Fig. 1.1
Examination of the eyeball. (a) Note the extra-scleral extension of a malignant choroidal melanoma along the vessels (arrow). (b) Histological survey of a similar area
Internal tumours are looked for by transillumination. This is best performed with a fibre optic source in dimmed light (Fig. 1.2). Draw the outlines of the shadow with a tissue pencil upon the sclera to facilitate correct cutting of the globe.
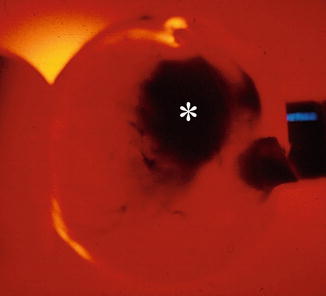
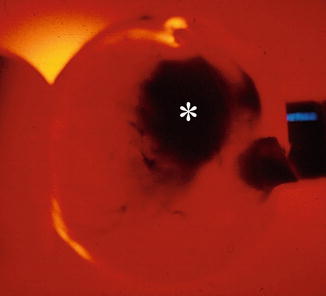
Fig. 1.2
Diascleral illumination of an eye with a malignant choroidal melanoma. The localisation and size of the tumour is determined from its shadow (asterisk)
The globe is by standard cut into three: two calottes and a central ring. Use disposable blades; those used for cleaning glass windows for sticky paper are excellent (Fig. 1.3). If no intraocular tumours are suspected, the globe is divided by horizontal cuts. The two cuts are placed with a distance of app. 8 mm to create a ring that includes the cornea, the sclera, the anterior chamber, the optic nerve, the fovea, and the lens.
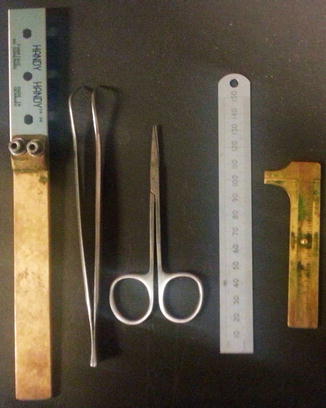
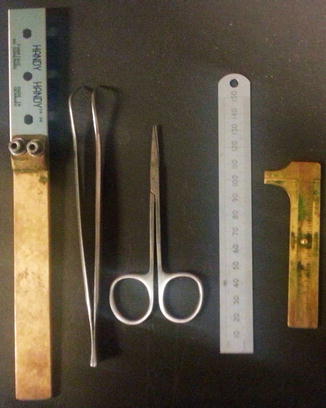
Fig. 1.3
Tools for sectioning of the eyeball
Embedding in paraffin follows routine protocols; however, since the specimen is a ring with a stiff outer wall (the sclera) and a thin friable inner wallpaper (the choroid and the retina), only supported by a collapsed vitreous body (age or the fixative), most types of paraffin tend not to prevent artificial detachment of the retina during processing and cutting. Admixture of 7 % ordinary bee wax to the paraffin is a good solution to this problem.
Photographic documentation is performed in a photomicroscope equipped with focal light sources. Submersing of the central ring in water is recommendable to prevent multiple reflexes.
1.1.3 The Ordinary Sequence in Which to Describe the Eye in Histopathology
By tradition, a sectioned eye is described in a specific sequence: cornea, limbal tissue, sclera, anterior chamber, iris, ciliary body, choroid, retina, optic nerve, optic disc, vitreous body and lens.
1.2 Embryology, Anatomy and Development
1.2.1 Embryology
The embryology of the eye has been splendidly described in classic textbooks [3] and in a series of more recent papers [4–7]. The embryonic development of the eye can be observed 3 weeks after conception when the optic sulci appear in the neural folds. The genesis of the eye is characterised, on the molecular level, by the expression of “eye-field transcription factors”, regulating the development of the eye structures from the neuroectoderm, the surface ectoderm and the mesenchyme. When the neural tube closes, optic sulci deepen and become optic vesicles, arising as hemispherical extensions on the lateral sides of the forebrain vesicle and in continuity with the neural tube that is composed of neuroectodermal cells. At the end of the fourth week, the optic vesicles approach the surface ectoderm, inducing the formation of the lens placodes which invaginate, creating the two lens vesicles. Concurrently, the growth of the cells of the optic vesicle leads to infolding of the anterior part of the vesicle into the posterior part, forming the optic cup (Fig. 1.4).
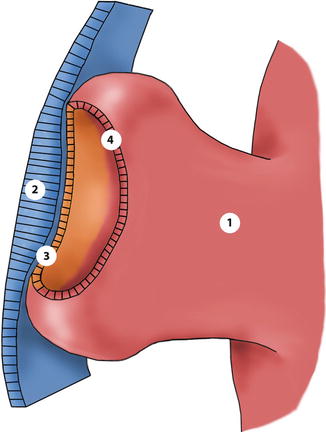
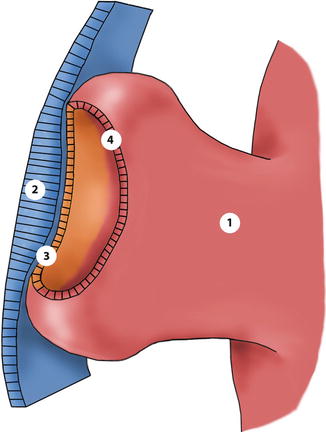
Fig. 1.4
Drawing of the optic vesicle. The vesicles evaginate from the anterior neural tube during the second foetal week. 1 optic vesicle, 2 lens placode, 3 future anterior iris epithelium + non-pigmented ciliary epithelium + neuroretina, 4 future anterior iris epithelium + outer ciliary epithelium + retinal pigment epithelium
During the second month the inner layer of the optic cup differentiates into the posterior iris epithelium, the inner ciliary epithelium and the neurosensory retina. The outer layer develops into the anterior iris epithelium and the iris muscles, the outer ciliary epithelium and the retinal pigment epithelium (RPE). The cup is incomplete inferiorly. This cleft is called the embryonic fissure, and it extends from near the lens to the optic stalk and allows vessels of the hyaloid system to get into the eye (Fig. 1.5). Subsequently, the two edges of the fissure fuse.
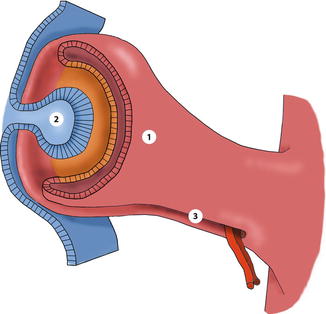
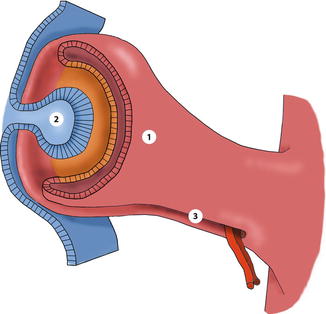
Fig. 1.5
At 4 weeks the optic cup is created by invagination of optic vesicle. Evagination of lens placode forms the lens vesicle. 1 outer layer of optic cup, 2 lens vesicle, 3 hyaloid artery enters through the open embryonic fissure
Differentiation of the neurosensory retina begins with three to four rows of cells with high mitotic activity. The inner third is called inner marginal zone and differentiates into the nerve fibre layer. Neural and glial cells keep evolving simultaneously and develop into the inner and outer neuroblastic layers. They are separated by the transient nerve fibre layer of Chievitz, which around 10–12 weeks becomes the inner plexiform layer, except in the macule where it remains until birth. By the end of the third month, the four major horizontal layers of the retina are developed. Between the third and fifth month, the ganglion cells are the first to differentiate and axons enter the optic stalk, inducing the formation of the optic nerve. Photoreceptors arise from the outermost layer of neuroblastic cells, and the differentiation of cones starts in the future foveal area. The differentiation of cone outer segments begins at the fifth month, and the rod outer segments develop during the seventh month when also bipolar cells develop. The development of the retina originates from the posterior area, and prearrangement of foveal organisation starts early because it is the central point from where cells extend peripherally. The foveal pit is recognisable by the seventh month due to the thinning of the inner nuclear layer. By the eighth month, the ganglion cell layers have decreased to two layers, and the inner nuclear layer is reduced to three rows of cells or less because of a lateral displacement of the remaining layers. At birth, the fovea still contains the transient layer of Chievitz, and its maturation to form the foveola begins at 4 months after birth; the remodelling continues until 4 years of age.
Retinal pigment epithelium develops from the outer layer of the optic cup, and at week 6 melanogenesis begins. The RPE are the first cells in the body to produce melanin. The RPE cells turn into cuboidal and cylindrical cells by the fourth month, and RPE is supposed to be functional at this time.
A primary vitreous is established during the second month, when mesenchymal cells and the hyaloid system vessels enter the embryonic fissure. Subsequently, as the secondary vitreous develops and is composed of collagen fibrils and hyalocytes, the primary vitreous and its hyaloid system regress, leaving a remnant postnatal channel: the Cloquet’s canal. The condensation of collagen fibrils around the lens equator and the outer vitreous constitutes the final tertiary vitreous (Fig. 1.6).
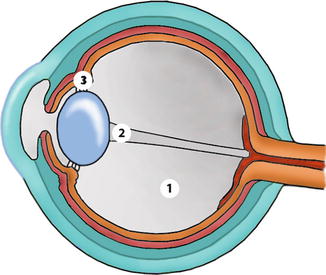
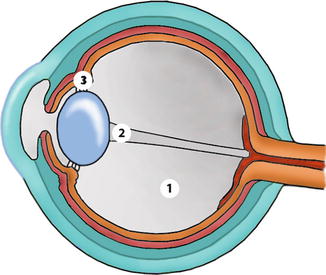
Fig. 1.6
At 9 weeks most structures of the future mature eye can be identified. 1 secondary vitreous, 2 canal of Cloquet, 3 tertiary vitreous
The optic nerve develops from the optic stalk, the connection between the forebrain and the optic vesicle. By the seventh week, the optic disc comprises the hyaloid artery surrounded by axons derived from the ganglion cells. The number of axons rises rapidly reaching the adult level around the 34th week. At birth 75 % of the growth of the optic nerve and disc has developed and 95 % is reached before 1 year of age.
The lens arises from the lens placode (surface ectoderm) under the induction from the underlying optic vesicle. Pax6 acts in this phase as the master control gene, and genes encoding cytoskeletal proteins, structural proteins and membrane proteins become activated (Fig. 1.5). Invagination of the lens placode leads to the formation of a lens vesicle, and the basal lamina of the epithelial cells makes up the lens capsule. The posterior row of cells stops to divide but elongate to reach the anterior part of the cells. Thereby the primary lens fibres and the embryonic nucleus are formed by the end of the second month. Pre-equatorial cells preserve the mitotic activity and produce the secondary lens fibres that are joined centrally in the shape of a Y anteriorly and as an inverted Y posteriorly. The cells in the anterior part of vesicle remain cuboidal and continue to divide through life. The zonules form from the tertiary vitreous and reach the lens by the fifth month.
The cornea develops from the surface ectoderm, when the lens vesicle move into the optic cup, and neural crest-derived cells from the periocular mesenchyme migrate in front of it and into the later cornea. Ectodermal cells form the epithelium. The neural crest-derived mesenchymal cells (mesectodermal cells) become keratocytes that produce collagen fibrils and matrix, thus creating the corneal stroma. The mesectodermal cells also form the corneal endothelium, which becomes a single layer of cells by the third month. The endothelial basement membrane produces the foetal Descemet’s membrane.
The sclera derives also from the mesectodermal cells around the optic cup; posteriorly these cells migrate into the nerve fibres in the optic nerve to form the cribriform layer.
The choroid arises very early in the development from mesectodermal cells around the optic cup. They differentiate into the choroidal stroma, which initially is composed of a framework of collagen fibrils and fibroblasts. Melanocytes appear by the sixth month. A palisade of vessels form externally to the RPE, and the choriocapillaris begins to differentiate. They develop communications with the precursors of ciliary arteries by the second month. In the third month, an outer layer of large vessels (Haller’s layer) develops, and along the fourth month, the anterior ciliary and long posterior ciliary arteries form the major arterial circle of the iris. The third layer of medium-sized vessels (Sattler’s layer) develops at sixth month.
Ciliary body and iris development commences at the second month with the indentation of the outer pigmented layer of neuroectoderm (future pigmented ciliary epithelium) by small capillaries in the inner vascular mesenchyme. The vascular young branch, covered by a double layer of epithelial cells, enlarges and forms primitive radial folds around the lens, being the basis of the ciliary processes. The ciliary muscle differentiates between the fourth and fifth month from mesenchymal cells between the primitive ciliary epithelium and the anterior sclera. Iris development is related to the formation of a tunica vasculosa lentis. During thethird month, there is an expansion of neuroepithelial cells at the cup anterior margin to the primitive ciliary body, growing centripetally between the primitive cornea and the lens surface, incorporating vessels from the pupillary membrane, lying on the anterior surface of the lens. The vascular mesenchyme forms the future iris stroma. Pigmentation of the posterior epithelial layer, which is a continuation of the non-pigmented layer of the ciliary body and neurosensory retina, is completed by the end of the seventh month. Sphincter muscle differentiation from the anterior layer of epithelium starts at 3 months, but complete development finishes at the 8th month. Dilator muscle appears at sixth month as basal extensions of the anterior pigmented iris epithelium, and differentiation continues after birth as well as the full development and pigmentation of stroma.
1.2.2 Anatomy
The eye is a specialised organ in the transformation of light energy in the retina into neural potentials transmitted through the optic tract to the brain, where the information is processed as vision. Splendid surveys of the anatomy may be consulted [8, 9]. The eyeball or globe is an almost spherical structure with an anterior-posterior diameter in the adult of about 23–25 mm and 24 mm in transverse diameter. The eye is composed of three concentric layers: the external or fibrous (cornea and sclera), the middle or uvea (iris, ciliary body and choroid) and the inner or neural layer (retina and optic nerve). It contains also three compartments (anterior chamber, posterior chamber and vitreous cavity), and these chambers are filled with aqueous humour and the posterior cavity with the vitreous gel (Figs. 1.7 and 1.8).
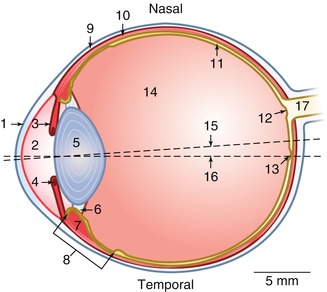
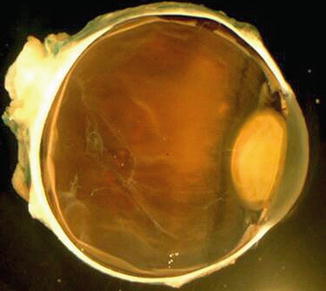
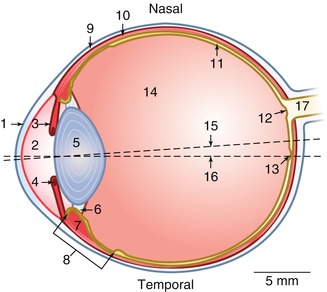
Fig. 1.7
Dimensions and details of a human eye. 1 cornea, 2 anterior chamber, 3 iris, 4 pupillary sphincter muscle, 5 lens, 6 zonules, 7 m. ciliaris, 8 ciliary body, 9 sclera, 10 choroid, 11 retina, 12 optic disc, 13 foveola, 14 vitreous body, 15–16 visual axis, 17 optic nerve
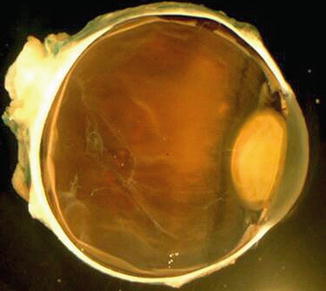
Fig. 1.8
Horizontal section of a human eye
1.2.3 External Layer
The external layer of the eyeball consists of the transparent cornea anteriorly (1/6) and the opaque sclera posteriorly (5/6). The transitional zone between the two is the corneal limbus. The external layer is composed of connective tissue with refractive (cornea) and protective functions.
The cornea is a transparent, nearly spherical structure, located in the centre of the anterior pole of the globe and continuous with the sclera at the limbus. In the adult, it measures 12 mm in the horizontal meridian and 11 mm in the vertical, being almost 1 mm thick in the periphery and 0.5 mm centrally. Corneal transparency is due to several characteristics: regularity of the epithelium, avascularity, regular arrangement of stromal components and relative dehydration. Its optical function is important being a positive lens of 43 diopters, i.e. the main refractive element of the eye. The exposed surface of the cornea is covered by the tear film, which is essential for normal corneal function. It lubricates and produces a smooth optical surface and provides nutrients and other components necessary for the maintenance of the ocular surface. The cornea is composed of five layers (Fig. 1.9).
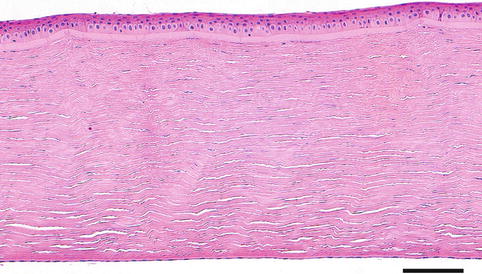
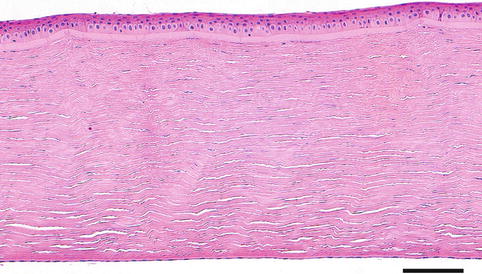
Fig. 1.9
The normal cornea is composed of five layers, i.e. the epithelium, Bowman’s layer, the stroma, Descemet’s membrane and the endothelium. H&E stain, bar = 100 μm
The corneal epithelium is a stratified squamous non-keratinised epithelium of five or six layers attached to the underlying basement membrane by hemidesmosomes. It has a basal layer of columnar cells, then two or three layers of polygonal “wing cells”, and an outermost superficial layer of cells that are flattened and thin. New cells are derived from mitotic activity in the basal-peripheral cell layer. The new cells float towards the centre and displace older cells superficially and centripetally. Non-epithelial cells may appear as wandering histiocytes, macrophages, lymphocytes and antigen-presenting Langerhans cells.
The Bowman’s membrane or layer is considered a modified region of the stroma and consists of a uniform acellular zone below the epithelial basement membrane, tightly bound to the corneal stroma. It is composed of small collagen fibres and has pores for the terminal branches of corneal nerves, which continue into the basal layers of the epithelial cells. Bowman’s membrane is not regenerated after injury.
The stroma constitutes 90 % of the corneal thickness and is a dense connective tissue of extraordinary regularity and consists of collagenous lamellas oriented parallel to the corneal surface, with modified flattened fibroblast containing crystallines, termed keratocytes. The transparency of the cornea is highly dependent on the arrangement of the collagen fibrils, which is regulated by glycosaminoglycans and proteoglycans. The stroma is avascular but sensory nerve fibres are present in the anterior layers to reach the epithelium.
Descemet’s membrane is the basal lamina of the corneal endothelium, and its thickness increases with age. It is composed of the anterior zone band, developed before birth, and the posterior non-banded zone formed by corneal endothelium. The non-banded zone grows through the entire life of the person.
A separate structure the Dua layer has recently been suggested to exist [10]. The layer is ascribed to the cleavage site when performing Descemet’s membrane pealing. It is an acellular structure found beyond the last row of keratocytes, measuring 10 μm in thickness, and is composed of five to eight lamellas of predominantly type I collagen bundles arranged in transverse, longitudinal and oblique directions. Its recognition has not yet been confirmed by other authors and it cannot be identified by ordinary histology.
The corneal endothelium is a monolayer of hexagonal cells derived from the neural crest; they contain large nucleus and abundant mitochondria. The main function of the endothelium is to maintain corneal dehydration and transparency. The cells have a low regenerative capacity and decrease in number with age. Lost cells are replaced by spreading of the adjacent cells. Endothelial dysfunction leads to oedema of the stroma and loss of transparency.
The sclera, in contrast to the cornea, is opaque and has an anterior opening for the cornea and a posterior for the optic nerve, crossed by lamina cribrosa. It consists of bundles of collagen, fibroblasts and ground substance. It is essentially avascular except anteriorly where it is covered by episclera and is crossed by numerous emissary channels through which pass arteries, veins or nerves. The four rectus muscles insert anteriorly around the limbus forming the spiral of Tillaux. The medial rectus muscle inserts closer to the limbus (5.5 mm) and the superior muscle the farthest (7.7 mm). The equator of the eyeball is approximately 16 mm behind the limbus (Fig. 1.10). Posteriorly, the insertions of oblique muscles in the temporal halves and the vortex veins in the four quadrants are found. The short posterior ciliary arteries and nerves enter around the optic nerve. Two long ciliary nerves and arteries enter at the horizontal meridian on each side of the optic nerve and advance through the suprachoroidal space to the ciliary body. The optic channel is crossed by a fenestrated plate, the lamina cribrosa, where the axons from the ganglion cells meet to exit the eye forming the optic nerve.
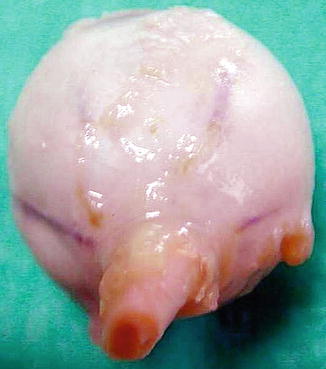
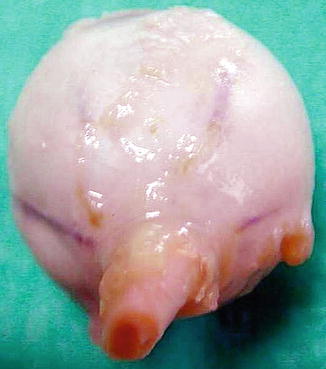
Fig. 1.10
An enucleated eye seen from behind. Note the emissaries as bluish structures
The limbus is the transitional zone between the transparent cornea and the opaque sclera, relevant due to the relation with the anterior chamber angle. At this location, the corneal epithelium is continued into the conjunctival epithelium, increasing the number of cell layers and with the appearance of a sub-conjunctival tissue containing vessels and progenitor stem cells, responsible for the turnover of corneal epithelial cells. Bowman’s membrane ends, and the transparent corneal stroma continues with the opaque sclera, drawing a concavity called external scleral sulcus. Deep in the limbus, the Descemet’s membrane ends in the Schwalbe’s line where the anterior chamber angle begins (Fig. 1.11).
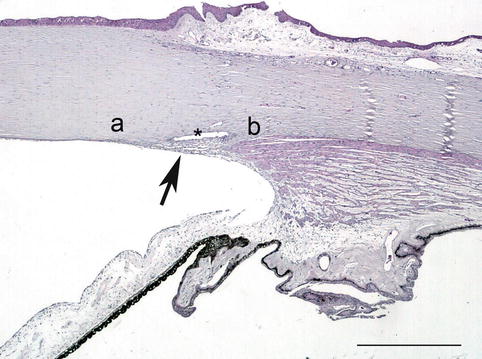
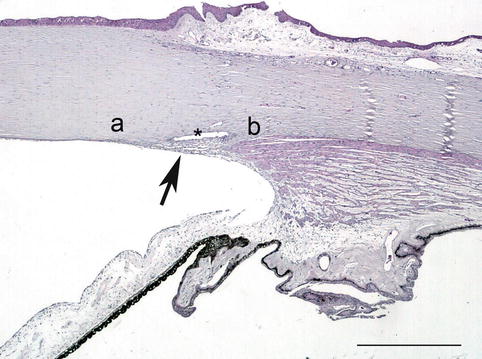
Fig. 1.11
The limbal area and the chamber angel. The trabecular meshwork (arrow) is located between the Schwalbe’s line (a) and the scleral spur (b) and makes up the internal wall of the Schlemm’s canal (asterisk). H&E stain, bar = 200 μm
The anterior chamber angle is situated at the confluence of the cornea, sclera and iris and is limited anteriorly by Schwalbe’s line and posteriorly by the scleral spur (Fig. 1.11). The inner sclera forms the internal scleral sulcus containing the trabecular meshwork and the Schlemm’s canal, the main structures in the drainage of the aqueous humour. The longitudinal ciliary muscle fibre inserts in the scleral spur and myofibroblast-like cells are found circumferentially.
1.2.4 Middle Layer
The uveal tract is the middle vascular pigmented layer of the eye which consists of the choroid, ciliary body and iris. These constituents are continuous and have an opening anteriorly, the pupil, and posteriorly they surround the optic nerve channel. The uvea is responsible for the nutrition of most intraocular components, but the passage of nutrients into the eye is controlled by vascular barriers. In the iris, the capillary endothelium is non-fenestrated; in the ciliary body, the blood-aqueous barrier; and in the retina, the outer blood-retina barrier. The uvea is attached firmly to the sclera in three locations only: at the scleral spur, at the exits of the vortex veins and at the optic nerve.
The iris is the anterior part of the uvea and has a central hole, the pupil. The iris stroma adapts to its variable size being loose and mobile, presenting folds during mydriasis and being relatively smooth in miosis. It is the border between the anterior and the posterior chambers. The iris is composed of the anterior surface, the mesectodermal stroma and the two posterior, pigmented epithelia (Fig. 1.12). The anterior surface is an accumulation of stromal components, avascular and without continuous cell coverage, characteristically irregular with ridges and folds. The stroma is composed of connective tissue and pigmented and non-pigmented melanocytes whose density of pigmentation is responsible for the iris colour. The stroma near the pupil contains the sphincter muscle, a circular band of smooth muscle fibres around the pupillary margin (Fig. 1.13). Blood vessels follow a radial course from the major arterial circle towards the pupil forming the minor vascular circle of the iris, usually incomplete. The anterior epithelial layer emits non-pigmented cytoplasmic extensions with myofibrils constituting the iris dilator muscle. The posterior iris pigment epithelium is heavily pigmented. The two iris epithelia continue uninterruptedly with the ciliary epithelia.
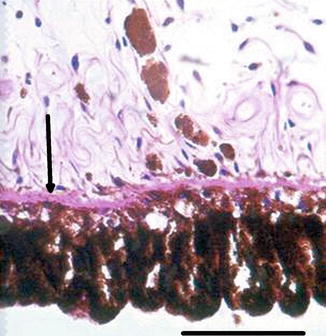
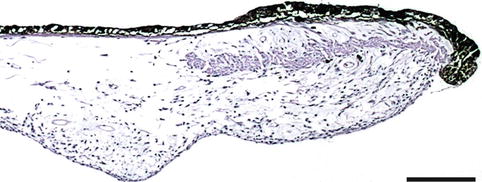
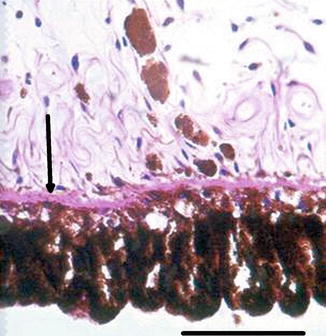
Fig. 1.12
The two pigmented epithelial layers of the iris. Arrow at dilatator muscle within the anterior epithelial layer. H&E stain, bar = 50 μm
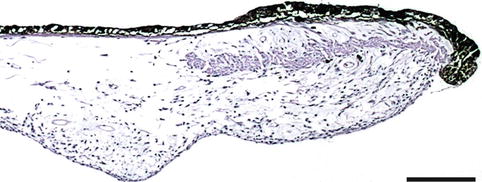
Fig. 1.13
Pupillary margin. Note the sphincter muscle in the stroma near the pupil. H&E stain, bar = 100 μm
The ciliary body has a triangular shape with the base in the iris and the vertex to the choroid. Its main functions are aqueous humour formation and lens accommodation. It is consists of a pars plicata and a pars plana (Fig. 1.14). The pars plicata is composed of approximately 70 radial folds, the ciliary processes, consisting of a vascular connective axis covered by a double epithelial layer (pigmented and non-pigmented) which continues uninterruptedly with the epithelium of the iris and the pars plana (Fig. 1.15).
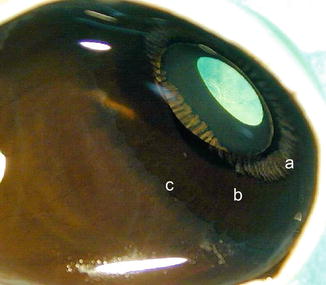
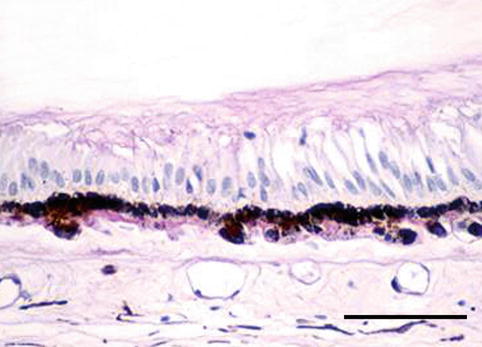
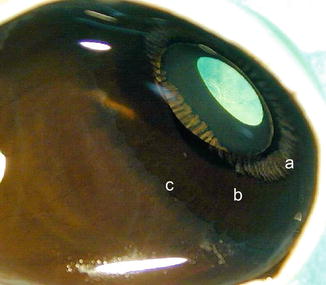
Fig. 1.14
View of ciliary body from behind. Pars plicata (a) has a folded structure, pars plana is flat (b) and ora serrata (c) is the border towards the retina
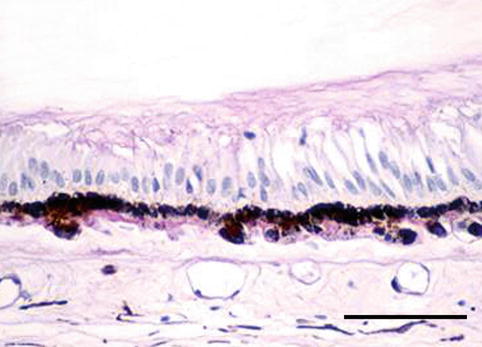
Fig. 1.15
Ora serrata. The non-pigmented ciliary epithelium transform to elongated cells that more posteriorly continues into the neuroretina. H&E, bar = 100 μm
The epithelial cells are joined by tight junctions forming a barrier to the passage of blood products to the posterior chamber, thus constituting the blood-aqueous barrier. In the depth of the pars plicata is the ciliary muscle with three layers: the outer-longitudinal, the middle-radial and the inner-circular muscles functioning as one unit. The zonules of the lens are attached to the ciliary processes. Contraction of the ciliary muscle relaxes the tensile force of the zonules, allowing accommodation of the lens for near vision. The pars plana consists of a thin layer of connective tissue covered by two separate epithelial cell layers, continuous with those of the pars plicata. The inner non-pigmented epithelium is pseudostratified and is strongly linked to the vitreous. The point where the non-pigmented inner layer becomes a multilayer marks the beginning of the neurosensory retina and is known as the ora serrata, with a characteristic anatomical and clinical morphology recognisable at clinical examinations (Fig. 1.15).
The choroid is the posterior portion of the uvea, and its main function is to nourish the outer portion of the retina and to keep the temperature of the retina within normal metabolic range – the photosensory mechanisms and the degradation of photons within the retina create immense heat. The choroid is composed of vessels, connective tissue and melanocytes. Perfusion comes from the long and short ciliary arteries and from retrograde branches from the perforating anterior ciliary arteries in the periphery. It consists of three layers: externally large vessels, at the middle small vessels and at the innermost the choriocapillaris, with its tightly packed capillaries containing multiple fenestrations facing the retina. Bruch’s membrane is a membrane resulting from the fusion of the basement membranes of the retinal pigment epithelium and the choriocapillaris. It is highly permeable to small molecules, but the tight junctions between the RPE cells prevent the free passage of substances into the retina, i.e. the outer blood-retina barrier (Fig. 1.16).
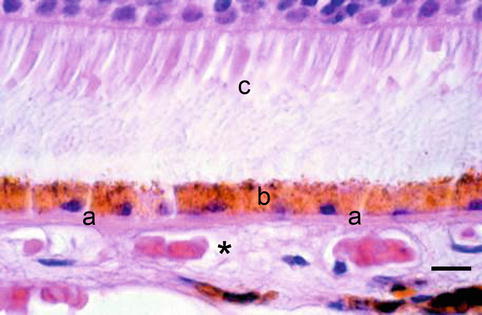
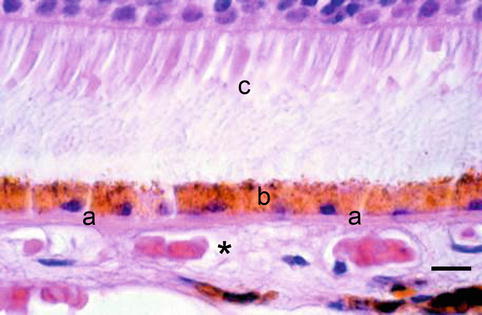
Fig. 1.16
Choriocapillaris (asterisk) is situated outside the Bruch’s membrane (a), the retinal pigment epithelium (b) and the photoreceptor outer segments (c). H&E, bar = 10 μm
1.2.5 Inner Layer
The retina is the innermost layer of the eye. It is the focus for the eye’s optical system and contains photoreceptors and neural elements in which photons are transformed to visual neural impulses that are transmitted to the brain. Branches from the central retinal artery vessels enter through the optic nerve and divide in nasal, temporal, superior and inferior branches. The blood vessels are similar to the cerebral blood vessels, composed of non-fenestrated endothelial cells with tight junctions, constituting the inner blood-retina barrier.
The retina is composed of the outer RPE and the inner neurosensory retina with nine different layers (Figs. 1.17 and 1.18).
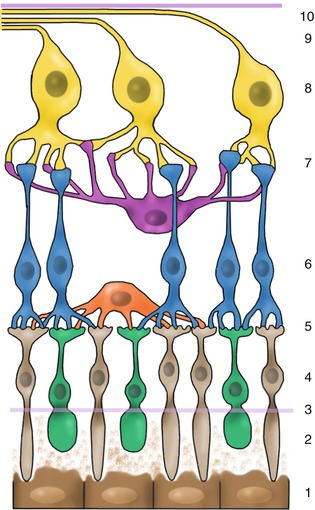
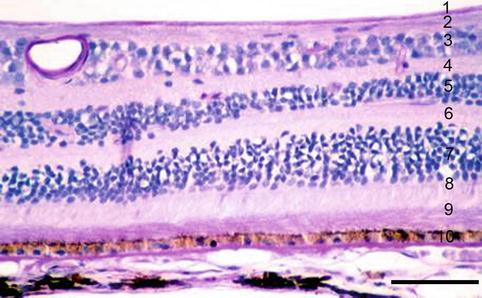
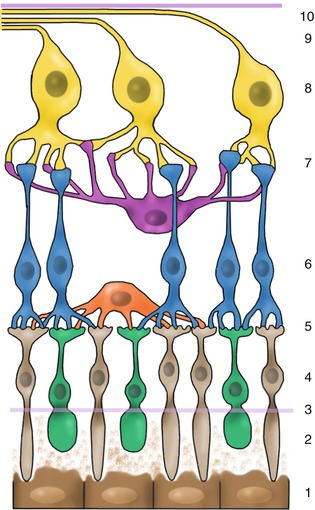
Fig. 1.17
Drawing of retina with major structures. 1 retinal pigment epithelium, 2 photoreceptor outer segments, 3 external limiting membrane, 4 outer nuclear layer, 5 outer plexiform layer, 6 inner nuclear layer, 7 inner plexiform layer, 8 ganglion cell layer, 9 nerve fibre layer, 10 internal limiting membrane
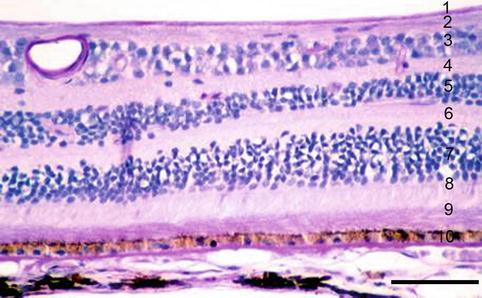
Fig. 1.18
The retinal layers. 1 internal limiting membrane, 2 nerve fibre layer, 3 ganglion cell layer, 4 inner plexiform layer, 5 inner nuclear layer, 6 outer plexiform layer. 7 outer nuclear layer, 8 outer limiting membrane, 9 photoreceptor outer segments. 10 retinal pigment epithelium. H&E, bar = 100 μm
RPE is a regular monolayer of highly pigmented cells continuous with the pigmented epithelium of the ciliary body. Its basal cytoplasmic membranes and its basement membrane form the inner layer of Bruch’s membrane. The apical surface of the RPE cells has processes that surround the photoreceptor outer segments. The main functions include the outer blood-retina barrier (active transport of nutrients to the outer retinal layers), vitamin A metabolism, absorption of stray light and maintenance of the outer segments of the photoreceptors.
The neurosensory retina consists of neuronal and glial cells. The photoreceptors are highly specialised cells – rods and cones. Their outer segments are in contact with the apical processes of the RPE surrounded by a mucopolysaccharide matrix. The inner segment contains the synaptic body to establish the connection with dendrites from bipolar cells and horizontal cells. Axons from bipolar cells synapse with ganglion and amacrine cells. The axons from ganglion cells become parallel to the inner surface of the retina to form the nerve fibre layer and to merge to constitute the optic nerve. Temporal fibres follow a curved path around the macula, and fibres from the fovea travel straight to the optic nerve. Glial cells are mainly Müller cells that extend from the external to the internal limiting membrane and together with astrocytes and microglia provide support and nutrition to the retina.
The central retina – the posterior pole or the macula – lies between the temporal vascular arcades; outside this area is the peripheral retina. In the centre of the macula is an area 1.5 mm in diameter – the fovea – responsible for higher visual acuity and colour vision. At the centre of the fovea is a central depression, the foveola, approximately 0.35 mm in diameter. In this area, there is only the photoreceptor layer composed exclusively of cones and responsible for the highest visual acuity. In the macular area the retina is thicker; the RPE cells are taller and contain more and larger melanosomes. In the neuroretina of this region, the cones are still the dominant photoreceptor type, and the ganglion cell layer has several rows of cells (Fig. 1.19). In the periphery the retina is still thinner and dominated by rods (Fig. 1.20).
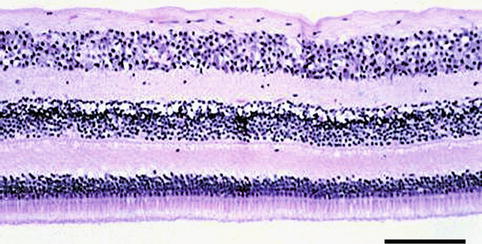
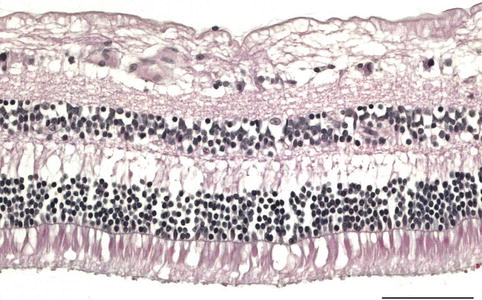
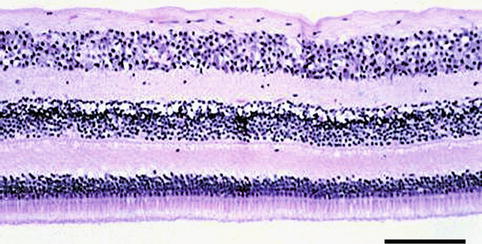
Fig. 1.19
Retina at the macular area. Note the thick ganglion cell layer. H&E, bar = 100 μm
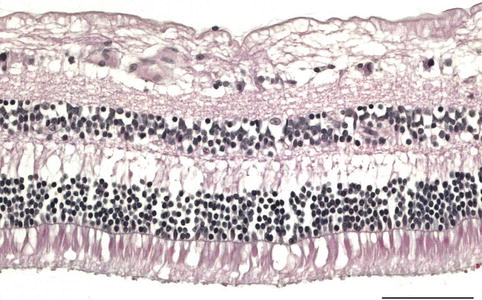
Fig. 1.20
Retina at the equator has less ganglion cells than at the macula. H&E, bar = 100 μm
1.2.6 Optic Nerve
The ganglion cell axons meet at the back of the eyeball, preserving the retinotopic organisation in the optic nerve head formed by four layers.
The superficial nerve fibre layer, composed of the non-myelinated ganglion cell axons with spots of myelinated fibres resulting from migration of oligodendrocytes through the lamina cribrosa.
The pre-laminar region in which the ganglion cell axons are organised into bundles supported by astrocytic glial cells that pass through the lamina cribrosa.
The laminar portion or lamina cribrosa consists of sheets of connective tissue with type I and III collagen, elastin, laminin and fibronectin, which comprise about ten fenestrated connective plates integrated with the sclera, and with pores traversed by the axon bundles (Fig. 1.21).
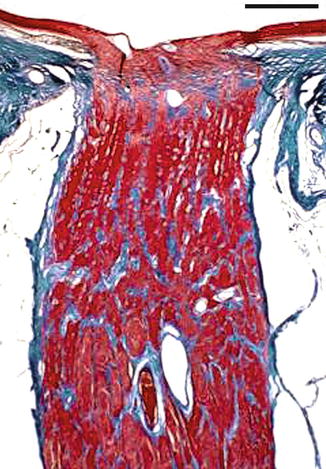
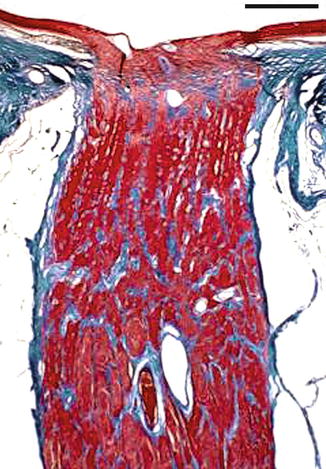
Fig. 1.21
Longitudinal section of the optic nerve. Masson trichrome stain, bar = 100 μm
The retro-laminar portion, where nerve fibres become myelinated, is surrounded by meningeal sheaths constituting the optic nerve. This portion continues to the apex of the orbit forming the orbital part of the optic nerve (Fig. 1.21).
1.2.7 Lens
The lens is a biconvex structure located behind the posterior chamber and the pupil. Its main function is refractive. Reduced tension of the zonules, controlled by the ciliary muscle, changes the radius of curvature of the lens, creating the accommodation required for near vision. The lens lacks innervation, is avascular and depends on the aqueous and vitreous humour for nutrition. To understand the structure of the lens, it is necessary to remember its embryologic origin from an invagination of surface ectoderm. The lens capsule is the basement membrane of the lens epithelium. It is thicker in the front (as it is produced throughout life by the anterior epithelium) than in the back (only produced during the embryonic period where the posterior epithelium still exists as an epithelium). The anterior epithelium is a low prismatic monolayer located under the anterior capsule. It grows radially, and at the lens equator, the cells divide and migrate into the lens vesicle. The cells elongate, sending processes anteriorly and posteriorly, and mature to be lens fibres, forming the adult lens cortex. Mitotic activity is highest in the pre- and equatorial lens epithelium known as the germinative zone. The lens sutures are formed by the interdigitations of the anterior and posterior tips of the fibres. When new lens fibres are formed, the older are pushed deeper into the cortex. The old lens fibres do not contain nuclei. In the final transformation, the fibres fuse forming the adult lens nucleus.
1.2.8 Vitreous Body
The vitreous cavity occupies four fifth of the posterior eyeball having a volume close to 4 ml. The cavity contains the vitreous body, which has a gel-like structure. It contains 99 % water together with collagen fibres in which hyaluronic acid molecules are bound. Although the vitreous body is mainly acellular, a few cells – hyalocytes – appear mainly in the cortex. The main function of the vitreous body is the metabolic maintenance of intraocular structures like lens, ciliary body and retina. The vitreous body consists of the cortical and the central vitreous. The cortical vitreous contains more densely packed collagen fibrils that at the peripheral border form the hyaloid membrane. The hyaloid membrane is attached to the retina and pars plana by condensation of collagen fibrils. The binding is tight at the peripheral retina, along the margins of the optic disc and around the fovea and retinal vessels. The central vitreous is traversed by a central canal, the hyaloid or Cloquet’s canal, representing the remnants of the hyaloid artery in the foetal eye.
1.3 Development
1.3.1 The Eyeball
The globe as such evolves throughout life, and the physiological changes can be observed as growth and ageing. The anterior-posterior diameter of the eye is approximately 16 mm at birth, reaching around 23 mm by 3 years of age and adult size before puberty. Details of the ocular development are presented in resent publications [8, 11].
In the cornea the Descemet’s membrane increases its thickness through life. In elderly people, peripheral excrescences known as Hassall-Henle warts develop at the posterior aspect of the Descemet’s membrane. Central excrescences (cornea guttata) may also appear with increasing age (Fig. 1.22). The number of endothelial cells decreases with age. There are approximately 4,000 cells/mm2 at birth, 2,500 in middle age and 2,000 in old age. Consequently, with age, the hexagonal arrangement typical of young corneas is replaced by a fewer cells with heterogeneous size and shape. These cells have a low regenerative capacity and decrease in number with age, and lost cells are replaced by spreading of the adjacent cells.
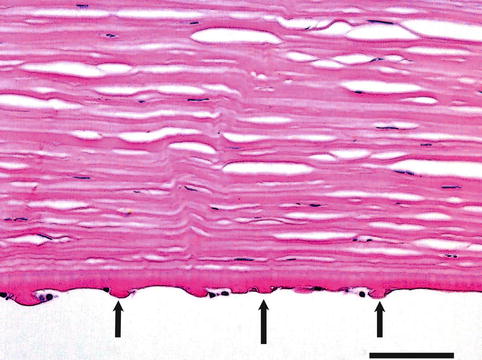
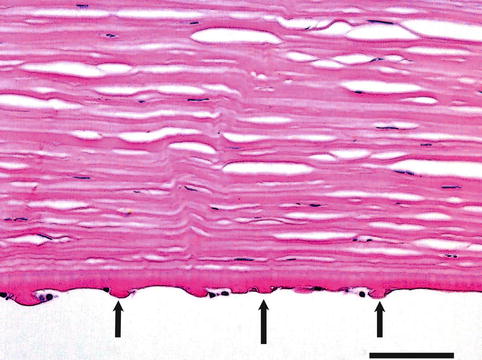
Fig. 1.22
Inner aspect of the cornea in a person with Fuch’s endothelial dystrophy. Arrows at guttate excrescences on Descemet’s membrane. H&E, bar = 75 μm
The population of endothelial cells of the trabecular meshwork also decreases over time, and together with the deposition of extracellular materials in the trabeculum, the resistance to aqueous humour outflow increases and the intraocular pressure increases.
In the lens, the continuous process of proliferation and differentiation of epithelial cells from the equator and in ward continues throughout life. The lens nucleus increases the size and transparency gradually decreases. The anterior epithelium may disappear focally or may undergo fibrous metaplasia (anterior subcapsular opacities) or may migrate to the posterior subcapsular area.
Microcysts may appear in the peripheral retina immediately behind the ora serrata due to degenerative occlusive disease in the peripheral arterioles producing ischaemia: peripheral micro-cystoid degeneration. Cyst may join to form a retinoschisis in the outer plexiform layer or a large cyst. RPE display pleomorphism and a decrease in number. With time, the material from the degradation of outer segment discs gradually accumulates within the RPE as lipofuscin. A further, aberrant excretion of the material takes place in the form of basal laminar deposits and other substances at the retinal side of the Bruch’s membrane forming drusen (Fig. 1.23).
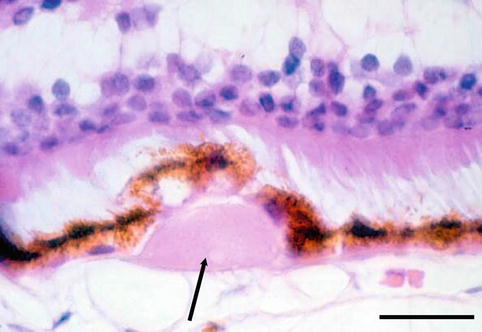
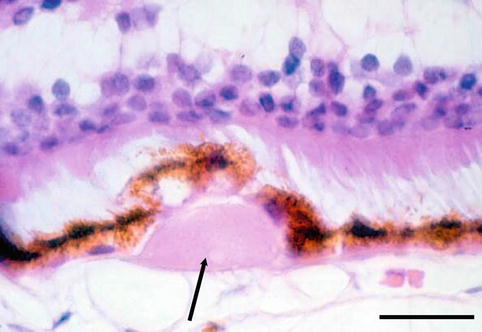
Fig. 1.23
Drusen (arrow) between the retinal pigment epithelium and Bruch’s membrane. H&E, bar = 50 μm
1.4 Congenital Abnormalities
1.4.1 Anophthalmia
Definition
Anophthalmia means, in ophthalmic pathology, a complete lack of any ocular structures in the orbit.
Synonyms
Anophthalmos, anopia
Epidemiology
Extremely rare. In our files at ØPI-DK, there are none.
Aetiology
Complete lack of development of the ophthalmic vesicle in the fourth embryonic week results in a complete lack of an eyeball or any bulbar structures in a small orbit that contains orbital fat, vessels, peripheral nerves, rectus muscles (from the mesoderm not induced by the ophthalmic vesicle) and tear gland.
Clinical Features
The patient presents with rather normal eyelids and conjunctiva in a small orbit. No ocular structures can be detected by palpation and imaging including ultrasound, and no movements of an eye can be discerned. Mostly, when an eye cannot be detected clinically, anophthalmia is diagnosed. However, the histology of orbital tissue from such cases most often demonstrates rudimentary eye structures, i.e. a case of microphthalmia.
Genetics
A number of genetic disturbances are known to be involved in anophthalmia; however, these are also bound to the presence of microphthalmia, and since most of these studies are not based on a very careful analysis of the content of the orbit harbouring the anophthalmic eye, the known gene defects are termed to cause anophthalmia/microphthalmia. A recent review presents an up-to-date survey [12]. Some defects are also described under microphthalmia below.
1.4.2 Congenital Cystic Eye
Definition
Cystic structure lined by connective tissue, pigmented cells and glial strands
Epidemiology
Very rare
Aetiology
At the 7.5 mm stage of the embryo, the optic vesicle invaginates to form the optic cup. If this process is disturbed at this stage, the result is a disorganised cystic structure. The content of the cyst is glial structures, and the wall contains scleral lamellas, muscle and fibrovascular tissue.
1.4.3 Microphthalmia
Definition
The presence of a rudimentary eye in the orbit
Epidemiology
Aetiology
Clinical Features
Histopathology
Histologically, the findings are very varied from small strands of neuroepithelium and lens anlage to small eyes with anterior segment dysgenesis, iris coloboma, cataract retinal dysplasia and optic disc hypoplasia (Fig. 1.24) [18].
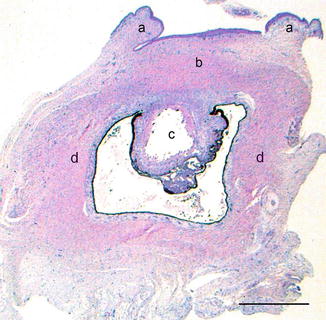
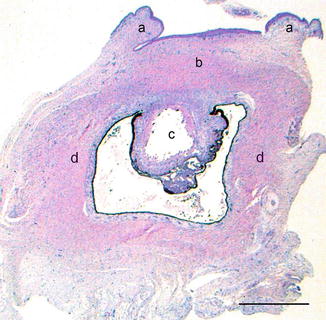
Fig. 1.24
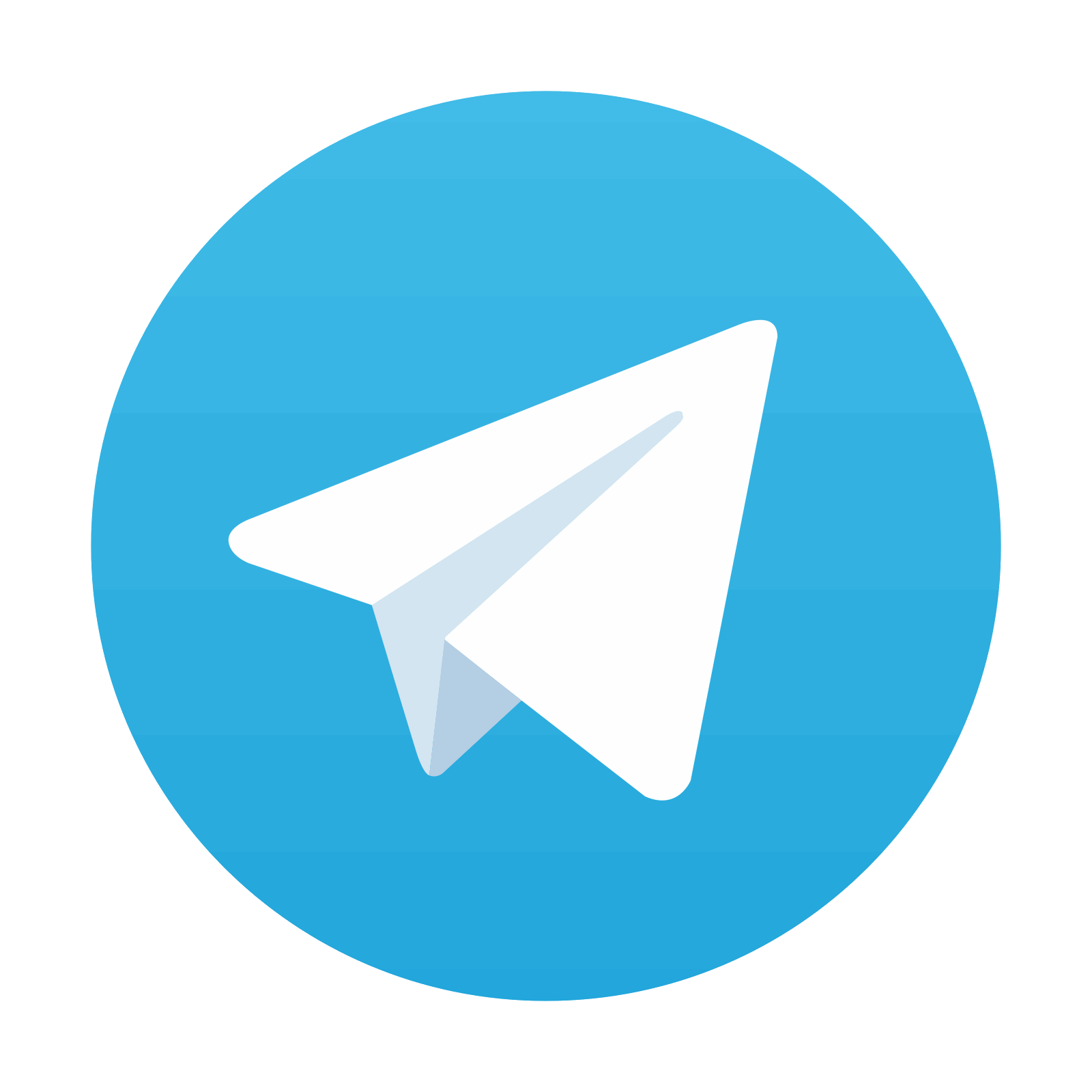
Foetal microphthalmic eye. Eyelids are present (a). The anterior chamber is not formed and the cornea (b) rests against the cataractous lens (c). The sclera is formed (d). H&E, bar = 400 μm
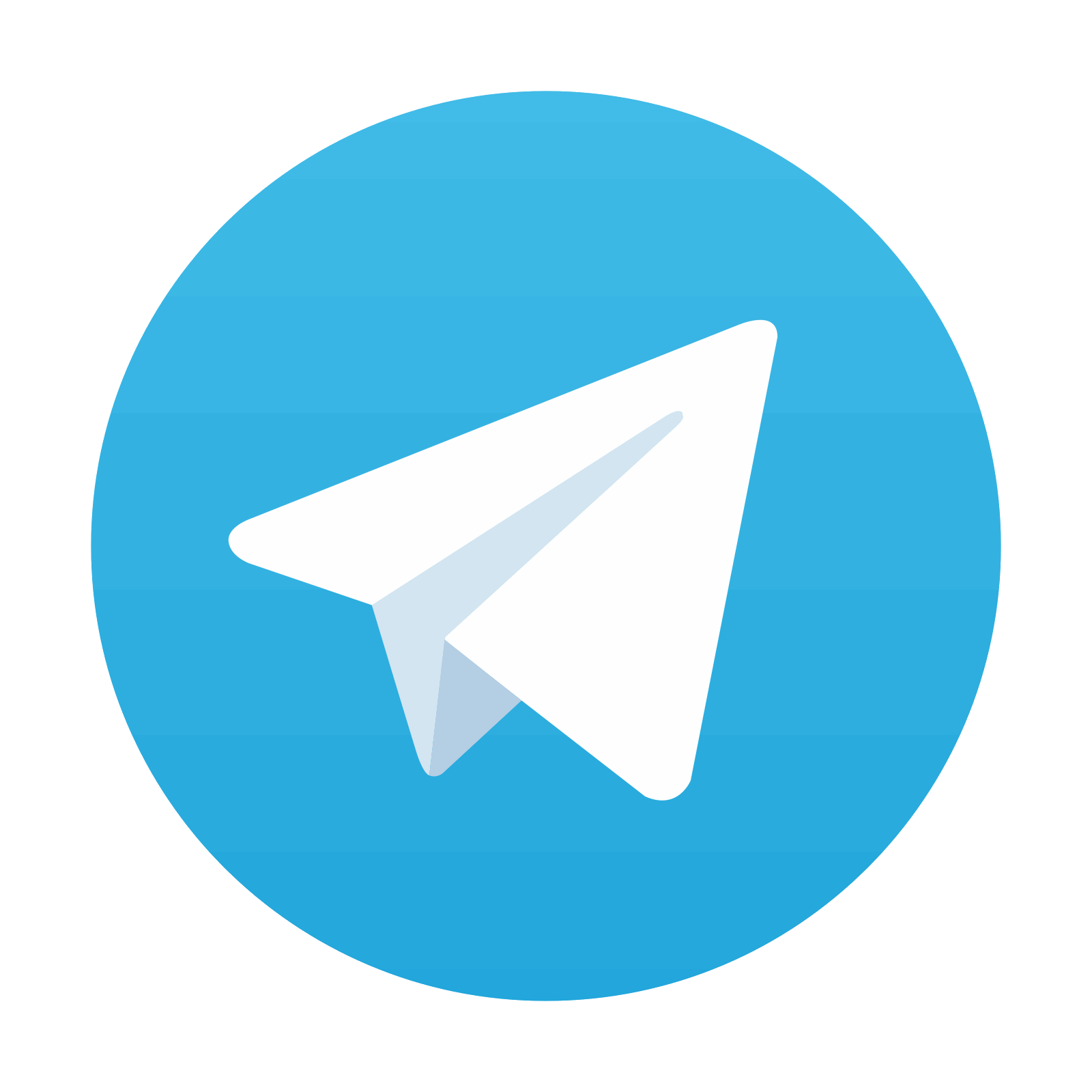
Stay updated, free articles. Join our Telegram channel

Full access? Get Clinical Tree
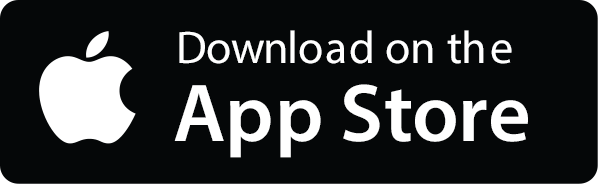
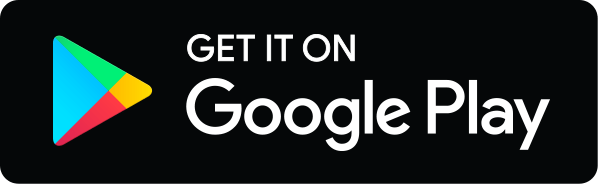
