruction of normal vitreous structure results, leading ultimately to diabetic,93 age-related,94,95 structural changes. Others91 demonstrated that the sulfate group of a GAG was largely responsible for such interactions with the guanidino groups of arginine and epsilon-amino groups of lysine in collagen. Comper and Laurent56 proposed that in vitreous, electrostatic binding occurs between negatively charged polysaccharides and positively charged proteins. These authors extensively reviewed the existing data characterizing the electrostatic properties of glycosaminoglycans and the factors influencing their electrostatic interactions with different ions and molecules.
HA-collagen interaction in vitreous may be mediated by a third molecule.96 Studies by Hong and Davison97 have identified a type II procollagen in the soluble fraction of rabbit vitreous and raised the question of a possible role for this molecule in mediating collagen-HA interaction. Measurements of the dynamic viscoelasticity of bovine vitreous showed that the shapes of the master relaxation curves of the vitreous body are similar to those of lightly cross-linked polymer systems.98 Notably, the behavior of these relaxation curves is different from those observed in solutions of HA and collagen. This suggests that the physicochemical properties of vitreous in vivo are not simply the result of a combination of these two molecular elements, but that HA and collagen form a lightly cross-linked polymer system. Swann and colleagues99 have demonstrated large amounts of noncollagenous protein associated with collagen in the insoluble residue fraction of vitreous. Asakura40 studied bovine vitreous by ruthenium red staining and demonstrated the presence of amorphous structures on collagen fibrils at 55- to 60-nm intervals along the fibrils that are believed to be HA (Fig. 4). Filaments connect the collagen fibrils and these amorphous masses. These filaments may represent link structures that are either proteoglycans or a glycoprotein, such as hyaluronectin.100 In cartilage, link glycoproteins have been identified that interact with proteoglycans.101 Supramolecular complexes of these glycoproteins are thought to occupy the interfibrillar spaces in cartilage and may have a similar role in vitreous. In the cornea, chondroitin sulfate, and keratan sulfate bridge the interfibrillar spaces and keep the fibrils at specified distances to achieve transparency.102 This is clearly important in vitreous as well, where HA could bind to collagen fibrils by such linkage molecules, most probably in a repeating order. This type of arrangement would bind collagen fibrils to the protein core of a proteoglycans, such as chondroitin sulfate, as described by Asakura,40 keratan sulfate, or both, as in the cornea, and would organize the network in a manner to keep the vitreous collagen fibrils apart by at least one wavelength of incident light, the critical distance needed to minimize light scattering. Although such supramolecular organization to maintain vitreous transparency was proposed41 more than a decade ago, no evidence has yet been generated.
Bishop62 has proposed that to understand how vitreous gel is organized and stabilized requires an understanding of what prevents collagen fibrils from aggregating and by what means the collagen fibrils are connected to maintain a stable gel structure. Scott and colleagues103 have proposed that the chondroitin sulfate chains of type IX collagen bridge between adjacent collagen fibrils in a ladderlike configuration spacing them apart. This arrangement might account for vitreous transparency, because keeping vitreous collagen fibrils separated by at least one wavelength of incident light would minimize light scattering, allowing unhindered transmission of light to the retina for photoreception. However, depolymerizing with chondroitinases does not destroy the gel, suggesting that chondroitin sulfate side chains are not essential for vitreous collagen spacing. Complexed with HA, however, the chondroitin sulfate side chains might space apart the collagen fibrils sufficiently.41,103 Yet, Bishop believes that this form of collagen-HA interaction would only be very weak. Instead, he proposes that the leucine-rich repeat (LRR) protein opticin is the predominant structural protein in short-range spacing of collagen fibrils. Concerning long-range spacing, Scott41 and Mayne et al104 have claimed that HA plays a pivotal role in stabilizing the vitreous gel via this mechanism. However, studies105 using HA lyase to digest vitreous HA demonstrated that the gel structure was not destroyed, suggesting that HA is not essential for the maintenance of vitreous gel stability and leading to the proposal that collagen alone is responsible for the gel state of vitreous.
Several types of collagen-HA interactions may occur in different circumstances. Further investigation must be undertaken to identify the nature of collagen-HA interaction in vitreous. This question is important for an understanding of normal vitreous anatomy and physiology but also as a means by which to understand the biochemical basis for age- and disease-related vitreous liquefaction and posterior vitreous detachment (PVD).
SPECIES VARIATIONS
Vitreous of all species is composed of essentially the same extracellular matrix elements, organized to fill the center of the eye with a clear viscous substance surrounded by a dense cortex that is attached to the basal laminae of surrounding cells. There are, however, species variations in the relative concentrations of the major structural components (i.e., HA and collagen). These differences explain variations in the rheologic (gel–liquid) state of vitreous in different species. It should be emphasized that there are also age-related differences in higher order species. Consequently, when selecting an appropriate animal with which to model human diseases for scientific investigation, consideration must be given to these species variations and age-related differences.
Significant species differences exist for HA, which is present in vitreous of all species studied except fish. In fish, the posterior and peripheral vitreous adjacent to the retina is a gel. The anterior and central vitreous is liquid. Because there is no anterior vitreous cortex, this liquid vitreous is continuous with the posterior and anterior chambers, thereby surrounding the lens. In place of HA, the fish vitreous contains icthyosan, a large (MW = 2 to 4 × 106) nonsulfated polysaccharide consisting of HA and a chondroitin chain held together by noncolvent bonds.106 HA concentration in human vitreous (approximately 192 μg/mL) is about the same as in the rhesus monkey, which is less than in the owl monkey (291.8 ± 18.8 μg/mL) and bovine (469 ± 44.0 μg/mL) vitreous.107,108 The molecular weight of rhesus monkey HA is 2.9 ± 0.06 × 106,108 which is significantly less than in humans (4.6 × 106; p > 0.001).107 In pigs, both collagen and HA are found in half the concentration as in humans.
Collagen concentration in vitreous gel is estimated to be approximately 60 μg/mL in bovine eyes and approximately 300 μg/mL in human eyes.109 Snowden and Swann110 demonstrated that collagen fibrils in rabbit vitreous measure 7 nm in diameter, whereas bovine and canine vitreous collagen fibrils are between 10 and 13 nm in diameter. Human vitreous collagen fibrils are 10 to 25 nm in diameter.111 Although the baby owl monkey vitreous is a gel with a network of collagen-like fibrils that have a diameter of 11.3 nm, the adult owl monkey has no collagen and vitreous is a viscous fluid that contains mostly high molecular weight HA.112
VITREOUS ANATOMY
EMBRYOLOGY
Interfaces
During invagination of the optic vesicle, the basal lamina of the surface ectoderm enters the invagination along with the ectodermal cells that have become specialized neural ectoderm.113 The ectodermal cells that are on the surface of the inner with the outer basal lamina give rise to retinal pigment epithelium attached to Bruch’s membrane, while the invaginating neural ectoderm and its basal lamina give rise to the neural retinal cells adherent to the internal limiting lamina (ILL). Thus, the basal laminae of both the retina and RPE have the same embryologic origin. Figure 5 demonstrates the continuity of these two basal laminae. It is important to appreciate that these basal laminae serve as interfaces between adjacent ocular structures. In the case of the ILL, this basal lamina is the interface between the retina and vitreous. Bruch’s membrane separates the RPE and retina from the choroid (neural crest origin).
These interfaces play an important role in a significant biologic event that underlies one of the most devastating causes of blindness in humans: neovascularization. At the ILL interface between vitreous and retina, neovascularization in advanced diabetic retinopathy and other ischemic retinopathies, including retinopathy of prematurity (see below), is a significant cause of vision loss. At the level of Bruch’s membrane, an interface of identical embryologic origin as the ILL, neovascularization in age-related macular degeneration is a significant and growing problem. Both of these conditions result from vascular endothelial cell migration and proliferation onto and into interfaces of the same embryologic origin: the basal lamina of the surface ectoderm. Improving our understanding of endothelial cell interaction with these interfaces should provide new insights into therapy and prevention of these important disorders.
Vitreous Body
Early in embryogenesis, the vitreous body is filled with blood vessels known as the vasa hyaloidea propia. This network of vessels arises from the hyaloid artery, which is directly connected to the central retinal artery at the optic disc. The vessels branch many times within the vitreous body and anastomose anteriorly with a network of vessels surrounding the lens, the tunica vasculosa lentis. This embryonic vascular system attains its maximum prominence during the ninth week of gestation or 40-mm stage.114 Atrophy of the vessels begins posteriorly with dropout of the vasa hyaloidea propria, followed by the tunica vasculosa lentis. At the 240-mm stage (seventh month) in the human, blood flow in the hyaloid artery ceases.115 Regression of the vessel itself begins with glycogen and lipid deposition in the endothelial cells and pericytes of the hyaloid vessels.115 Endothelial cell processes then fill the lumen and macrophages form a plug that occludes the vessel. The cells in the vessel wall then undergo necrosis and are phagocytized by mononuclear phagocytes.116 Gloor117 claims that macrophages are not involved in vessel regression within the embryonic vitreous but that autolytic vacuoles form in the cells of the vessel walls, perhaps in response to hyperoxia. Interestingly, the sequence of cell disappearance from the primary vitreous begins with endothelial and smooth muscle cells of the vessel walls, followed by adventitial fibroblasts and lastly phagocytes,118 consistent with a gradient of decreasing oxygen tension.
It is not known precisely what stimulates regression of the hyaloid vascular system, but studies have identified a protein native to the vitreous that inhibits angiogenesis in various experimental models.119,120,121 Teleologically, such activity seems necessary if a transparent tissue is to inhibit cell migration and proliferation and minimize light scattering to maintain transparency. This may also be the mechanism that induces regression of the vasa hyaloidea propia. Thus, activation of this protein and its effect on the primary vitreous may be responsible for the regression of the embryonic hyaloid vascular system as well as the inhibition of pathologic neovascularization in the adult. Hyaloid vessel regression may also result from a shift in the balance between growth factors promoting new vessels, such as vascular endothelial growth factor (VEGF)-A, and those inducing regression, such as placental growth factor.122
Recent studies123,124 have suggested that vasa hyaoidea propria and the tunica vasculosa lentis regress via apoptosis. Mitchell and colleagues123 pointed out that the first event in hyaloid vasculature regression is endothelial cell apoptosis and propose that lens development separates the fetal vasculature from VEGF-producing cells, decreasing the levels of this survival factor for vascular endothelium, inducing apoptosis. After endothelial cell apoptosis, there is loss of capillary integrity, leakage of erythrocytes into the vitreous, and phagocytosis of apoptotic endothelium by macrophages, which were felt to be important in this process. Subsequent studies by a different group125 confirmed the importance of macrophages in promoting regression of the fetal vitreous vasculature and further characterized these macrophages as hyalocytes. Meeson and colleagues126 proposed that there are actually two forms of apoptosis that are important in regression of the fetal vitreous vasculature. The first (initiating apoptosis) results from macrophage induction of apoptosis in a single endothelial cell of an otherwise healthy capillary segment with normal blood flow. The isolated dying endothelial cells project into the capillary lumen and interfere with blood flow. This stimulates synchronous apoptosis of downstream endothelial cells (secondary apoptosis) and ultimately obliteration of the vasculature. Removal of the apoptotic vessels is achieved by hyalocytes.
A better understanding of this phenomenon may provide insights into new ways to induce the regression of pathologic angiogenesis or inhibit neovascularization in such conditions as proliferative diabetic retinopathy and exudative age-related macular degeneration (AMD). Indeed, the recently developed synthetic VEGF inhibitors seem to be of limited usefulness in treating pathologic neovascularization in exudative AMD and this or a superior inhibitory mechanism may prove to be useful in other proliferative retinopathies, such as retinopathy of prematurity.
Retinopathy of Prematurity
The pathogenesis of retinopathy of prematurity (ROP) begins with birth prior to complete maturation and development of the peripheral retina. Postnatal oxygen administration triggers retinal vasoconstriction with endothelial cell necrosis and vaso-obliteration in response to hyperoxia.127,128,129 After the discontinuation of supplemental oxygen, arterial pO2 levels return to normal and the obliterated (or at best, highly constricted) vessels are not adequately reperfused, causing the peripheral retina they subserve to become ischemic and release neovascular growth factors. An alternative hypothesis of pathogenesis proposes that spindle cells in the immature peripheral retina are stimulated by excessive amounts of reactive oxygen species, whether related to oxygen therapy and subsequent relative hypoxia or other metabolic circumstances, to release angiogenic growth factors.130 In either case, the result is migration and proliferation of capillary endothelial cells that form new blood vessels at the posterior ridge of tissue between the vascularized and avascular retina. This results in neovascularization arising from the ridge that demarcates the developed posterior retina from the immature peripheral retina (Fig. 6). The new vessels grow into the vitreous body, onto and into the plane that demarcates the interface between the gel vitreous posteriorly (overlying developed retina) and the liquid vitreous anteriorly (overlying immature retina). While somewhat similar to neovascularization in diabetic retinopathy,131 the new vessels in ROP grow farther anteriorly and with greater exuberance. This is perhaps because of the participation of cells of the ocular fetal vasculature, whose apoptosis has been retarded or arrested by the presence of high levels of VEGF.132
There are no clearly identified vitreous changes during stages I and II of acute ROP, although this may simply be due to our present inability to detect such abnormalities.133 Indeed, the abundance of reactive oxygen species in the retina and vitreous of premature infants could induce widespread vitreous liquefaction.82 There are also likely to be localized areas of liquid vitreous, particularly at the periphery. At surgery for stage IV-A ROP with retinal detachment, there is a trough in the periphery.134 This structure is most likely the consequence of underlying immature retina in the periphery, with consequent lack of typical gel vitreous synthesis, normally a Müller cell function, overlying the immature retina. The liquid vitreous trough is probably present early in the natural history of disease but goes undetected by present vitreous imaging techniques.133 Such disruption of normal vitreous composition and structure probably alters a number of physiologic processes within the vitreous, including the ability of vitreous to inhibit cellular and vascular invasion.135 Furthermore, the interface between posterior gel vitreous and peripheral liquid vitreous at the ridge causes vitreous traction to be exerted at the retinal ridge.
In stage III ROP, new blood vessels extend from the inner retina into the vitreous cortex. The cortex, overlying the rear guard of differentiated capillary endothelial cells, becomes opaque and contains linear, fibrous structures adjacent to a large pocket of liquid vitreous.136 In advancing from stage III to stage IV, the neovascular tissue arising from the rear guard grows through the vitreous body toward Wiegert’s ligament on the posterior lens capsule.136 This configuration of neovascularization is probably the result of cell migration and proliferation along the walls of the future Cloquet’s canal or the tractus hyaloideus of Eisner. Cells of the primary vitreous likely contribute to the formation of the dense central vitreous stalk and retrolental membrane seen in the cicatricial stage, because these cells could also undergo migratory and proliferative responses to intraocular angiogenic stimuli.
Familial Exudative Vitreoretinopathy
This autosomal (long arm of chromosome 11) dominant (sporadic cases of X-linked recessive disease have been reported) vitreoretinopathy features inner retinal non-perfusion, neovascularization and retinal detachment. The histologic vascular changes are indistinguishable from those of ROP, however, patients with familial exudative vitreoretinopathy (FEVR) have no history of prematurity or neonatal oxygen exposure.137,138 Mild forms of the disease are characterized by areas of peripheral nonperfusion, cystoid degeneration, and condensation of the temporal vitreous base and vitreous membranes. Moderate forms involve vitreous base traction, flat or elevated neovascularization, and retinal or subretinal exudates. The severe forms of FEVR, which usually cause vision loss, are similar to cicatricial ROP, featuring iris atrophy, neovascular glaucoma, cataracts, falciform retinal folds, macular heterotopia, and retinal detachment.139,140 In a recent series141 of 28 patients who underwent vitreoretinal surgery, 25 had rhegmatogenous and 2 had traction retinal detachments. Vitreoretinal adhesion was so strong in the avascular peripheral retina that iatrogenic retinal breaks occurred in 22 of 28 eyes during surgery. It is quite plausible that in similar fashion to ROP, FEVR has poorly developed vitreous overlying the nonperfused, and therefore immature, peripheral retina. The instability between the well-developed posterior gel vitreous and the liquefied peripheral anterior vitreous (Fig. 6) probably exerts traction upon the retina inducing the rhegmatogenous and tractional detachments.
POSTNATAL DEVELOPMENT
Vitreous Size
Vitreous undergoes significant growth during childhood. A study of 926 children found that the average length of the newborn vitreous is 10.5 mm in males and 10.2 mm in females.142 Immediate postnatal development was studied in the rhesus monkey where axial length was found to increase most rapidly during the first 5 to 7 months, increase at a slower pace during the next 6 months, and continue to increase slowly until 4 years of age. This purportedly corresponds closely to axial elongation in humans.143 Fledelius144 used ultrasound to measure ocular dimensions in adolescents and found that in the absence of changes in refractive status, there was a mean vitreous elongation of 0.35 mm from age 10 to 18 years. By the age of 13 years, the axial length of the human vitreous body increases to 16.1 mm in the male and 15.6 mm in the female.144 Derangements in ocular growth that result in ametropia are associated with abnormal vitreous dimensions. In children born prematurely (birth weight less than 2000 g), axial lengths of the eye and vitreous at age 10 years are less than normal, a deficiency that is also present at the age of 18 years, suggesting that prematurity does not simply induce a temporary delay of eye growth but a permanent deficit.144
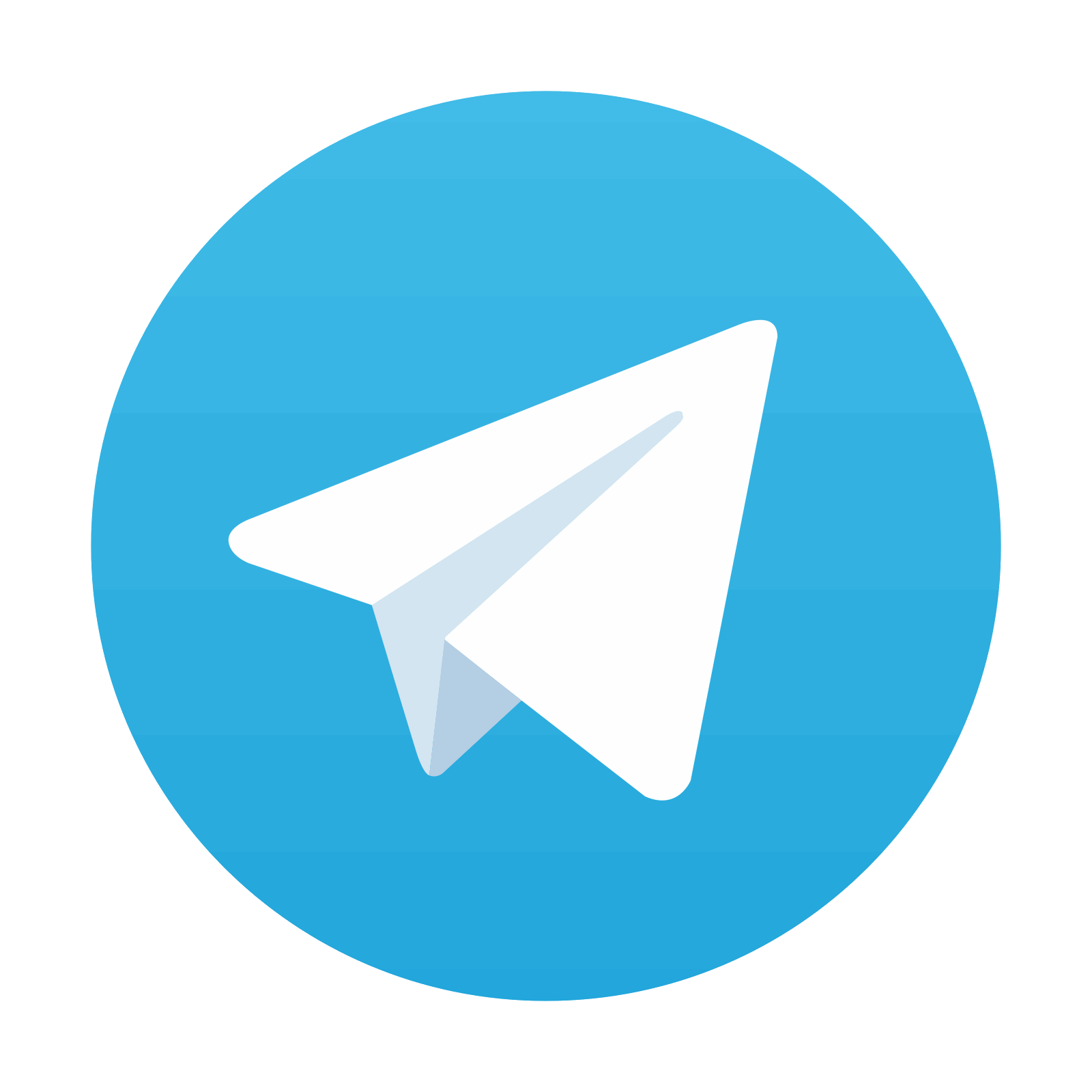
Stay updated, free articles. Join our Telegram channel

Full access? Get Clinical Tree
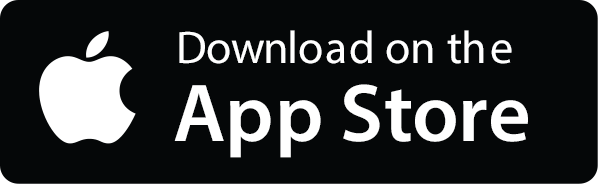
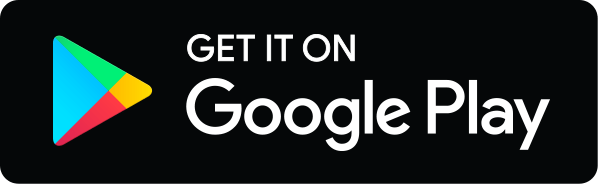
