11.1 Introduction
Following cataract surgery, residual lens epithelial cells (LECs) proliferate and migrate from the peripheral posterior capsular bag into the space between the posterior capsule and an optic of the intraocular lens (IOL). These LECs produce posterior capsule opacification (PCO) [1–5], a common complication causing decreased visual acuity after cataract surgery [1, 2]. Patients whose PCO is treated with an Nd: YAG laser to remove the central region of the opacified posterior capsule usually recover their visual acuity, but this procedure may be costly and uncommon, but severe complications such as retinal detachment and secondary glaucoma could occur [1, 2, 6, 7].
The incidence of PCO is thought to be influenced by the design of the IOL such as the shape of the optic edge [8–26], material of the IOL [19–30] such as adhesiveness between the IOL optic and the posterior capsule, which in turn might reflect adhesive properties of IOL materials [13, 31, 32], design of the posterior convexity [33–37], and/or the mechanical force generated by compression of the haptics [38]. Although recent clinical studies indicated that a sharp optic edge plays a key role in preventing PCO irrespective of optic material [8–26], the extent of PCO in eyes implanted with a silicone IOL with a rounded optic edge was less than that with polymethylmethacrylate (PMMA) IOL with a rounded optic edge [17, 28–30]. The extent of PCO in eyes implanted with a hydrophobic acrylic IOL with a sharp optic edge was less than that with a hydrophilic acrylic IOL with a sharp optic edge [19, 20]. These findings suggest that factors in addition to sharpness of the optic edge can influence PCO. Moreover, the shape of optic edge is affected by the design, method, and polishing; the inhibitory effect of optic edge may be different from each other even among groups with sharp optic edge [39, 40]. The degree of pressing the posterior capsule by optic edge may affect PCO although the pressure between the optic edge and posterior capsule may be dependent on design and material of the haptic.
On the other hand, in clinical studies the comparison was performed among IOLs which differs in more than two factors. The silicone IOL with sharp optic edge (CeeOn 911A) prevents PCO more effectively than acrylic IOL with sharp optic edge (AcrySof MA60BM) [41]. However, optic and haptic of CeeOn 911A is made of silicone and polyvinylidene fluoride (PVDF), and AcrySof MA60BM is of acrylic and PMMA, respectively. Moreover, the posterior curvature of two IOLs is different even if the optical power is equal. So it is still unclear whether the difference of PCO among IOLs is dependent on optic material, pressure between posterior capsule and optic edge, and/or the design of the optic edge.
Aspects of LEC migration are difficult to observe in patients by slit lamp examination in vivo since PCO in patients and experimental animals may be influenced by a variety of factors such as age, surgical procedure, and postoperative inflammation [1]. Given the limitations of in vivo studies, we developed a simple in vitro system to facilitate quantitative examination of LEC migration under the IOL optic [13]. To reveal whether the differences of abilities of preventing PCO by optic edge and adhesive properties of IOL are dependent on edge design (sharpness), optic material, or pressure between optic edge and posterior capsule, LECs were cultured with IOLs using a modified in vitro system previously reported [42].
11.2 Methods
11.2.1 Cell Culture
Porcine LECs obtained as described previously [42] were cultured in a humidified atmosphere of 5 % CO2 and 95 % air at 37 °C in MED 10 medium, which consisted of Dulbecco’s Modified Eagle Medium (Life Technologies, Gaithersburg, MD), 10 % fetal bovine serum (FBS; Bioserum, Victoria, Australia), 0.15 % sodium bicarbonate solution (Life Technologies), 50 units/mL penicillin (Life Technologies), and 50 μg/mL streptomycin (Life Technologies). LECs in primary culture were harvested using a solution of trypsin-EDTA (Life Technologies) for use in the following experiments. All procedures were carried out in accordance with the guidelines in the Association for Research in Vision and Ophthalmology Resolution on the Use of Animals in Research.
11.2.2 Assay for Blocking Assessment
Migration of LECs around the IOL optic edge was evaluated in the culture model previously reported [13] with the following modifications (Fig. 11.1). The model consisted of a cell culture chamber insert with a diameter of 10 mm that contained a collagen membrane (Koken collagen CM-24, Funakoshi Inc., Tokyo, Japan). The collagen membrane consisted of aterocollagen that had been extracted from the insoluble collagen of bovine dermis. Substances of low molecular weight such as amino acids and glucose can penetrate this membrane. The insert was placed in a 12-well plate (Falcon, Lincoln Park, NJ) and 1.5 mL of MED 10 was added to the well. A 250-μL volume of MED 10 was added to the insert. The IOL optic, of which haptics were removed, was placed onto the center of the collagen membrane. The IOL optic pressed collagen membrane with the stainless weight, which placed onto the central IOL optic. We used 33, 55, 110, and 260 mg of stainless weight. Small rubber ring was inserted between stainless weight and IOL optic to fix weight. The extent of pressure between collagen membrane and IOL optic depended on the stainless weight. Following incubation of this model in 5 % CO2 at 37 °C for 48 h, 100 μL of a suspension of porcine LECs (15,000 cells/insert) was added to the insert. The IOL optic and stainless weight were removed 48 h after incubation. Migration of the LECs under the IOL optic was observed and photographed.
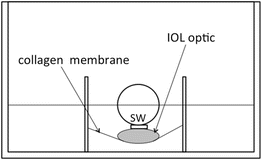
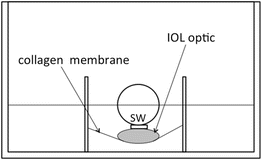
Fig. 11.1
Schematic drawing of the culture system used to evaluate migration of LECs. An insert (10 mm in diameter) containing a collagen membrane was placed at the center of a 12-well plate. The IOL optic pressed upon the membrane with the stainless weight (SW). To fix stainless weight, small rubber ring was inserted between stainless weight and IOL optic
To examine the extent of blocking of LEC migration by the edges of the various IOL optic, we measured and summed the angles at the center of the optic that subtended margin portions where LECs had not migrated at the removal of the IOL optic and stainless weight. The ratio of the sum of these angles to the angle of the entire circumference (360°) was taken as a measure of blocking ability (Fig. 11.2).
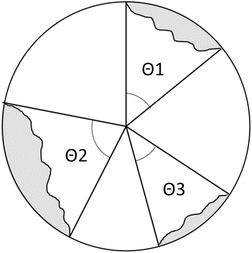
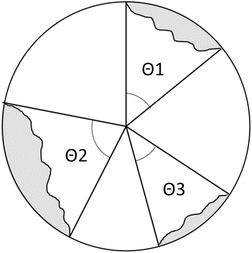
Fig. 11.2
Measurements of angles (θ 1–3) subtending portions of the margin where LECs had not migrated at removal of IOL optic and stainless weight. Blocking ability was defined as the ratio of the sum of these angles to the angle of the entire circumference (360°). The gray zone indicates LECs
The characteristics and physical parameters of the IOLs used in this experiment are shown in Table 11.1. Two types of acrylic IOLs (AR40, AR40e; Abbott Medical Optics, Irvine, CA) have almost the same optic design and material except the shape of optic edge. The AR40e has a sharp optic edge, while the AR40 has a rounded optic edge. The silicone IOL (ClariFlex; Abbott Medical Optics) is the same design of optic as the AR40e. To equalize the area of collagen membrane which was attached to the IOL optic, the posterior curvature of the IOL was arranged. The optic of the IOLs was 6 mm in diameter. The IOLs had a biconvex configuration.
Table 11.1
Characteristics of implanted intraocular lenses (IOLs)
IOL model | AMO Sensar AR40e | AMO Sensar AR40 | AMO ClariFlex |
---|---|---|---|
Optic material | Acrylate copolymer | Acrylate copolymer | Silicone |
Optic diameter (mm) | 6.0 | 6.0 | 6.0 |
Configuration | Biconvex | Biconvex | Biconvex |
Power (diopters) | +17.0 | +17.0 | +17.0 |
Radius of curvature of posterior surface (mm) | 15.7 | 15.5 | 15.7 |
Weight (mg) | 25.1 | 22.4 | 25.1 |
11.2.3 Assay for Invasion Assessment
To evaluate migration of LECs on the membrane under the IOL optic, the culture model described above was modified as follows (Fig. 11.3). The silicone rubber ring, used as a pedestal, had an inner diameter of 1.9 mm and an outer diameter of 6.6 mm. After the silicone rings were placed in the centers of wells in a 12-well plate, 1.5 mL of MED 10 was added to the well. The insert was placed upon the silicone ring, which caused elevation of the central collagen membrane in the insert. A 200-μL volume of MED 10 was added to the insert. After removal of the haptics, the IOL optic was placed upon the collagen membrane elevated by the silicone ring. The periphery of the IOL optic was not attached to the collagen membrane, since the area elevated by the silicone ring was smaller than the area of the posterior IOL optic. The IOL optic was pressed by a stainless steel weight as mentioned above. As a result, in this culture model, the collagen membrane of the insert was sandwiched between the silicone ring and the IOL optic. The degree of pressure between the collagen membrane and the IOL optic depended on the weight applied. Following incubation of this model in 5 % CO2 at 37 °C for 48 h, 100 μL of a suspension of porcine LECs (15,000 cells/insert) was added to the insert; then, the LECs were cultured for 48 h.
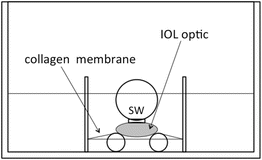
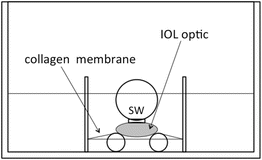
Fig. 11.3
Schematic drawing of the culture system used to evaluate migration of LECs. A silicone ring (SR) was placed at the center of a 12-well plate. An insert (10 mm in diameter) containing a collagen membrane was placed on the silicone ring, which elevated the central collagen membrane of the insert. The IOL optic was pressed to the membrane by a stainless steel weight (SW). To fix the weight in place, a small rubber ring was inserted between the stainless steel weight and the IOL optic
To determine a weight sufficient to almost completely prevent LEC migration onto the collagen membrane under the IOL optic, culture was stopped in some wells where the IOL and the stainless steel weight were removed and migration of the LECs under the IOL optic was observed and photographed. We experimented with 55-, 110-, 260-, and 510-mg stainless steel weights. The cell-free area where the LECs had failed to migrate onto collagen membrane beneath the IOL optic was measured.
To examine whether adhesive properties of IOL materials influenced migration of LECs on collagen membrane under the IOL optic, LECs were cultured for another 48 h after the weights were removed. If the IOL optic possessed an adhesive property with respect to the collagen membrane, the IOL optic could remain attached to the collagen membrane after weight removal and continue to inhibit LEC migration into the space between the collagen membrane and the IOL optic. After incubation, IOL optics were removed, and migration of LECs under the IOL optic was observed and photographed. Cell-free areas 48 h after weight removal also were measured to determine the area representing LEC migration during the 48 h after weight removal. The cell-free area 48 h after the weight removal was subtracted from the area immediately after the removal. The ratio of the cell-migration area to the cell-free area immediately after weight removal (migration ratio) was determined and was used to assess the extent of LEC migration under each IOL optic.
Characteristics and physical parameters of the IOLs used in this study are shown in Table 11.2. The posterior curvature of the IOL was opposed to the membrane with care to equalize the area of collagen membrane attached to the IOL optic. Each IOL optic was 6 mm in diameter. IOLs had a biconvex configuration. Although one type of silicone IOL (SI-40NB; Abbott Medical Optics, Irvine, CA) had an optic consisting of an optical zone and a peripheral rim, the area of collagen membrane attached centrally to the IOL optic was smaller than the optical zone, so the peripheral rim did not affect the experiments.
Table 11.2
Characteristics of implanted intraocular lenses (IOLs)
IOL model | Alcon AcrySof MA60BM | HOYA AF-1 VA60BB | AMO Sensar AR40e | AMO PhacoFlex II SI-40 | Pfizer CeeOn 911A | NIDEK NP-74D |
---|---|---|---|---|---|---|
Optic material | Acrylate copolymer | Acrylate copolymer | Acrylate copolymer | Silicone | Silicone | PMMA |
Optic diameter (mm) | 6.0 | 6.0 | 6.0 | 6.0 | 6.0 | 6.0 |
Configuration | Biconvex | Biconvex | Biconvex | Biconvex | Biconvex | Biconvex |
Power (diopters) | +20.5 | +29.5 | +17.0 | +17.0 | +15.5 | +20.0 |
Radius of curvature of posterior surface (mm) | 15.8 | 15.7 | 15.7 | 15.5 | 15.7 | 15.6 |
Weight (mg) | 20.5 | 21.6 | 22.3 | 20.8 | 24.1 | 21.5 |
11.2.4 Adhesion Property
Adhesion between the collagen membrane and the IOL optic was measured directly with a tensiometer (Instron mini-44 tensiometer; Instron, Canton, MA). The above culture model was mounted on a tensiometer and suspended in a balanced salt solution (BSS plus, Alcon, TX) at 37 °C. The IOL optics without haptics were attached to the plunger of the tensiometer. The plunger descended at 1 mm/min until the pressure between the IOL optic and the collagen membrane reached 15.3 g. After this pressure was maintained for 1 min, the plunger was raised at 10 mm/min until the pressure between the IOL optic and collagen membrane fell to zero. The time course of the tension was recorded automatically (Scope Corder DL750; Yokogawa, Tokyo, Japan). Maximum tension was measured.
11.2.5 Statistical Analysis
Data are presented as the mean ± SD. Differences with respect to data were evaluated by one-way or two-way analysis of variance (ANOVA) and Fisher’s protected least significant difference test. Simple regression analysis was used to evaluate the relationship between the stainless weight and the blocking ability. Spearman’s rank correlation coefficient was used to evaluate the relationship between migration ratio and maximum tension. A value of P < 0.05 was accepted as indicating statistical significance.
11.3 Results
11.3.1 Blocking Ability of IOL Optic Edge
Within 48 h after plating, LECs migrated into the space between the collagen membrane and the IOL optic except when the stainless weight was 260 mg. When the stainless weight was 260 mg, the LEC migration under IOL optic was completely inhibited regardless of IOL type and the blocking ability of each IOL reached to 100 %. However, it was unclear whether the blocking ability of each IOL reached the maximum (100 %) using stainless weight less than 260 mg (Table 11.3). To evaluate the relationship between the stainless weight and blocking ability of each IOL, the data of blocking ability using the stainless weight less than 260 mg, which did not reach to the maximum (100 %), were used.
Table 11.3
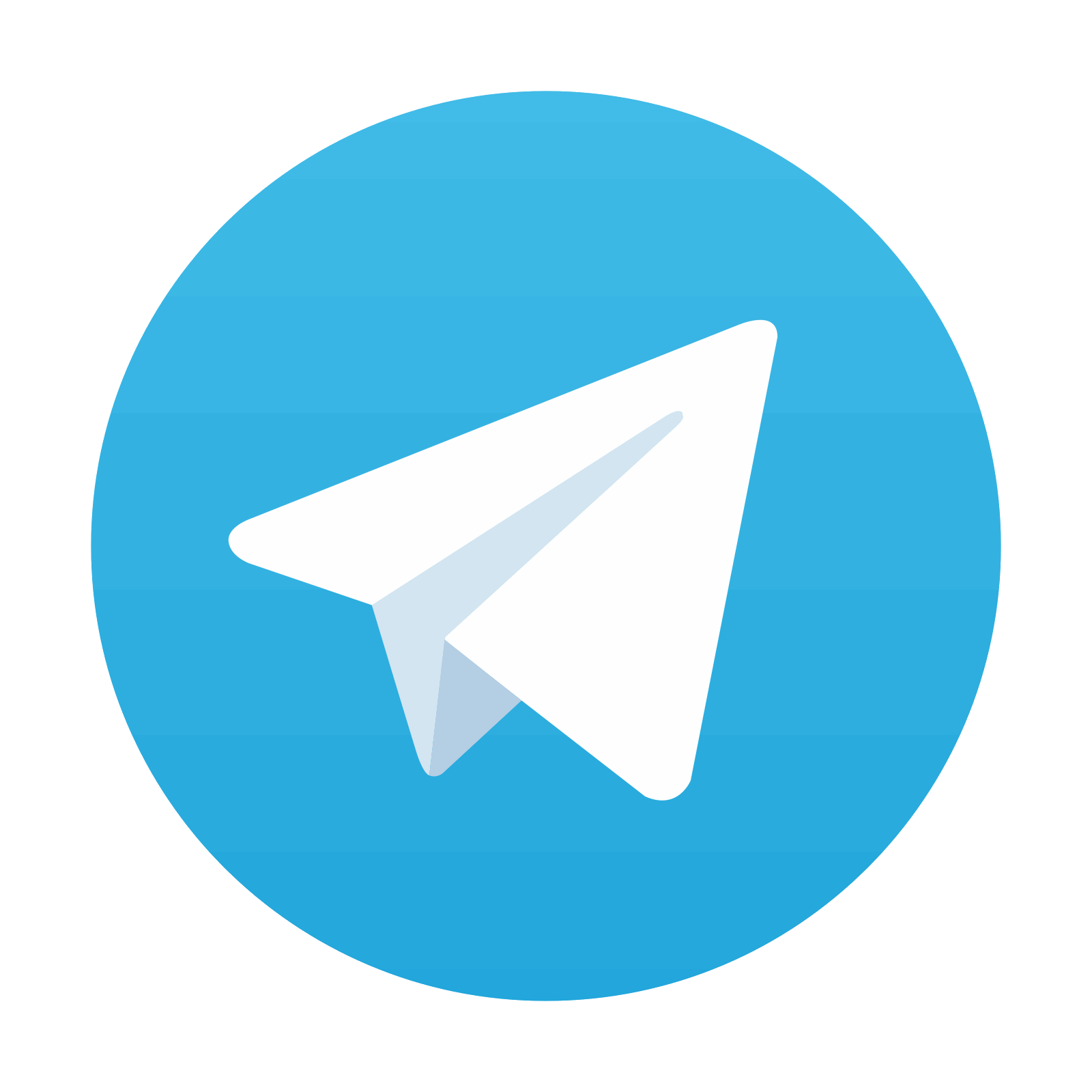
Relationship between blocking ability of IOL and stainless weight
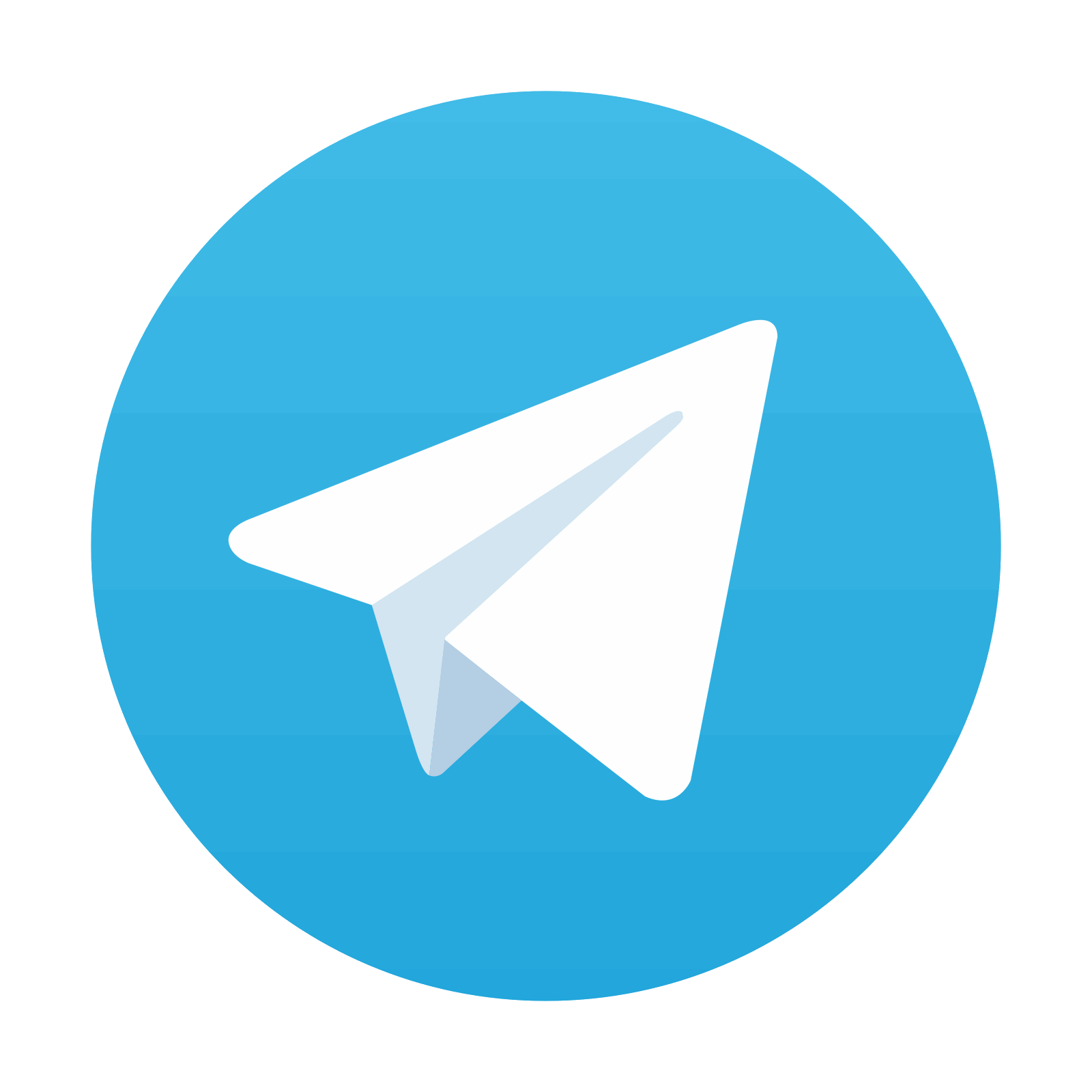
Stay updated, free articles. Join our Telegram channel

Full access? Get Clinical Tree
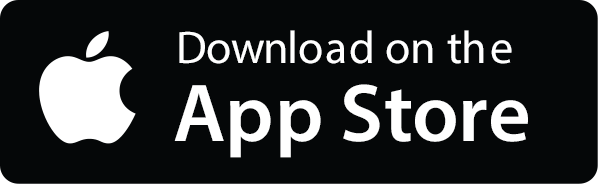
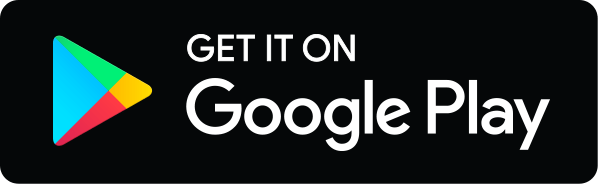
