Vitreous Biochemistry, Structure, and Clinical Evaluation
J. Sebag
BIOCHEMISTRY
COLLAGENS
There are 27 types of collagen molecules identified to date, referred to as types I to XXVII. These collagens are organized into either fibrils or sheetlike structures. In vitreous, nearly all of the collagen is in the form of fibrils that are thin, uniform, and heterotypic (of mixed composition) containing primarily collagen types II, IX, and the hybrid V/XI (Fig. 38-1). Gross1 initially claimed that vitreous collagen fibrils are morphologically distinct from collagen in other connective tissues. However, Swann et al2 demonstrated that the amino acid composition of the insoluble residue of vitreous is similar to that of cartilage collagen and later identified that it is most similar to cartilage collagen composed of alpha 1, type II chains.3 Comparisons of the arthrogenic and immunologic properties of collagens from bovine articular cartilage (type II) and vitreous showed that the two were indistinguishable by these assays.4 Subsequent studies5 demonstrated that, although vitreous collagen contains an alpha 1, type II chain similar to cartilage collagen, there is a lower alanine content. Furthermore, these studies found that vitreous collagen has additional peptides present as uncleaved extension chains containing an amino acid composition different from the alpha chain component. The investigators concluded, however, that the overall similarities in amino acid composition and in the types of cyanogen bromide cleavage peptides indicated that the fibers of the central and posterior peripheral regions of the vitreous are composed of a collagen that should be classified as type II. Linsenmayer et al6 measured in vivo synthesis of types I and II collagen in chick embryo vitreous by radioimmunoprecipitation after tritiated proline labeling and found that more than 90% of the labeled material in the vitreous was type II collagen. Snowden7 provided further physicochemical evidence in support of the similarities between vitreous and cartilage collagens. This may explain why certain clinical phenomena, such as inborn errors of type II collagen metabolism in arthro-ophthalmopathies, manifest phenotypic expression in each of these two tissues.
There are some distinct differences in the chemical composition of vitreous and cartilage collagens that are only partly owing to the presence of terminal peptide constituents in vitreous collagen.5 Swann and Sotman8 have demonstrated that the carbohydrate content of pepsin-solubilized vitreous alpha chains is significantly greater than that of cartilage alpha chains, indicating that the carbohydrate side chains of vitreous collagen are largely composed of disaccharide units similar to those found in basement membrane collagen. They proposed that these distinct chemical features are related to the special structure of the mature vitreous fibrils in vivo. Liang and Chakrabarti9 have shown that there are differences between bovine cartilage and vitreous with respect to collagen fibril growth, melting temperature, and fluorescence with a hydrophobic fluorescent probe. These investigators and others10 proposed that vitreous collagen should be considered a “special” type II collagen. Schmut et al11 used differential salt precipitation of pepsin-solubilized collagen from bovine vitreous and found that type II collagen is the major component of native vitreous fibers.
Gloor12 pointed out that collagen content is highest where the vitreous is a gel. Ayad and Weiss13 studied bovine vitreous collagen to determine whether the gel-like structure of vitreous could be explained on the basis of chemical composition. Their findings demonstrated that type II is the major vitreous collagen, but collagens composed of α1, α2, and α3 chains as well as C-PS disulfide-bonded collagen were present in concentrations similar to those in cartilage. In contrast to cartilage, however, vitreous type II collagen was significantly more hydroxylated in the lysine and proline residues. The α1, α2, and α3 collagen chains were interpreted by Van der Rest14 to represent type IX collagen, although Eyre et al15 felt that there was evidence to indicate the presence of type V collagen in vitreous. Furthermore, with respect to the disulfide-bonded collagen, vitreous had three times more C-PS1 and C-PS2 collagens than cartilage, although the molar ratio of C-PS 1 to C-PS2 in both was 1:1, suggesting that in both tissues these collagens are components of a larger molecule. Other studies14 demonstrated that these disulfide-linked triple-helix fragments were actually derivatives of type IX collagen. In this regard vitreous is again similar to cartilage, insofar as both contain type IX collagen,15 although the two tissues differ in the sizes of type IX collagen chains.16
Hong and Davison17 identified a procollagen in the soluble fraction of rabbit vitreous that was identified as type II by segment long-spacing banding patterns. Detection of a propeptide extension only at the N terminus prompted these investigators to conclude that this was a novel type II procollagen. More recent studies by Bishop et al18 confirmed the presence of this procollagen. These findings as well as the identification of immature cross-links in adult bovine vitreous19 and morphologic evidence of synthesis of vitreous collagen by the peripheral retina in the adult eye20 all suggest that postnatal collagen synthesis does occur, although probably only at a relatively low level. This question is relevant to both the origin of vitreous macromolecules as well as the question of vitreous physiology following vitrectomy. Concerning the former, studies by Newsome et al21 in the chick embryo implicated retinal Müller cells as the cells responsible for vitreous collagen synthesis during early development, whereas hyalocytes assume that responsibility later in life. Previous20 and recent in vitro22 studies confirmed that Müller cells can synthesize collagen. It does not necessarily follow, however, that these are responsible for vitreous collagen synthesis. Rather, another hypothesis proposes that the ciliary body is the site of synthesis of the macromolecules of both the vitreous body and the internal limiting lamina of the retina.23,24 What is not clear, however, is whether these macromolecules, particularly collagen, are resynthesized throughout life.
Balazs claimed that following vitreous removal there is no resynthesis of vitreous collagen, only hyaluronan. Recent studies, however, suggest that following vitrectomy a type II procollagen is secreted in humans.25 What is not clear, however, is whether this is de novo synthesis that is induced by the act of vitreous removal, or whether there is ongoing synthesis of vitreous collagen in the adult that is up-regulated after vitrectomy. Clinical experience would suggest that there is little or no up-regulation of collagen synthesis following vitrectomy, since reoperations reveal that the gel state of vitreous is not re-established, at least not in the first weeks or months following vitrectomy, the usual time frame in most clinical scenarios. Whether this is owing to a lack of collagen resynthesis or some other molecular component of vitreous that is responsible for gel formation is open to conjecture and serves as fertile ground for future research.
MAJOR VITREOUS COLLAGENS
Type II Collagen
Type II collagen constitutes 60% to 75% of the total collagen content in vitreous. It is a homotrimer composed of three identical alpha chains designated as [α1 (II)]3. When first synthesized as a procollagen and secreted into the extracellular space, type II collagen is highly soluble. The activity of N-proteinase and C-proteinase enzymes reduces the solubility and enables type II collagen molecules to cross-link covalently in a quarter-staggered array. Within this array are likely to be N-propeptides, which probably extend outward from the surface of the forming fibril.11 This may influence the interaction of the collagen fibril with other components of the extracellular matrix. Recent studies26 combined immunolocalization with Western blot analysis of macromolecules extracted from bovine vitreous collagen fibrils and found that the pN-type IIA procollagen is located on the surface of the vitreous collagen fibril. The finding27 that type IIA procollagen propeptides specifically bind transforming growth factor-beta 1 (TGFβ1) and bone morphogenetic protein 2 (BMP-2) supports the concept that, in certain circumstances, growth factors and cytokines interact with vitreous fibrils to promote the cell migration and proliferation that likely play a role in pathophysiology of proliferative diabetic retinopathy, proliferative vitreoretinopathy, and other proliferative disorders at the vitreoretinal interface.
Type IX Collagen
Type IX collagen accounts for up to 25% of vitreous collagen.28 It is a heterotrimer that is disulfide bonded and has a configuration of [α1 (IX) α2 (IX) α3 (IX)]. This heterotrimer is orientated regularly along the surfaces of the major collagen fibrils in a “D periodic” distribution, in which it is cross-linked onto the fibril surface. Type IX is not a typical collagen but is a member of the fibrillar-associated collagens with interrupted triple helixes (FACIT) group of collagens. It contains collagenous regions described as COL1, COL2, and COL3 interspersed between noncollagenous regions called NC1, NC2, NC3, and NC4.29,30 In vitreous (as opposed to cartilage) the NC4 domain is small and, therefore, not highly charged and not likely to exhibit extensive interaction with other extracellular matrix components.31 In vitreous, type IX collagen always contains a chondroitin sulfate glycosaminoglycan chain,29,30 which is linked covalently to the α2 (IX) chain at the NC3 domain; this enables the molecule to assume a proteoglycan form. Electron microscopy of vitreous stained with cationic dyes enables visualization of the chondroitin sulfate chains of type IX collagen. In some of these studies sulfated glycosaminoglycans are found distributed regularly along the surface of vitreous collagen fibrils32 and often bridge between neighboring collagen fibrils. Duplexing of glycosaminoglycan chains from adjacent collagen fibrils may result in a ladder-like configuration.33
Type V/XI Collagen
Less than 10% of vitreous collagen is a hybrid V/XI collagen, which is believed to compose the central core of the major collagen fibrils of vitreous (Fig. 38-1).34 Type V/XI is a heterotrimer that contains α1 (XI) and α2 (V) in two chains, but the nature of the third chain is presently not known.15 Along with type II collagen, type V/XI is a fibril-forming collagen. Although the interaction of the fibril with other extracellular matrix components is probably influenced by a retained N-propeptide that protrudes from the surface of the fibril in cartilage,34 it is not known whether this is the case in vitreous.35
MINOR VITREOUS COLLAGENS
Type VI Collagen
There are only small amounts of type VI collagen in vitreous, and it is not found in the major fibrils of vitreous (Fig. 38-1). Rather, type VI collagen in vitreous forms its own microfibrils. In spite of the paucity of this collagen in vitreous, the ability of this molecule to bind both type II collagen and hyaluronan (HA) suggests that it could be important in organizing and maintaining the supramolecular structure of vitreous gel.36,37 Other studies38 have detected type VI collagen at the vitreoretinal interface and suggested that this molecule may also play a role in maintaining vitreoretinal adhesion (discussed later in this chapter).
Type XVIII Collagen and Endostatin
One of the important functions of vitreous is the maintenance of transparency within the eye to enable the unhindered transmission of photons to the retina where photoreception occurs and vision begins.39 To do so, there is an important organization of the macromolecules within vitreous so as to minimize light scattering (discussed later in this chapter). Other potential sources of light scatter are cells, which are abundant in the tissues surrounding vitreous. Studies40 have shown that one of the minor collagens of vitreous is type XVIII, a progenitor of endostatin, which is known to be a potent inhibitor of angiogenesis. Subsequent studies41 mapped the distribution of endostatin and detected a pattern that encompassed the internal limiting lamina of the retina and the anterior vitreous behind the lens and the ciliary body. This distribution would provide potent inhibition of cell migration and proliferation within the vitreous body, contributing mightily to the maintenance of vitreous transparency.
GLYCOSAMINOGLYCANS
Glycosaminoglycans are polysaccharides of repeating disaccharide units, each consisting of hexosamine (usually N-acetyl glucosamine or N-acetyl galactosamine) glycosidically linked to either uronic (glucuronic or iduronic) acid or galactose. The nature of the predominant repeating unit is characteristic for each glycosaminoglycan, and the relative amount, molecular size, and type of glycosaminoglycan are said to be tissue specific.42 Glycosaminoglycans do not normally occur as free polymers in vivo but are covalently linked to a protein core; the ensemble is called a proteoglycan. A sulfated group is attached to oxygen or nitrogen in all glycosaminoglycans except HA. Balazs43 first documented the presence of sulfated galactosamine-containing glycosaminoglycans in bovine vitreous (<5% of total vitreous glycosaminoglycans), and others44,45 identified these as chondroitin-4-sulfate and undersulfated heparan sulfate. Studies in the rabbit46 found a total vitreous glycosaminoglycans content of 58 ng with 13% chondroitin sulfate and 0.5% heparan sulfate.
Hyaluronan
Although HA is present throughout the body, it was first isolated from bovine vitreous. In humans, HA first appears after birth and then becomes the major vitreous glycosaminoglycan. Although it has been proposed that hyalocytes synthesize HA,39 other plausible candidates are the ciliary body and retinal Müller cells. Whereas the synthesis of HA seems to continue at a constant rate in the adult while no extracellular degradation occurs, HA levels are in a steady state because the molecule escapes via the anterior segment of the eye.43 Laurent and Fraser47 showed that the passage of HA from the vitreous to the anterior segment is strongly molecular-weight-dependent, indicating a diffusion-controlled process. In contrast, disappearance of HA from the anterior chamber is independent of molecular weight, suggesting that this is controlled by bulk flow.
HA is a long, unbranched polymer of repeating glucuronic acid β-1,3-N,N-acetylglucosamine disaccharide moieties linked by β 1–4 bonds.5 It is a linear, left-handed, threefold helix with a rise per disaccharide on the helix axis of 0.98 nm.48 Rotary shadowing electron microscopy of human and bovine vitreous detected lateral aggregates of HA that form an anastomosing three-dimensional network.49 This periodicity, however, can vary depending on whether the helix is in a compressed or extended configuration.50 Changes in the degree of extension of HA could be important in the role vitreous plays in retinal disease. Indeed, the volume of the unhydrated HA molecule is about 0.66cm3/g, whereas the hydrated specific volume is 2,000 to 3,000 cm3/g.43 Thus, the degree of hydration has a significant influence on the size and configuration of the HA molecular network. Although there is no definitive evidence that adjacent HA chains bind to one another, Brewerton and Mayne51 first proposed such an arrangement. Recent rotary shadowing electron microscopy studies52 of bovine and human vitreous found lateral aggregates of HA that formed three-dimensional lattice-like networks.
HA is essentially a large polyanion whose surface charge can influence both the entire vitreous body as well as diffusion of substances through the vitreous. Regarding the former, HA interaction with surrounding mobile ions can induce changes in HA conformation that are owing to changes in the surrounding ionic milieu.53 A decrease in surrounding ionic strength can cause the anionic charges on the polysaccharide backbone to repel one another, resulting in an extended configuration of the macromolecule. An increase can cause contraction of the molecule and, in turn, the entire vitreous body. As a result of HA’s entanglement and immobilization within the vitreous collagen fibril matrix, this mechanical force can be transmitted by collagen fibrils to the retina, optic disc, and other structures such as neovascular complexes. In this way, changes in the ionic milieu of vitreous may be converted into mechanical energy via extension or contraction of the HA macromolecule. This can be important in certain pathologic conditions that feature fluctuations in ionic balance and hydration, such as diabetes.54 Diffusion of substances through vitreous is an important consideration, since one of the most frequently performed procedures in ophthalmology today is the injection of drugs intended to treat the retina (and in the future the optic nerve) into vitreous. Studies55 have identified that anionic particles have more rapid diffusion through vitreous, probably because of the anionic charge of the HA molecule.
The sodium salt of HA has a molecular weight of 3 to 4.5 × 106 Da in normal human vitreous.43 Laurent and Granath56 used gel chromatography and found the average molecular weight of rabbit vitreous to be 2 to 3 × 106 Da and of bovine vitreous to be 0.5 to 0.8 × 106 Da. In these studies there were age-related differences in the bovine vitreous, in which HA molecular weight varied from 3 × 106 Da in the newborn calf to 0.5 × 106 Da in old cattle. Furthermore, there may be several species of HA within vitreous that have polysaccharide chains of different lengths57 with a variable distribution in different topographic regions within the vitreous body.58
An important property of HA is steric exclusion.59 With its flexible linear chains and random coil conformation, HA occupies a large volume and resists the penetration of this volume by other molecules to a degree dependent on their size and shape.50 This excluded volume effect can influence equilibria between different conformational states of macromolecules and alter the compactness or extension of these molecules.59 Steric exclusion also causes an excess of osmotic pressure when such compounds as albumin and HA are mixed, because the resultant osmotic pressure is greater than the sum of the two components. This could be important in diabetes, in which vascular incompetence can increase vitreous concentrations of serum proteins such as albumin. These osmotic effects can induce contraction and expansion of the vitreous body similar to the foregoing description of hydration and ion-induced changes within vitreous and can similarly play an important role in neovascularization and diabetic vitreous hemorrhage.54 An increase in the chemical activity of a compound because of steric exclusion can also cause its precipitation if the solubility limit is reached. This could be important in the formation of pathologic vitreous opacities, such as asteroid hyalosis and amyloid bodies.39
Chondroitin Sulfate
Vitreous contains two chondroitin sulfate proteoglycans. The minor type is actually type IX collagen, which was described previously. Most vitreous chondroitin sulfate is in the form of versican.52 This large proteoglycan has a globular N terminal that binds HA via a 45-kDa link protein.52 Thus, in human (but not bovine) vitreous, versican is believed to form complexes with HA, as well as with microfibrillar proteins such as fibulin-1 and fibullin-2.35 Recent studies60 have suggested that versican has the ability to bind hyaluronan via its N terminal and other binding partners via its C-terminal region and thus can play a crucial role in maintaining structural stability of the vitreous.
Heparan Sulfate
This sulfated proteoglycan is normally found in basement membranes and on cell surfaces throughout the body. It was first detected in bovine vitreous in 197745 and in chick vitreous (as agrin) in 1995.61 However, it is not clear whether heparan sulfate is a true component of vitreous or a contaminant from adjacent basement membranes, such as the internal limiting lamina (ILL) of the retina.62 As pointed out by Bishop35 this may also be the case for nodogen-1, fibrillins, and fibronectin.
NONCOLLAGENOUS STRUCTURAL PROTEINS
Fibrillins
Fibrillin-containing microfibrils are more abundant in vitreous than the type VI collagen microfibrils described previously. These beaded microfibrils are found in vitreous gel as well as in the zonules of the lens, which explains why in Marfan syndrome the defects in the gene encoding fibrillin-1 (FBN1 on chromosome 15q21) result in both ectopia lentis and vitreous liquefaction.35 The latter probably plays a role in the frequent occurrence of rhegmatogenous retinal detachment in these patients. In many tissues, fibrillin-containing microfibrils provide a scaffold for elastin assembly, but in the zonules and vitreous they are not associated with elastin. The major component of these microfibrils is fibrillin-1; this is a glycoprotein of 350 kDa containing multiple calcium-binding epidermal growth factor (EGF)-like domains. It has been shown60 that the N-terminal region of fibrillin-1 binds to microfibril-associated glycoprotein-1 in these microfibrils.
Opticin
The major noncollagenous protein of vitreous is a leucine-rich repeat (LRR) protein, which is bound to the surface of the heterotypic collagen fibrils, known as opticin.63 Formerly called vitrican, opticin is believed to be important in collagen fibril assembly and in preventing the aggregation of adjacent collagen fibrils into bundles. Thus, a breakdown in this property or activity may play a role in age-related vitreous degeneration64 (discussed later in this chapter). Recent studies have demonstrated that opticin binds glycosaminoglycans (GAGs), including heparan sulphate (HS) and chondroitin sulphate.65,66 These findings suggest that by binding heparan sulfate proteoglycans, opticin may play a role in vitreoretinal adhesion by providing a link between cortical vitreous collagen fibrils and the inner limiting lamina. Opticin may also stabilize vitreous gel structure by binding chondroitin sulfate proteoglycans. Interestingly, opticin is also found in articular cartilage67 where it is hypothesized to render stability to the joint structure, once again drawing a corollary between vitreous and joints.
VIT 1
Another novel vitreous protein is VIT1, a collagen-binding macromolecule.68 Because of its propensity to bind collagen, this highly basic protein may also play a role in maintaining vitreous gel structure.
MISCELLANEOUS MOLECULAR COMPONENTS
Amino Acids and Proteins
Free amino acids are present in the vitreous, but at one fifth of the levels found in plasma.69 An amino acid concentration gradient is present within the vitreous body, with anterior vitreous concentrations greater than posterior levels. This may be owing to uptake and utilization of amino acids by the retina, a consideration that led Reddy70 to propose that the vitreous acts as a metabolic repository for retinal protein metabolism. Other studies71 found that the soluble proteins of vitreous resemble serum proteins with isoelectric points less than 6.0, leading investigators to conclude that the soluble proteins of vitreous derive from plasma and are constantly renewed. Flood, who is cited in Balazs and Denlinger,72 studied 920 human eyes and found the following age-related protein concentrations: ages 10 to 50, 400 to 600 μg/mL; ages 50 to 80, 700 to 800 μg/mL; older than 80 years of age, about 1,000 μ/mL. These age-related findings may be owing to increased leakage of plasma proteins from the intravascular compartment into the vitreous, resulting from decreased tight-junction integrity with aging of the retinal and ciliary body vasculature and epithelia.
Glycoproteins
Glycoproteins are heteropolysaccharide macromolecules, as opposed to the homogeneous, repeating disaccharide units of glycosaminoglycans that are mostly proteinaceous and contain only a minor carbohydrate component (5%–10% by weight). According to Balazs,43 the most important difference between vitreous and serum proteins is the high content of glycoproteins in the vitreous, because these constitute 20% of the total noncollagenous protein content of vitreous. Sialic acid–containing glycoproteins are believed to be synthesized by hyalocytes.73 Other studies74 have led to the postulate that the inner layer of the ciliary epithelium is responsible for vitreous glycoprotein synthesis. Cartilage oligomeric protein (COMP), an acidic glycoprotein with a characteristic five-armed structure, is present in vitreous, but its function is currently unknown.
Ascorbic Acid
Ascorbic acid concentrations in vitreous are about 0.43 mmol/kg.75 Thus, the vitreous-plasma ratio for ascorbic acid is 9:1. Vitreous levels that are much higher than plasma concentrations are believed to be owing to active transport by the ciliary body epithelium.76 The purpose of having high concentrations of ascorbic acid in the vitreous may relate to the abilities of this compound to absorb ultraviolet light and to serve as a free-radical scavenger,77 which may protect not only the retina and lens but also vitreous from the untoward effects of metabolic and light-induced78 singlet oxygen generation.
Lipids
Swann et al79 found that the residual fraction of vitreous contained a significant quantity of lipid. Later studies80 found evidence of active lipid metabolism in vitreous of dogs and humans, but in the latter species no significant changes in vitreous lipid composition between the ages of 37 and 82 years.
SUPRAMOLECULAR ORGANIZATION
As described by Mayne,81 vitreous is organized in a dilute meshwork of collagen fibrils interspersed with extensive arrays of long HA molecules. The collagen fibrils provide a scaffold-like structure that is inflated by the hydrophilic HA. If collagen is removed, the remaining HA forms a viscous solution; if HA is removed, the gel shrinks53 but is not destroyed. Early physiologic observations82 suggested the existence of an interaction between HA and collagen that stabilizes collagen. Biomechanical studies83 of vitreous viscoelasticity suggested similar effects on HA. Early investigators84 observed reversible complexes of an electrostatic nature between solubilized collagen and various glycosaminoglycans and suggested that collagen-HA interaction occurs on a physicochemical rather than chemical level. Others85 later demonstrated that the sulfate group of a glycosaminoglycan is largely responsible for such interactions with the guanidino groups of arginine and epsilon-amino groups of lysine in collagen. Comper and Laurent53 proposed that in vitreous electrostatic binding occurs between negatively charged polysaccharides and positively charged proteins. These authors extensively reviewed the existing data characterizing the electrostatic properties of glycosaminoglycans and the factors influencing their electrostatic interactions with different ions and molecules.
Balazs43 hypothesized that the hydroxylysine amino acids of collagen mediate polysaccharide binding to the collagen chain via O-glycosidic linkages. These polar amino acids are present in clusters along the collagen molecule, which may explain why proteoglycans attach to collagen in a periodic pattern.32 Hong and Davison17 identified a type II procollagen in the soluble fraction of rabbit vitreous and proposed a possible role for this molecule in mediating collagen-HA interaction. In cartilage, “link glycoproteins” have been identified that interact with proteoglycans86 and HA.87 Supramolecular complexes of these glycoproteins are believed to occupy the interfibrillar spaces. In Asakura’s32 studies of bovine vitreous stained with ruthenium red there are amorphous structures located on collagen fibrils at 55- to 60-nm intervals along the fibrils and filaments connecting these amorphous masses to adjacent collagen fibrils. These filaments may represent link structures of either a glycoprotein or proteoglycan nature. HA is known to interact with link proteins, as well as with an HA-binding glycoprotein called hyaluronectin.88 In the cornea, chondroitin sulfate and keratan sulfate bridge the interfibrillar spaces and keep the fibrils at specified distances to achieve transparency.89 The protein cores of these proteoglycans could be the linkage sites to collagen fibrils.
Bishop35 has proposed that an understanding of how vitreous gel is organized and stabilized requires an understanding of what prevents collagen fibrils from aggregating and by what means the collagen fibrils are connected to maintain a stable gel structure. Studies90 have shown that the chondroitin sulfate chains of type IX collagen bridge adjacent collagen fibrils in a ladder-like configuration spacing them apart. This arrangement might account for vitreous transparency, because keeping vitreous collagen fibrils separated by at least one wavelength of incident light would minimize light scattering, allowing unhindered transmission of light to the retina for photoreception. However, depolymerizing with chondroitinases does not destroy the gel, suggesting that chondroitin sulphate side chains are not essential for vitreous collagen spacing. Complexed with HA, however, the chondroitin sulfate side chains might space the collagen fibrils33,89,90 apart, but Bishop believes that this form of collagen-HA interaction is very weak. Instead, he proposes that the LRR protein opticin is the predominant structural protein in short-range spacing of collagen fibrils. Concerning long-range spacing, Scott33 and Mayne et al91 have claimed that HA plays a pivotal role in stabilizing the vitreous gel, supporting the early beliefs of Balazs. However, studies92 using lyase to digest vitreous HA demonstrated that the gel structure was not destroyed, suggesting that HA is not essential for the maintenance of vitreous gel stability and leading to the proposal that collagen alone is responsible for the gel state of vitreous.35
Recent studies93 have provided evidence to support the hypothesis that versican plays a crucial role in the supramolecular organization of vitreous by dint of versican’s ability to bind hyaluronan via its N terminal and other binding partners via its C-terminal region, resulting in structural stability of the vitreous. The binding of versican to HA is stabilized by a glycoprotein called link protein, which has also been identified in vitreous.52 Mutations that alter the splicing of the central chondroitin sulphate–bearing domains of versican have been implicated in the vitreoretinopathy Wagner syndrome, a condition with excess vitreous liquefaction.94
STRUCTURE
HISTORICAL PERSPECTIVE
Duke-Elder95 claimed that the first theories of vitreous structure proposed that the vitreous is composed of “loose and delicate filaments surrounded by fluid,” as conceptualized in 1741 by Demours,96 who formulated the alveolar theory. In 1780 Zinn97 proposed that the vitreous is arranged in a concentric, lamellar configuration similar to the layers of an onion. The dissections and histologic preparations of Von Pappenheim98 and Brucke99 provided evidence for the lamellar theory. The radial sector theory was proposed by Hannover in 1845.100 Studying coronal sections at the equator, he described a multitude of sectors approximately radially oriented around the central anteroposterior core that contains the Cloquet canal. Hannover likened this structure to the appearance of a cut orange. In 1848 Bowman101 introduced the fibrillar theory. Using microscopy, he described fine fibrils that form bundles that Retzius102 described as fibrous structures arising in the peripheral anterior vitreous that assume an undulating pattern similar to a horse’s tail in the central vitreous but maintain a concentric configuration at the periphery. The elegant studies of Szent-Gyorgi103 in 1917 supported the descriptions of Retzius and introduced the concept that vitreous structure changes with age. Eisner104 studied dissections of human vitreous and found “membranelles,” which he described as funnels packed into one another diverging outward and anteriorly from the prepapillary vitreous. Worst105 has also studied preparations of dissected human vitreous and described that the tracts of Eisner constitute the walls of cisterns within the vitreous body. In Worst’s studies these cisterns are visualized by filling with white India ink. Worst has also studied the premacular vitreous in great detail and has proposed the existence of a “bursa premacularis,” which he described as a pear-shaped space that is connected to the cisternal system in front of the ciliary body. Kishi and Shimizu106 recently described a “posterior vitreous pocket” similar to what Worst has reported, which they claim to be an anatomic structure. However, because more than 95% of their study population was age 65 years or older, this is likely to be an age-related phenomenon.107
VITREOUS BODY
There is heterogeneity in the distribution of collagen throughout the vitreous body. Chemical108,109 and light-scattering110,111 studies have shown that the highest density of collagen fibrils is present in the vitreous base, followed by the posterior vitreous cortex anterior to the retina, and then by the anterior vitreous cortex behind the posterior chamber and lens. The lowest density is found in the central vitreous and adjacent to the anterior cortical gel. HA molecules have a different distribution from collagen. They are most abundant in the posterior cortical gel with a gradient of decreasing concentration as one moves centrally and anteriorly.112,113,114 Balazs114 has hypothesized that this is because vitreous HA is synthesized by hyalocytes in the posterior vitreous cortex and cannot traverse the ILL of the retina. HA leaves the vitreous to enter the posterior chamber by way of the annulus of the anterior vitreous cortex that is not adjacent to a basal lamina. Bound water (nonfreezable) has a distribution within the vitreous similar to that of HA,115 presumably resulting from binding by HA.
Laboratory investigations of vitreous structure have long been hampered by the absence of easily recognized landmarks within the vitreous body. Consequently, removal of the vitreous from the eye results in a loss of orientation. The transparency of the vitreous renders observation in conventional diffuse light unrewarding. Attempts to study vitreous structure with opaque dyes105 do visualize the areas filled by dye but obscure the appearance of adjacent structures. The use of histologic contrast-enhancing techniques usually involves tissue fixation, which often includes dehydration of the tissue. Because vitreous is 98% water, dehydration induces profound alteration of the internal morphology. Consequently, any investigation of vitreous structure must overcome these difficulties.
The sclera, choroid, and retina can be dissected and the naked vitreous body can be maintained intact and attached to the anterior segment of the eye (Fig. 38-2A). This enables study of internal vitreous morphology without a loss of intraocular orientation. However, depending on the person’s age and consequently the degree of vitreous liquefaction,72 the dissected vitreous will remain solid and intact in young persons or will be flaccid and collapse in older individuals. Consequently, vitreous turgescence must be maintained to avoid distortion of intravitreal structure. Immersion of a dissected vitreous specimen that is still attached to the anterior segment into a physiologic solution maintains vitreous turgescence and avoids structural distortion (Fig. 38-2B). The limitations induced by the transparency of the vitreous were overcome by Goedbloed,116 Friedenwald and Stiehler,117 and Eisner104 who employed darkfield slit illumination of the vitreous body to achieve visualization of intravitreal morphology. Illumination with a slitlamp beam directed into the vitreous body from the side and visualization of the illuminated portion from above produces an optical horizontal section of the vitreous body.118 The illumination/observation angle of 90° that is achieved by using this technique maximizes the Tyndall effect and, thus, overcomes the limitations induced by vitreous transparency. Furthermore, the avoidance of any tissue fixation eliminates the introduction of many of the artifacts that flawed earlier investigations. Recent studies have used these techniques to investigate human vitreous structure. Within the adult human vitreous there are fine, parallel fibers coursing in an anteroposterior direction39,118,119,120 (Figs. 38-3B and C and 38-4). The fibers arise from the vitreous base (Figs. 38-3H and 38-4) where they insert anterior and posterior to the ora serrata (Fig. 38-3H). As the peripheral fibers course posteriorly they are circumferential with the vitreous cortex, whereas central fibers undulate in a configuration parallel with the Cloquet canal.102 The fibers are continuous and do not branch. Posteriorly, these fibers insert into the vitreous cortex (Figs. 38-3E and F).
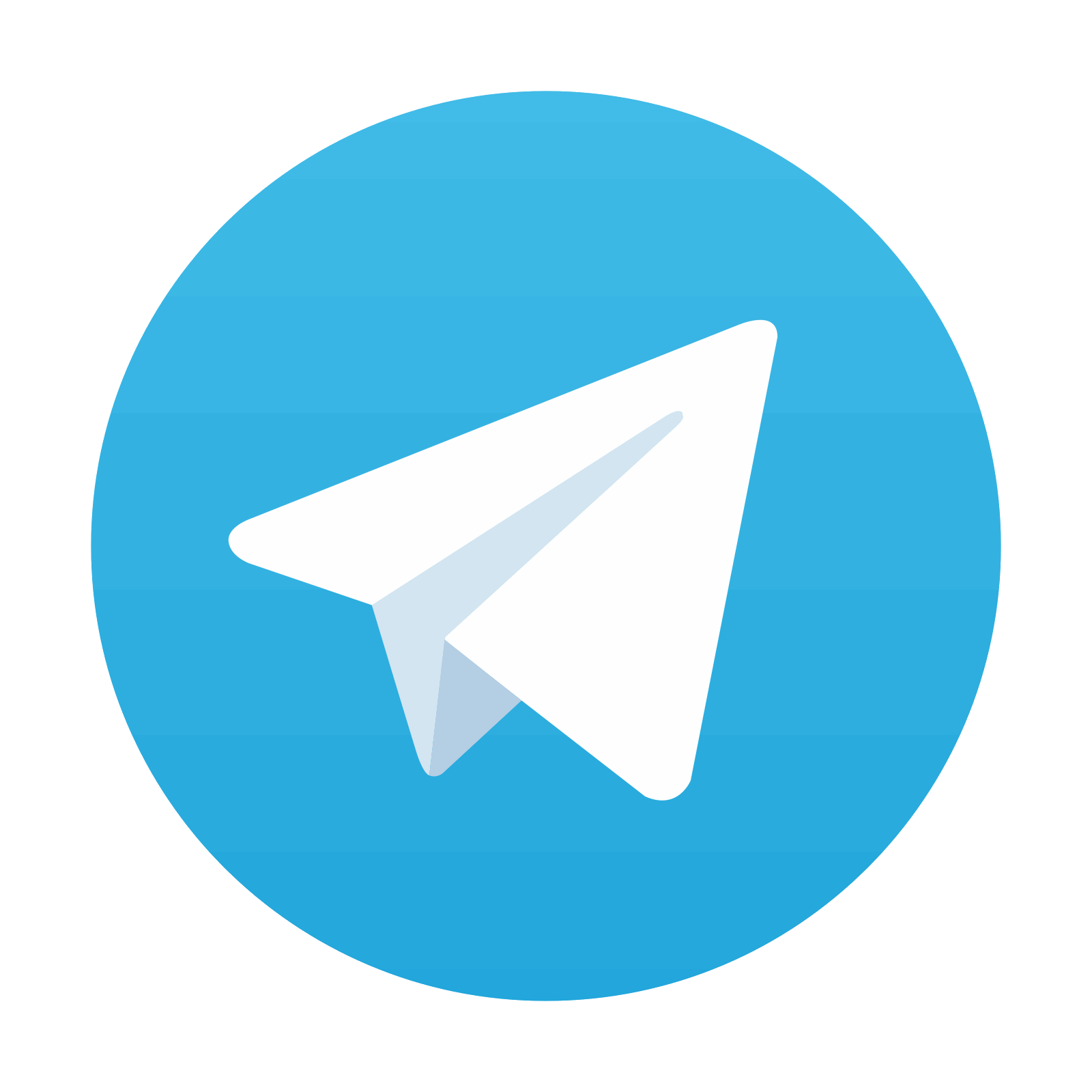
Stay updated, free articles. Join our Telegram channel

Full access? Get Clinical Tree
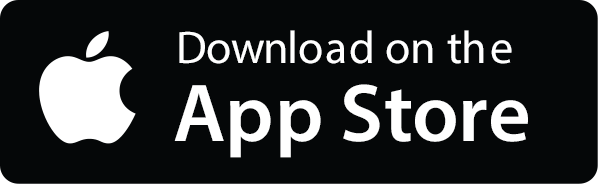
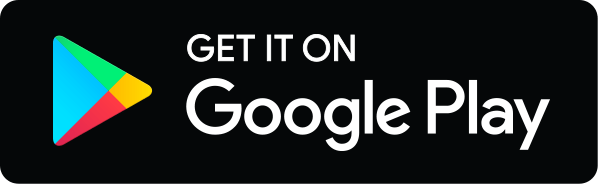