CHAPTER 154 Vestibular and Auditory Ototoxicity
Aminoglycoside Antibiotics
The aminoglycoside antibiotics are an important class of anti-infectious agents. They were developed to combat tuberculosis and other life-threatening infections. The first members of this class of drugs were streptomycin and dihydrostreptomycin. Initial clinical trials showed that these compounds could damage the kidneys and the inner ear. Since then, many new aminoglycosides have been developed. Dihydrostreptomycin proved to be too toxic and was taken off the market. Neomycin was found to be too toxic for systemic use and has been relegated to local application. Other members of this group of drugs include kanamycin, gentamicin, tobramycin, amikacin, netilmicin, and sisomicin. Some of these agents are more toxic to either the cochlea or the vestibular apparatus, although their ototoxicity is not completely selective. Toxicity generally occurs only after days or weeks of exposure. The overall incidence of aminoglycoside auditory toxicity is estimated to be approximately 20%, whereas vestibulotoxicity may occur in about 15%.1
Pharmacokinetics
Aminoglycosides are highly charged molecules and are poorly absorbed orally. Only approximately 3% of an orally administered dose is absorbed from the gastrointestinal tract. Aminoglycosides are normally used parenterally for severe systemic infections. The concentrations of aminoglycosides in tissues are usually approximately one third of the corresponding serum concentration. Penetration of the blood-brain barrier is generally poor, so aminoglycosides are injected intrathecally to treat meningitis. Previous studies have suggested that aminoglycosides are not metabolized. A toxic metabolite may be formed in the inner ear, however.1 Aminoglycosides are excreted primarily by the kidney by glomerular filtration, and high concentrations of drug in the urine may be achieved. Impaired renal function reduces the rate of excretion. Renal failure is a risk factor for ototoxicity, and dosing of aminoglycosides must be modified to compensate for delayed renal excretion.
Measurement of peak and trough serum levels of aminoglycosides provides rough guidelines for therapeutic efficacy, but is not an absolute guarantee for prevention of ototoxicity, particularly vestibular ototoxicity. An association between trough levels of aminoglycosides and ototoxicity has been pointed out.2 Ototoxicity is likely related to the area under the curve of blood levels over time, rather than individual peak and trough measurements.3 An animal study showed no relationship between ototoxicity and plasma level of aminoglycoside. The total dose or area under the curve was a much better predictor of amikacin ototoxicity in this animal model.4
Suggested guidelines for monitoring serum levels of aminoglycosides are as follows:
Aminoglycoside ototoxicity may be detected, but because of a life-threatening infection and a lack of suitable alternative antibiotic therapy, it may be necessary to continue treatment. Antibiotic ototoxicity may continue even after cessation of aminoglycoside therapy.5
More recent studies in animals and in humans have shown the presence of a metabolite of streptomycin in the serum of patients with tuberculosis treated with streptomycin. Five male patients treated with streptomycin for 35 to 90 days with 1 g/day presenting with mild to severe inner ear malfunction were found to have streptidine present. Streptidine may represent an ototoxic metabolite of streptomycin.6
Histopathology
Animal and human temporal bone histopathologic studies show that the cochlear and vestibular hair cells serve as primary targets for injury. In the organ of Corti, the outer hair cells of the basal turn are damaged first. As drug treatment is continued, the damage may spread to more apical regions. Inner hair cells seem to be more resistant to injury than outer hair cells. This difference could be a result of the higher concentration of the natural antioxidant, glutathione, in the inner hair cells and in the apical turn outer hair cells compared with in the outer hair cells of the basal turn.7 Progressive destruction of spiral ganglion cells has been shown in animal studies and in human temporal bone reports.8,9 In some patients, spiral ganglion cells may be damaged directly by aminoglycosides without injury to outer hair cells.10 The stria vascularis may become thinner as a result of marginal cell death.11 In the vestibular system, the hair cell damage may begin in the apex of the cristae and in the striola regions of the maculi.12 Hair cell destruction may extend to the periphery of the vestibular sensory epithelium, where type I hair cells are primarily affected.13
A quantitative study of 17 temporal bones was carried out in patients with well-documented clinical evidence of aminoglycoside ototoxicity, and the results were compared with age-matched controls. Streptomycin caused a significant loss of type I and type II hair cells in all vestibular organs, with a greater loss of type I hair cells in the cristae, but not in the maculae. The vestibular toxic effects of kanamycin seemed to be similar to streptomycin, but neomycin did not cause the loss of any vestibular hair cells. There was no significant loss of Scarpa’s ganglion cells for any of the aminoglycosides.14
Clinical Manifestations
High-frequency hearing loss tends to occur first and may be detectable before it becomes clinically noticeable.15 Continued exposure to aminoglycosides may result in hearing loss that progresses to lower frequencies, including the important speech range, interfering with communication skills. A hearing loss of 20 dB or more at two or more adjacent frequencies should be documented to confirm the diagnosis of drug-related hearing loss after exclusion of other causes of hearing loss.
Delayed ototoxicity may occur after cessation of treatment with aminoglycoside antibiotics. Delayed onset of hearing loss usually manifests within 1 to 3 weeks after the end of therapy.16
Onset of vestibular ototoxicity is unpredictable, and may not correlate with cumulative dose of ototoxic medication.17 Ambulatory patients are asymptomatic until they notice the presence of imbalance and ataxia, dramatically worsened by motion, with relative freedom from symptoms during periods of complete immobility. Patients change from being normal to being symptomatic during an easily identifiable 1- or 2-day period, after which symptoms are always present during motion or ambulation. The severity of symptoms varies, ranging from imbalance and staggering to complete inability to walk without assistance. Severely affected individuals also usually experience oscillopsia. Although some clinical improvement can be seen beginning 2 months after onset of symptoms, it is rarely complete.18
Rotational vestibular testing in a few patients indicated that results were normal in patients receiving aminoglycoside therapy who were asymptomatic. When abnormalities were observed, as they always were in symptomatic patients, the low-frequency rotational responses were affected first and most severely. When responses to all frequencies were absent, oscillopsia and severe imbalance were usually present. Improvement in function sometimes could be shown by rotational testing. This improvement was always greatest in the high frequencies, and clinical recovery was often not as great as the improvements in test results would have suggested.18
Various risk factors have been studied to determine whether they play a role in increasing the likelihood of ototoxicity in various patient populations. Bacteremia, fever, hepatic dysfunction, and renal dysfunction have been associated with ototoxicity in prospective, double-blind clinical trials of gentamicin, tobramycin, and amikacin.19 Combinations of another ototoxic drug and an aminoglycoside may increase the risk and severity of ototoxicity. Ethacrynic acid was reported to exacerbate the ototoxicity of aminoglycosides in patients with uremia.3 Furosemide was reported to result in severe sensorineural hearing loss (SNHL) in a patient who had received only five doses of gentamicin for pneumonia.20 An extremely important risk factor is a mitochondrial RNA mutation, which dramatically increases the risk of ototoxicity. This mutation may sensitize a patient to even a single dose of aminoglycoside. This mutation follows a maternal inheritance pattern, and has been described in Chinese, Arab-Israeli, Japanese, and North American families.21 Seventeen percent of patients with aminoglycoside-induced hearing loss may have this mutation.1
Mutations in mitochondrial 12S ribosomal RNA render patients highly susceptible to aminoglycoside ototoxicity. The first described mutation was an A1555G mutation in the 12S rRNA gene. Mutations in the mitochondrial 12S rRNA make this mammalian RNA more similar to the bacterial rRNA, the primary target of the bactericidal activity of aminoglycosides.22 This mutation has been associated with spontaneous and aminoglycoside-induced hearing loss. In China, where this mutation seems to occur in 5% to 6% of sporadic patients, approximately one third of patients with aminoglycoside ototoxicity seem to have the A1555G mutation.22 For an unknown reason, only the auditory system, but not the vestibular part of the inner ear, is sensitized to the toxic effects of aminoglycosides in patients with this mutation.1
The risks of intratympanic therapy for Meniere’s disease and the use of potential otoprotective agents to prevent or reduce aminoglycoside ototoxicity are discussed in greater detail in Chapters 149, 155, and 165.
Mechanisms of Ototoxicity
Aminoglycosides, such as dihydrostreptomycin, enter the mouse outer hair cell through the mechanoelectrical transduction channel and act as a permeant blocker.23,24 This channel can act as a one-way valve for aminoglycoside entry, promoting the accumulation of aminoglycoside within the cell. There seems to be a competition between the aminoglycoside and calcium for entry into the outer hair cell. The normal low endolymph concentration of calcium promotes intracellular accumulation of aminoglycosides.24
ROS formation by aminoglycosides in vitro requires iron and the presence of polyunsaturated lipids as electron donors. Electron spray ionization mass spectrometry showed that gentamicin strongly binds to L-R-phosphatidylinositol 4,5-bisphosphate (PIP2), a membrane lipid rich in arachidonic acid. Studies using lipid-coated membranes confirmed that iron ions and gentamicin can form ternary complexes among gentamicin, iron, and phospholipids. Peroxidation of PIP2 by ferrous ions significantly increased in the presence of gentamicin, and oxidative damage to PIP2 was accompanied by the release of arachidonic acid. Arachidonic acid also forms a ternary complex with Fe2+/Fe3+-gentamicin. This complex reacts with lipid peroxides and molecular oxygen, leading to arachidonic acid peroxidation.25 These ROS can react with various cell components, including the phospholipids in the cell membrane, proteins, and DNA to disrupt the function, primarily in the outer hair cell. This process can trigger programmed cell death, resulting in apoptosis.1
Data from experimental studies strongly suggest that aminoglycosides trigger apoptosis at clinically relevant doses,26 whereas higher doses may trigger necrotic cell death.27 The ototoxic effects of neomycin28,29 seem to be mediated by c-Jun N-terminal kinase (JNK). JNK30 and extracellular regulated kinase (ERK) 1/2 mitogen-activated protein kinases seem to be associated with gentamicin-induced apoptosis in the inner ear.31 Gentamicin seems to trigger apoptosis by ROS and to be mediated by Ras/Rho GTPases.30
Inhibitors of apoptosis proteins play an important role in regulating apoptosis by preventing activation of effector caspases. The effects of specific inhibitors of the X-linked inhibitor of apoptosis (XIAP) on hair cell death caused by gentamicin were examined in basal turn organ of Corti explants from postnatal day 3 or 4 rats in tissue culture exposed to gentamicin. XIAP inhibitors increased gentamicin-induced hair cell loss, but an inactive analog had no effect. A caspase-3 inhibitor decreased caspase-3 activation and hair cell loss from gentamicin plus an XIAP, but caspase-8 and caspase-9 inhibitors did not. These findings indicate that XIAP acts normally to decrease caspase-3 activation and hair cell loss during gentamicin ototoxicity.32
Apoptosis may be related to the enzyme protein kinase C zeta. Amikacin treatment in animals induced the cleavage of this enzyme and nuclear translocation, leading to chromatin condensation and decreased nuclear factor kappa B (NFκB) levels in the nucleus. These deleterious effects were prevented by pretreatment with aspirin, resulting in protection of hair cells and spiral ganglion neurons.33
Concentration-dependent protection against the ototoxicity of kanamycin combined with ethacrynic acid was found in guinea pigs receiving dexamethasone by intracochlear administration with an osmotic minipump before and after the ototoxic treatment. A statistically significant preservation of outer hair cell counts and lower auditory brainstem evoked potential threshold shifts were found in the dexamethasone-infused ears. No significant rescue was observed.34
Antineoplastic Agents
Cisplatin
Pharmacokinetics
The pharmacokinetics of cisplatin follow a biphasic clearance pattern. After a 1-hour intravenous infusion of 70 mg/m2, the plasma half-lives in patients were 23 minutes and 67 hours; 17% ± 2.7% of the dose administered was excreted in the urine within the first 24 hours, primarily by glomerular filtration. Cisplatin is extensively bound to serum proteins. This bound form is inactive against tumor cells. The half-lives of free cisplatin in serum are much shorter than the total platinum, with half-lives of 8 minutes (free cisplatin) and 40 to 45 hours (total platinum).35 The liver rapidly converts cisplatin into nontoxic metabolites within 1 hour after administration. The cytosol of the liver cells seems to form adducts of cisplatin with glutathione and cysteine. Cisplatin is taken up into the cells of the cortex and outer medulla of the kidney during the time when nephrotoxicity begins.36
Clinical Ototoxicity
Hearing loss caused by cisplatin seems to be highly variable, and seems to be related to dose; age of the patient; and other factors such as noise exposure,37 exposure to other ototoxic drugs, depleted nutritional state including low serum albumin and anemia,38 and cranial irradiation.39 Children seem to be more susceptible than adults.40 Hearing loss tends to be permanent and bilaterally symmetric. Symptoms of ototoxicity include subjective hearing loss, ear pain, and tinnitus.41 Tinnitus has been reported in 2% to 36% of patients treated with cisplatin, and may be transient or permanent.41 Although higher frequencies are affected first, hearing impairment may extend into the middle frequency range when doses greater than 100 mg/m2 are used. When ultra-high-frequency audiometric testing is used, 100% of patients receiving high-dose cisplatin (150 to 225 mg/m2) may show some degree of hearing loss.38 Dose-related ototoxicity is illustrated by a report that 20% of patients treated with cisplatin for testicular cancer experienced permanent ototoxicity, but more than 50% of patients who received cisplatin in doses of greater than 400 mg/m2 cumulative dose had permanent hearing loss.37
Pediatric patients were studied for cisplatin ototoxicity by use of otoacoustic emissions. A good correlation between transient otoacoustic emissions and pure-tone audiometric threshold was shown in children with normal middle ear function. In this series, 90.5% of patients had a significant SNHL at 8 kHz. The magnitude of the hearing loss was associated with young age at first dose of cisplatin administration, large number of cycles of chemotherapy, and high cumulative dose of cisplatin.42
The incidence and severity of hearing loss are reportedly enhanced by cranial irradiation.39 Patients with nasopharyngeal carcinoma seem to be particularly susceptible to the interaction of cochlear irradiation and cisplatin chemotherapy. The required dose of radiation that acts on the cochlea is greater than 48 Gy in such patients.43 A more recent study reported that radiation therapy for brain tumors, such as medulloblastoma, can be modified to reduce the doses of radiation to the cochlea, while still delivering full doses to the desired target volume of tissue. Using the technique of intensity-modulated radiation therapy for these tumors, far fewer patients had hearing loss. Only 13% of patients receiving the intensity-modulated radiation therapy protocol with cisplatin had grade 3 or 4 hearing loss compared with 64% of the conventional radiation therapy group.39
Whether hearing loss progresses after cessation of cisplatin chemotherapy has been a matter of controversy. It has been reported that 8 years after cisplatin therapy for testicular cancer, elevated urinary and serum platinum levels can be measured; nevertheless, no long-term toxicity was detected in these patients.44 Long-term audiometric studies of 26 women treated with moderate-dose cisplatin (50 mg/m2 body surface area every 4 weeks) for gynecologic cancer showed progressive hearing loss equal to or greater than 15 dB at long-term (59 to 115 months after treatment) follow-up in 14 patients (54%). These changes were considered to be small and restricted to three frequencies or fewer in one ear. Only two patients (8%) showed more severe threshold changes. The authors concluded that adult patients who receive treatment with moderate-dose cisplatin have a negligible long-term risk of drug-induced social disability from hearing.45
Delayed ototoxicity from cisplatin seems to be much more significant in children. Pediatric patients treated with cisplatin at cumulative doses approaching 400 mg/m2 showed worsening of hearing with time after treatment. Audiograms showed hearing loss in 5% of patients before the end of therapy. After more than 2 years of follow-up, 44% had significant hearing loss.46 A more recent study found that the median time for first significant decrease in hearing was 135 days in children. Additional follow-up for 6 to 44 months showed mild further progression of hearing loss of 10 to 15 dB after completion of therapy.47
Studies of vestibular function in patients receiving cisplatin chemotherapy have suggested vestibulotoxic effects, especially in patients with preexisting vestibular problems.48 With the vestibular autorotation test to study the vestibulo-ocular reflex, patients receiving cisplatin were screened for vestibulotoxicity. Vestibulo-ocular reflex gains at 3.1 Hz, 3.9 Hz, and 5.1 Hz were decreased, and phase lags at 3.1 Hz and 3.9 Hz were increased.49
Because chemoradiation protocols with intra-arterial cisplatin include thiosulfate protection, one may think that there would be less ototoxicity with this regimen than with intravenous cisplatin. This does not seem to be the case, however. A prospective study50 evaluated 146 patients receiving high-dose intra-arterial cisplatin chemotherapy (150 mg/m2 for four courses) with sodium thiosulfate rescue and concurrent radiation therapy (70 Gy) for locally advanced head and neck cancer. After treatment, 23% of the ears were recommended to need hearing aids. With multivariate analysis, cumulative dose of cisplatin and radiation, and young age were found to have a causal relationship with increased SNHL during and after therapy. In the multivariate prediction analysis, the pretreatment hearing level of the involved ear was found to be an independent predictive factor for hearing capability after therapy.
Maximum threshold shifts occurred after the second cisplatin infusion, and occurred at 8 kHz. Hearing loss seemed to reach a plateau at higher levels (75 to 80 dB hearing loss) for frequencies greater than compared with frequencies less than 8 kHz (45 to 60 dB hearing loss). Recovery was detected after therapy in 27 ears that had extensive losses at 1 kHz, 2 kHz, and 4 kHz.51
Risk Factors
There seems to be considerable individual variation in susceptibility to cisplatin ototoxicity. The severity of hearing loss seems to be related to the cumulative dose.43 Age seems to be an important risk factor for ototoxicity. Children younger than 5 years and elderly adults are more susceptible to cisplatin-induced hearing loss than younger adults.52,53 Additional factors, such as renal insufficiency and preexisting hearing loss and noise exposure, may increase the probability of cisplatin ototoxicity.37
Genetic Predisposition
Genetic predisposition to cisplatin-induced hearing loss may be related to mitochondrial mutations. Five of 20 cancer survivors with cisplatin ototoxicity were clustered in a rare European J mitochondrial haplogroup that is also associated with Leber’s hereditary optic atrophy.54 Other genetic factors may render patients susceptible to cisplatin ototoxicity. Testicular cancer survivors who received cisplatin chemotherapy showed differences in functional polymorphisms for glutathione-S-transferase. Having both alleles of 105Val-GSTP1 seemed to offer protection against hearing loss from cisplatin. The risk of having a poor hearing result was more than fourfold greater in patients with 105Ile/105Ile-GSTP1 or 105Val/105Ile-GSTP1. Genotypes associated with poorer hearing after cisplatin could indicate patients with a limited amount of glutathione for detoxification of cisplatin.55
Another potential genetic variant that could influence cisplatin susceptibility is megalin. Megalin is a member of the low-density lipoprotein family that is highly expressed in kidney proximal tubular cells and in marginal cells of the inner ear. Marginal cells have been shown in experimental animal studies to accumulate high levels of platinum-DNA adducts.56 Twenty-five patients who developed hearing loss after cisplatin therapy were compared with 25 patients who received cisplatin chemotherapy without hearing loss, and were tested for nonsynonymous single nucleotide polymorphisms of megalin. A higher frequency of the A-allele of rs2075252 was observed in patients with hearing impairment compared with patients with normal hearing after cisplatin treatment. These findings suggest that single nucleotide polymorphisms at the megalin gene may affect individual susceptibility to cisplatin ototoxicity.57
Histopathology
Human temporal bone histopathology studies have shown the effects of cisplatin on the inner ear. The cochlea of a 9-year-old child with a brain tumor who sustained hearing loss after cisplatin showed degeneration of outer hair cells in the lower turns of the cochlea, spiral ganglia, and cochlear nerve. The vestibular ganglion cells and vestibular nerve were reportedly normal.58 Scanning electron microscopic studies of the inner ear tissues of five patients with cisplatin ototoxicity revealed fusion of stereocilia of the outer hair cells, with damage to the cuticular plate.59 Another study of patients receiving cisplatin with or without irradiation revealed a decreased number of inner and outer hair cells and spiral ganglion cells. The stria vascularis was also found to be atrophic.60
Experimental Studies
Histopathologic studies of the inner ear in animals mirror the findings reported in humans. The outer hair cells of the basal turn of the cochlea are damaged first, which extends to the more apical cells when dosing is continued.41,61 The first row of outer hair cells seems to be most vulnerable.41 Damage progresses to the outer hair cells, with dilation of the parietal membrane, softening of the cuticular plate, vacuole formation, and increased number of lysosome-like bodies in the apical portion of the hair cells. Stereocilia abnormalities, including fusion, on inner and outer hair cells are visible.62 Damage to the stria vascularis can also occur, especially after exposure to high-dose cisplatin.63 Electrophysiologic studies have shown that cisplatin ototoxicity can be manifested by a decrease in the endocochlear potential,64 by elevation in auditory brainstem response thresholds,64–67 and by elevations of the distortion product otoacoustic emissions (DPOAE).65,68,69 The DPOAE input/output protocol is most sensitive for early detection of hair cell damage.65 Elevations of DPOAE thresholds 4 days after cisplatin infusion in dogs could not be attributed to differences in peak plasma platinum levels. The variability in susceptibility in individual animals could not be explained by variations in plasma concentration of filterable platinum between animals.69
Mechanisms of Ototoxicity
The ototoxicity of cisplatin seems to be mediated by the production of ROS. Reactive oxygen molecules such as superoxide anion, hydrogen peroxide, and reactive nitrogen species such as nitric oxide can cause cell damage by reacting with cellular lipids, proteins, and DNA. The inner ear has an antioxidant defense system, consisting of the tripeptide glutathione and its related antioxidant enzymes, such as glutathione peroxidase, glutathione reductase, and the other antioxidant enzymes catalase and superoxide dismutase. Glutathione and the antioxidant enzymes have been shown to be decreased in association with cisplatin-induced ototoxicity in the rat.66,67 Cisplatin has been shown to increase the formation of ROS in the cochlea by use of electron paramagnetic resonance spectrometry70 and fluorescent dyes.71 The production of superoxide anions inside the cochlear hair cells has been shown in vitro by use of the nitroblue tetrazolium reduction assay.72 Superoxide dismutates to hydrogen peroxide either spontaneously or by means of superoxide dismutase. Increased hydrogen peroxide formation has been detected in the inner ear after cisplatin exposure.70
One of the enzymes that can produce superoxide radicals is an isoform of nicotinamide adenine dinucleotide phosphate (NADPH) oxidase, NOX-3, which is unique to the cochlea. Cisplatin has been shown to activate this enzyme, leading to a dramatic increase in superoxide production73 not only in cochlear cell lines, but also in the cochlea of rats exposed to cisplatin.74 Superoxide can lead to the formation of hydrogen peroxide, as just shown. The latter can be catalyzed by iron to form the very reactive hydroxyl free radical, which can react with polyunsaturated fatty acids in membranes to form the extremely toxic aldehyde, 4-hydroxynonenal. Superoxide can also react with nitric oxide to form peroxynitrite, which reacts with proteins to form nitrotyrosine. Cochlear hair cells of cisplatin-treated guinea pigs show immunoreactivity for 4-hydroxynonenal, but auditory neurons were found to be immunopositive for 4-hydroxynonenal and nitrotyrosine.75
Because iron chelators have been shown to provide partial protection to hair cells exposed to cisplatin in vitro, it has been proposed that part of the ototoxic mechanism of cisplatin includes an iron-dependent pathway.72 The reaction of ROS with the plasma membrane leads to the formation of membrane lipid peroxidation products such as 4-hydroxynonenal, which are highly reactive and can lead to cellular damage and cell death.76 The primary target of cisplatin ototoxicity seems to be the outer hair cells, with the hair cells in the basal turn being most susceptible. This increased susceptibility may result from the relatively low stores of glutathione in the outer hair cells of the basal turn compared with the inner hair cells and the outer hair cells in the more apical turns.7 The ROS and reactive nitrogen species can attack cellular components as discussed previously, and superoxide anion can react with nitric oxide to form the highly toxic peroxynitrite, which can also attack cellular components.
A partial scheme for the mechanism for cisplatin ototoxicity has been shown in vitro. The reactive molecules, such as superoxide anion, nitric oxide, and others, can activate a cellular protein, p53. This activation can activate enzymes in the cell death pathway, the caspases. Caspase-8 has been shown to be activated, which converts a cellular protein BID from an inactive to an active form, truncated BID. Activated BID acts on a cytosolic protein, BAX, which translocates to the mitochondria. Activated BAX makes the mitochondrial membrane leaky, and the mitochondrial enzyme cytochrome-c leaks out into the cytoplasm. Cytosolic cytochrome-c interacts with another cell death enzyme, caspase-9, which activates caspase-3 and caspase-7, resulting in apoptosis or death of hair cells.77 The application of caspase inhibitors to organ of Corti explants before and during exposure to cisplatin in vitro prevented apoptosis of hair cells.78 This finding provides further evidence that cisplatin ototoxicity may be mediated by the cell death pathways, resulting in hair cell death and permanent hearing loss. These results need to be confirmed by the use of in vivo models of cisplatin ototoxicity. Additional research may provide clinically useful drugs that can block parts of these pathways and protect against ototoxicity of cisplatin.
A more recent study in rats treated with cisplatin confirmed that cisplatin induces an intrinsic apoptotic pathway in the cochlea. Cisplatin significantly increased the levels of caspase-3 and caspase-7 activity, active caspase-3 protein expression, and caspase-9 activity, and increased BAX protein expression accompanied by a decrease in the anti-apoptotic protein Bcl-2 expression in the cochlea. These changes were accompanied by elevation of auditory brainstem response threshold measurements.79
Cisplatin-induced cell death may be independent of p53 and caspases. A more recent study using the OC-k3 cell line exposed to cisplatin suggested that the mitogen-activated protein kinase cascade may be involved in induction of cell death by cisplatin. Cells exposed to cisplatin were found to express an increased phosphorylation of ERK 1/2 that was inhibited by PD98059 and suramin. Both of these inhibitors protected these cells from cisplatin-induced cytotoxicity. Activated ERK 1/2 may be a main effector of cell death, causing nuclear fragmentation, actin cytoskeleton rearrangement, and cell death. Cell death from cisplatin apparently occurred in a p53-independent and caspase-independent manner because these cell lines are functionally depleted of p53.80
Inflammatory cytokines may also play a role in cisplatin ototoxicity. These cytokines (tumor necrosis factor [TNF], interleukin-1β, and interleukin-6) may be up-regulated by activation of ERK and NFκB; this was shown in HEIOC1 cells in vitro. Neutralization of these cytokines by antibodies and pharmacologic inhibition of ERK significantly reduced the death of these cells exposed to cisplatin. These in vitro studies were confirmed in vivo using rats treated with cisplatin. TNF was immunolocalized to the spiral ligament, spiral limbus, and organ of Corti. NFκB protein expression was very strong in cells where TUNEL-positive staining was observed—the organ of Corti, spiral ligament, and stria vascularis. These findings suggest that proinflammatory cytokines, especially TNF-α, play a significant role in cochlear damage caused by cisplatin.81
A more recent review of potential protective agents tested against cisplatin ototoxicity pointed out that no successful clinical trials of protective agents against cisplatin had yet been published. Although numerous successful experiments showed protection in animal models, it is important to try to translate these results to the patients. One concern about the use of systemic protective agents, such as antioxidants, is the possible interference with the therapeutic effect of cisplatin. This problem could be circumvented with intratympanic administration of the protective agent, provided that it diffuses through the round window membrane and penetrates into the inner ear. Positive results with otoprotective regimens in clinical practice may increase the efficacy of cisplatin, and improve the quality of life in survivors of chemotherapy with cisplatin.82
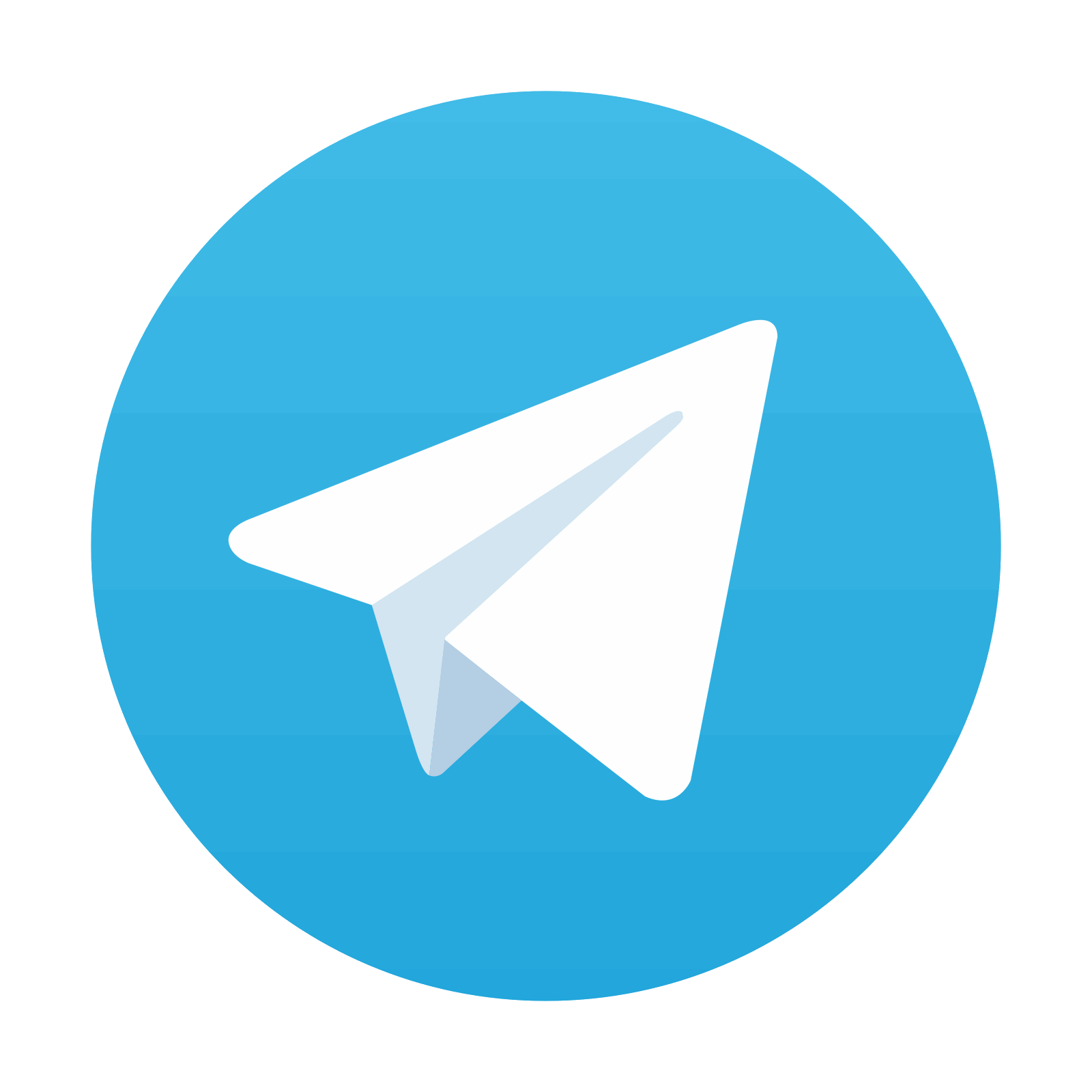
Stay updated, free articles. Join our Telegram channel

Full access? Get Clinical Tree
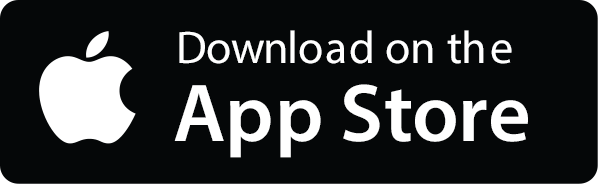
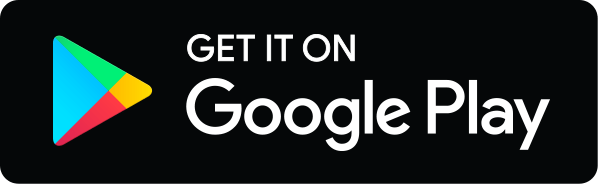