Fig. 6.1
(a) Intense glaucomatous excavation of the optic nerve head, in comparison to small physiological central excavation (b) (Courtesy of Christian Richter, University Eye Hospital Freiburg)
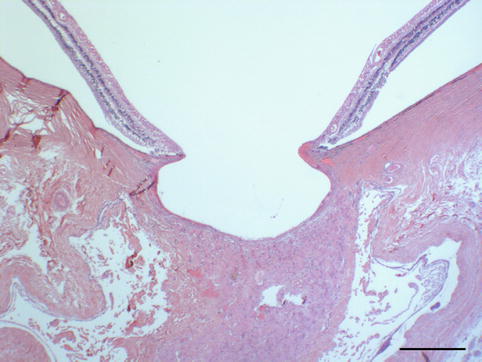
Fig. 6.2
Optic nerve head excavation in progressive pseudoexfoliation glaucoma (same eye as Fig. 6.10). Bar = 0.3 mm
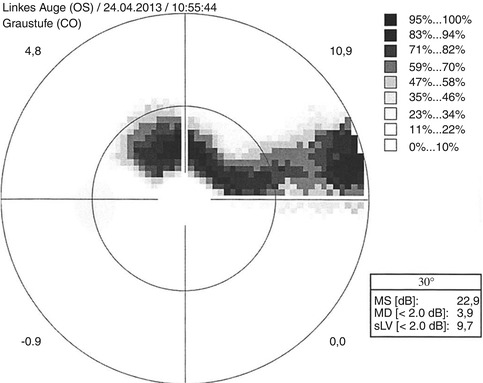
Fig. 6.3
Bjerrum scotoma, a sickle-shaped scotoma with extension to the blind spot which can be located superiorly, here in automated 30° perimetry of a left eye (Courtesy of Milena Stech, University Eye Hospital Freiburg)
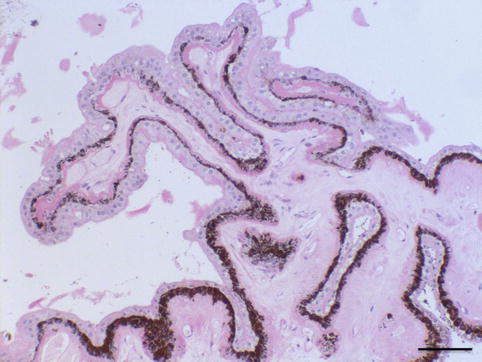
Fig. 6.4
Ciliary body with slight fibrosis and normal pigmented and unpigmented epithelium (PAS, bar = 50 μm)
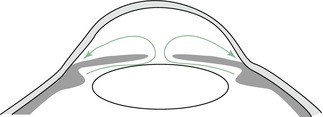
Fig. 6.5
Scheme of the aqueous humour flow from the ciliary body towards the trabecular meshwork passing the slit between the lens and pupil (Courtesy of Dr. Günther Schlunck, University Eye Hospital Freiburg)
The other forms of glaucoma show impaired outflow of the aqueous humour due to angle-closure, pretrabecular or posttrabecular problems. Angle closure tends to occur in short eyes (under 21 mm axial length), especially in mydriasis with pupil dilation followed by a dramatic decrease of the aqueous humour outflow with acute increase of intraocular pressure reaching 70 mmHg or more (Fig. 6.6, “primary angle-closure glaucoma”).
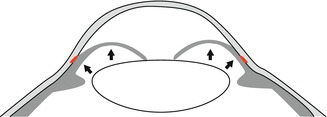
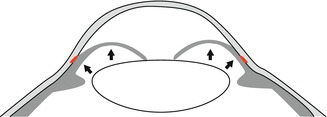
Fig. 6.6
Scheme of a pupillary block with blockage of the aqueous humour flow between the lens and iris and forward bulging of the iris obstructing the anterior chamber angle (red)
This situation can be imitated in normal-sized eyes by thickening of the lens or a process of the ciliary body pressing upon the anterior chamber angle from behind, like a ciliary neoplasm, e.g. malignant melanoma. The treatment of the acute angle-closure glaucoma consists in systemic carboanhydrase inhibition leading to immediate reduction of the aqueous humour production and for the long term a surgical iridectomy in primary closed-angle glaucoma where the pupillary block is bypassed and the aqueous humour directly flows from the posterior into the anterior chamber through the artificial opening in the iris basis (Fig. 6.7).
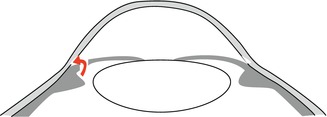
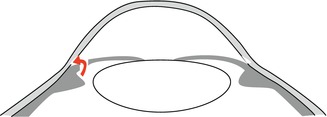
Fig. 6.7
Flow of the aqueous humour in pupillary block after performed iridectomy (Courtesy of Dr. Günther Schlunck, University Eye Hospital Freiburg)
Pretrabecular blockage of the aqueous flow occurs in developmental glaucomas and in eyes with ingrowth of various tissue types as fibro (vascular) tissues or epithelial cells. Posttrabecular blockage of the aqueous flow occurs in processes, which impair the flow of the episcleral veins as it happens in patients with nevus flammeus of the face or in sinus cavernosus thrombosis. This chapter contains only processes confined to the eye. While the different morphological changes of the anterior segment leading to increased intraocular pressure are described in the following sections of the chapter according to their specific underlying pathogenesis, the morphological changes of the posterior segment are quite uniform in the different glaucoma types and will be addressed here.
Progression of glaucoma-related optic nerve damage is judged by the morphological changes of the optic nerve head (ONH). The ONH shows a pathological excavation (Figs. 6.1 and 6.2), which enlarges in the case of progression. Histologically, the morphological changes of the glaucomatous excavated ONH consist of nerve fibre atrophy, alterations of the lamina cribrosa and glial cell activation. The lamina cribrosa is typically bowed posteriorly with loss of nerve fibres, neural connective tissue and vessels. Within years, the lamina cribrosa becomes attenuated and the edge below the scleral ring bulged due to a remodelling process: increased intraocular pressure and ischemia activate glial cells, i.e. astrocytes and Müller cells, which induce degenerative changes in the extracellular matrix, upregulation of matrix metalloproteinases, proteolytic enzymes, cytokines and tenascin [3, 4]. The progression of glaucomatous excavation is thus considered not only as the result of mechanical impact of elevated intraocular pressure but a complex bioactive process. As a secondary change, atrophy of the retinal ganglion cell layer occurs (Fig. 6.8).
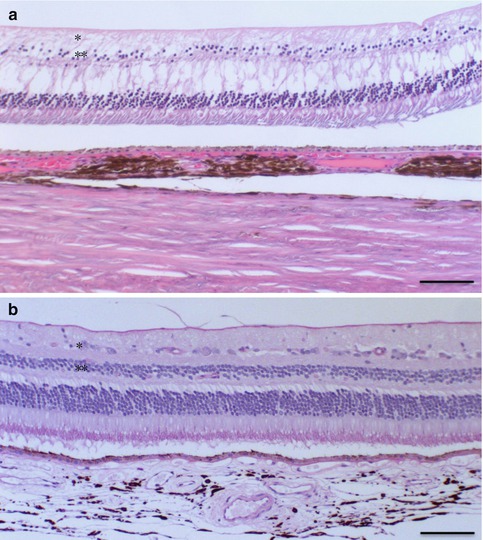
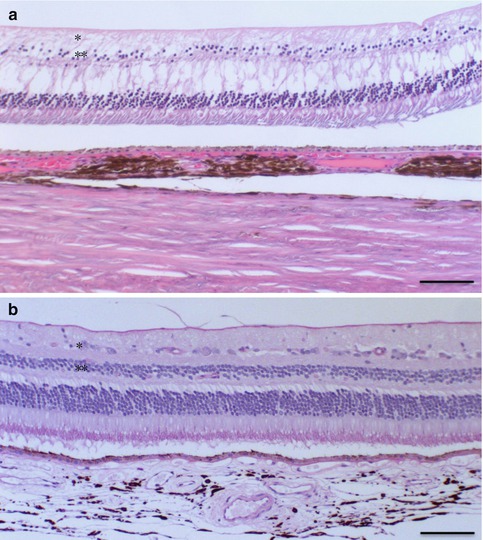
Fig. 6.8
Atrophy of the retinal ganglion cell layer and the outer nuclear layer (**) in secondary glaucoma following contusion and panretinal laser treatment with clumping of choroidal melanocytes (a, above), in comparison to the normal eye (b, below), HE, bar = 100 μm
6.2 Anatomy and Embryology
6.2.1 Anatomy
Most important anatomical landmarks involved in glaucoma are the ciliary body as producer of the aqueous humour, the anterior chamber angle (posterior cornea, trabecular meshwork and iris root) as drainage place and the optic nerve with the ganglion cell layer in the retina as structural endpoint of glaucoma damage. Episcleral veins drain the humour of Schlemm’s canal via intrascleral channels out of the eye into the conjunctival veins.
The trabecular meshwork is a three-dimensional sieve-like structure in a triangle in the angle between the iris root and the posterior side of the cornea (Fig. 6.9a–c).
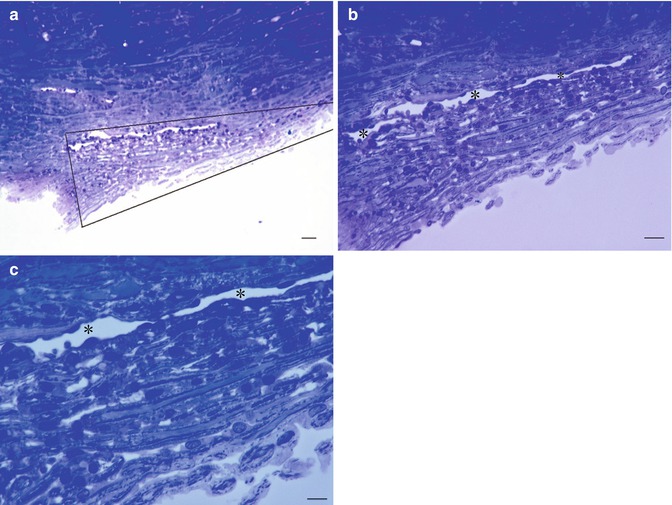
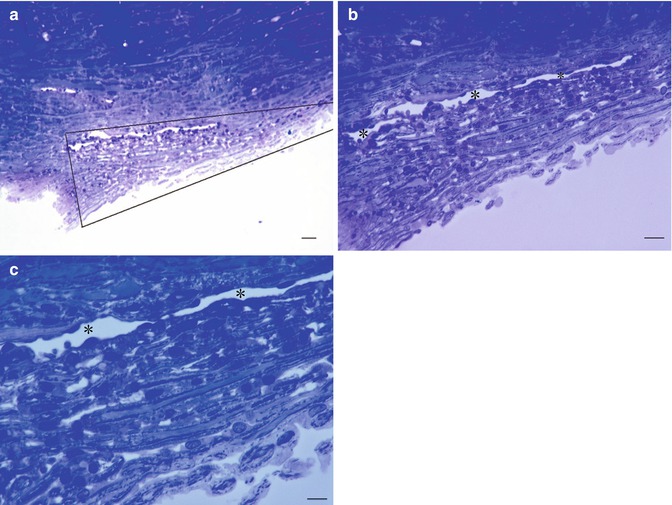
Fig. 6.9
Normal trabecular meshwork and Schlemm’s canal from donor eye of a 52-year-old patient, toluidine blue staining. (a) Overview of the sieve-like, triangular structure. Trabecular meshwork is between the lines of the triangle. (b, c) Further magnification of (a). Bars = 100 μm, 50 μm and 25 μm, resp
It is built of a core of collagen fibres with elastin and covered by a thin layer of endothelium cells. The mechanism of filtration of fluid must be kept in balance to keep the pressure in the eye at very constant levels; therefore, there is no direct communication: the fluid leaves the anterior chamber through the trabecular meshwork by giant vacuoles (Fig. 6.10).
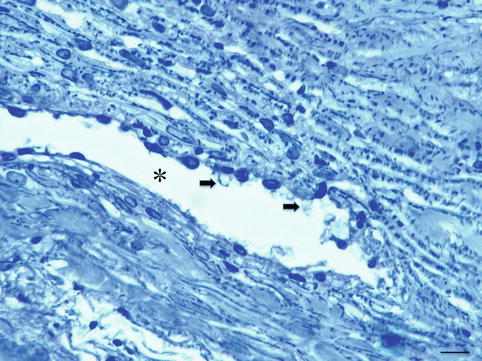
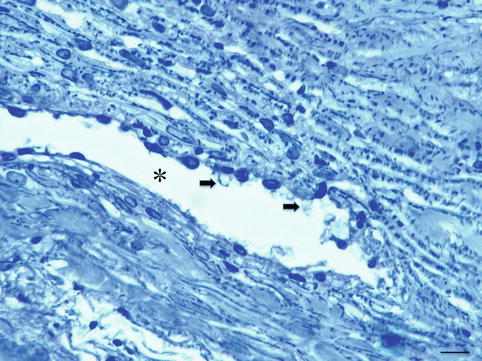
Fig. 6.10
Trabeculectomy specimen of a 79-year-old glaucoma patient. Trabecular meshwork and Schlemm’s canal (*) with giant vacuoles (arrows), toluidine blue staining, bar = 25 μm
The meshwork may be divided into three anatomical portions: (a) uveal meshwork, (b) corneoscleral meshwork and (c) juxtacanalicular tissue which is also referred to as the cribriform layer.
The uveal meshwork: this inner most portion is adjacent to the aqueous humour in the anterior chamber and is arranged in bands or ropelike trabeculae that extend from the iris root and ciliary body to the peripheral cornea. The arrangement of the trabecular bands creates irregular openings that vary in size from 25 to 75 μ [5]. The corneoscleral meshwork: this portion extends from the scleral spur to the anterior wall of the scleral sulcus and consists of sheets of trabeculae that are perforated by elliptical openings. These holes become progressively smaller as the trabecular sheets approach Schlemm’s canal with a diameter range of 5–50 μm [5]. The trabecular bands or sheets are composed of four concentric layers. First, an inner connective tissue core of typical collagen fibres types I and II and elastin [6, 7], basement membrane and continuous covering endothelium forms. The outermost portion of the meshwork adjacent to Schlemm’s canal consists of a layer of connective tissue lined on either side by endothelium; the outer endothelial layer comprises the inner wall of Schlemm’s canal, in between is a central connective tissue layer with variable thickness and not fenestrated with several layers of parallel spindle-shaped cells loosely arranged in a connective tissue ground substance [8].
Schlemm’s canal is a circular channel in the sclera and lined by a single endothelial layer. The anterior-posterior length is between 250 and 300 μ; the final flow of aqueous to this canal is by transition through giant vacuoles in the lining endothelium. Many of the cells of the inner wall contain giant vacuoles [9, 10]. These vacuoles seem to be transcellular micro channels by which aqueous humour can pass into Schlemm’s canal. From the outer wall of Schlemm’s canal, 25–35 channels emerge, which are connected to the vascular system of the limbal region.
The optic nerve head (or optic disc, or papilla) is formed by 1.1–1.2 million axons of retinal ganglion cells and glial cells, which behave as astrocytes or supporting cells. In the optic nerve head, these axons are bundled as regularly aligned and spaced columns [11]. Only outside the eye the glial cells form myelin and behave as oligodendrocytes. Between these fibres are the connective tissue components with vascular supply. At the optic nerve head, all the axons of the retinal nerve fibre layer come together and become so raised towards the vitreal surface that they create the nipple-lie elevation that gave rise to the designation of “papilla”. The optic nerve head is about 1 mm in length and is often described in three parts [11]: the lamina retinalis, the lamina choroidalis and the lamina scleralis; other terms used to describe the regional histology of the optic disc are based on the position of the lamina cribrosa of the sclera; these terms are prelaminar, laminar and retrolaminar. The lamina cribrosa is the vascular connective tissue that penetrates the lamina scleralis of the optic nerve head. The lamina scleralis or the posterior scleral foramen is a truncated cone having an anterior diameter of 2.0 mm or less and a posterior diameter of 3.5 mm. The sclera is perforated by approximately 300 canals that are extensions of the connective tissue of the inner one-third of the sclera, the lamina cribrosa. The other two-thirds of the sclera do not contribute to the lamina cribrosa but blend into the dural attachments of the optic nerve.
The increase of the physiological cup of the optic nerve head is the most accessible sign of POAG damage and is the result of neuronal atrophy and connective tissue changes with concave bending of the lamina cribrosa. Clinically, optic nerve damage is recognised by enlarging and deepening of the optic cup accompanied by thinning of the neural rim.
Cupping of the optic disc may occur as a part of normal embryologic development or as a part of a pathological process. The size of the optic cup is highly variable and depends on the size of the scleral canal. Cupping results from a mismatch of the size of the scleral canal with the number of axons passing through the optic nerve head. In glaucoma, cupping occurs when there is a decreased amount of neural tissue entering the scleral canal. Studies of the lamina cribrosa in glaucoma patients have shown collapse and rotation of the plates of the lamina cribrosa which would distort axon paths, interrupt axonal transport and predispose to cell death probably by apoptosis [5, 12].
6.2.2 Embryology
The eye is formed in the very early stages of development within the third and fourth week of foetal life, and if the formation of the anterior chamber is not perfect, there are repercussions on the cornea, lens, iris, trabecular meshwork and outflow [13, 14]. This means that in most developmental problems, all these structures can be more or less involved. The known genes in these diseases specify the problem, but the individual expression of the syndrome can even in dominant cases be very different in members of the same family.
In the development of the eye, mostly cells derived from the neuroectoderm play a role [15]. In the third gestational week, small bulbs sprout on both sides out of the wall of the diencephalon in what later becomes the forebrain region. With increasing growth, the bulb-like optic vesicles invaginate to form the two optic cups. The interior layer of the optic cup will become the retina and the exterior layer will develop into the pigment epithelium. The proximal region of the optic cup will form the optic stalk and develop later in the optic nerve.
In response to inductive signals, the overlying surface ectoderm which covers the region of the optic cup forms a vesicle as well just opposite of the optic cup and separates later from the superficial ectoderm. This then provides the basis for future lens development. In this stage, the Pax6 gene is mostly involved [16]. From the same ectoderm will form the corneal and conjunctival epithelium and at a later embryonic stage, the eyelids. All the other ocular structures are developed from cranial neural crest cells and cranial paraxial mesoderm-derived cells.
During the third gestational month, the lens is situated directly behind the cornea, the iris has only partially developed and the anterior chamber will gradually deepen during the weeks and months that follow. This leads to an increased distance between the lens and cornea. The ciliary body begins to form; the next step is the growth and maturation of the iris and the anterior chamber. The insertion of the iris and ciliary body migrates posteriorly, which is a necessary step for TM development. At 7 months gestation, the TM is separated from the anterior chamber by the layer of neural crest cells and little aqueous can drain. Retraction of these cells allows exposure of the TM and therefore drainage of aqueous (Fig. 6.11a–d) [17].
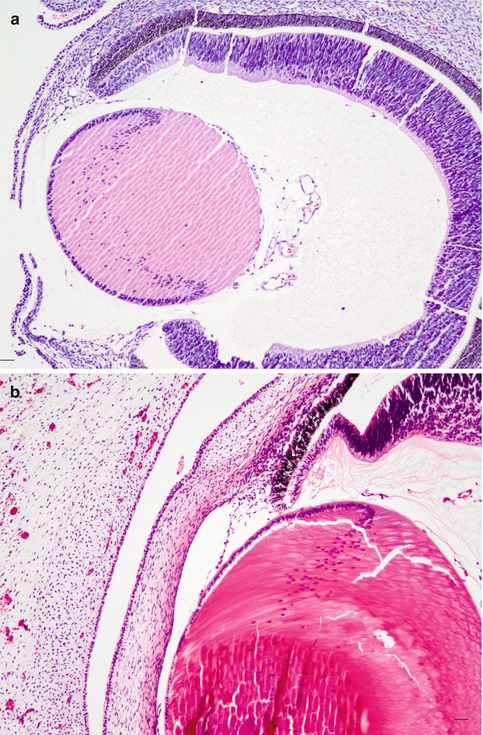
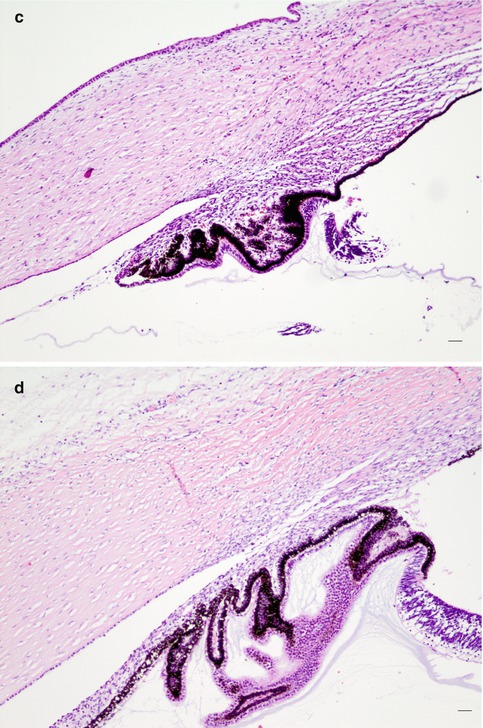
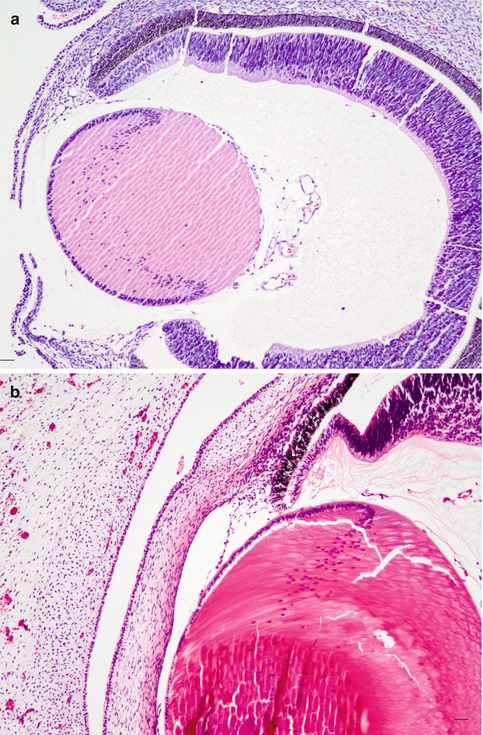
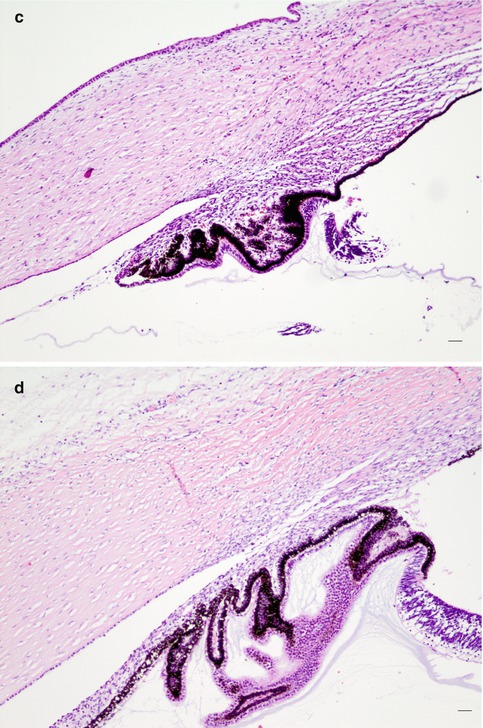
Fig. 6.11
(a) A 7-week-old foetus (HE, bar = 50 μm); (b) 12-week-old; (c) 18-week-old; (d) 23-week-old (HE, bars = 20 μm). Major steps in the development of the eye, HE staining. At 7 and 12 weeks’ gestational age, the iris is not yet separated from the pigmented layer. At 18 weeks, a rudimentary iris starts to sprout out. At 23 weeks, the anterior chamber angle is open with an immature iris and trabecular meshwork (Courtesy of Dr Rob Verdyck)
If this process of differentiation is defective or incomplete, the newborn’s trabecular meshwork cannot properly drain the aqueous humour, thus resulting in an increase of intraocular pressure (IOP). In all cases of aberrant lens development, the normal formation of the anterior chamber is also disturbed and developmental glaucoma is often found in neonatal cataract syndromes [18].
The development of the optic nerve starts with the out sprouting growth of the axons of the ganglion cells of the retina; these nerve fibres find their way through the optic disc and form the optic nerve and the optic chiasm and end in the brain in the lateral geniculate bodies. Much more nerve fibres are formed during this process, but only a small part find his connection to the brain; the other part disappears in a self-controlling way. Only later they are populated by septa of vascular connective tissue to form the blood supply and the structural support to pass through the scleral openings.
6.3 Developmental Glaucoma
The most common reason for an IOP increase is reduced outflow capacity usually located at the anterior chamber angle and the trabecular meshwork. Although the chance of developing an elevated IOP significantly rises as one ages, an increase in the IOP can occur at any age. Congenital glaucoma affects babies who are born with high IOP or develop an increase shortly after birth. An elevated IOP during childhood is defined as infantile glaucoma.
The term ocular anterior segment dysgeneses (anterior segment dysgeneses-neural crest, anterior segment cleavage syndrome) is a newer name for a genetically heterogeneous group of developmental disorders with malformations of the structures of the anterior segment that share some common features and a high prevalence of associated glaucoma [16, 19, 20]. Glaucoma can also be part of a syndrome with other ocular and non-ocular malformations, called developmental glaucoma with associated anomalies. In all these cases, there is a malformation of parts of the anterior chamber structures with malfunction of the trabecular meshwork. The most common genetic causes of anterior segment dysgeneses have been identified as mutations affecting the function of the PITX2 and FOXC1 transcription factors [21].
The most prevalent conditions associated with glaucoma, although all very rare, are primary congenital glaucoma, Axenfeld and Rieger anomaly, Peters anomaly and aniridia. Classification was formerly based on clinical grounds, but since more genes are detected, other categories will be formed [21].
Although medical management of developmental glaucoma is often a temporising measure, early surgical intervention is the definitive treatment. The choices of techniques are directed by the stepladder classification: in simple cases of congenital glaucoma, first, a goniotomy is performed then a trabeculotomy; if this treatment is not sufficiently lowering the pressure, a trabeculectomy is executed, followed by eventually a second trabeculectomy. It this is not successful, a glaucoma drainage device is implanted. If all these techniques are failing, then as last hope, cyclodestructive procedures are necessary [22].
6.3.1 Primary Congenital Glaucoma
Congenital glaucoma is a rare form of glaucoma. 1/10,000 newborns are affected. Both eyes are usually involved but to varying degrees; boys are affected slightly more frequently than girls. A hereditary factor is occasionally present and is mostly autosomal recessive; it is rare except in consanguineous marriages. The most prevalent gene involved is CYP1B1 [23]. The IOP elevation is caused by the failure of the anterior chamber angle and the trabecular meshwork to develop appropriately during intrauterine development. The trabecular meshwork has not yet fully developed and is still partially covered by a non-perforated immature structure of collagen fibres [24]. As consequence, the eyeball enlarges due to distensible sclera of a baby and especially the cornea expands (megalocornea). The Descemet membrane of the cornea is less elastic, and stretching may result in small tears that cause a central opacification (Fig. 6.12) (see also page 21). These opacifications form lines, called Haab’s stria, and are visible for the rest of life. Histologically, they are visible as longitudinal scrolls of the PAS-positive Descemet membrane at the posterior side of the cornea (Fig. 6.12c, d), with an increased diameter of the cornea. There is an anterior displacement of the iris insertion and ciliary body [25, 26].
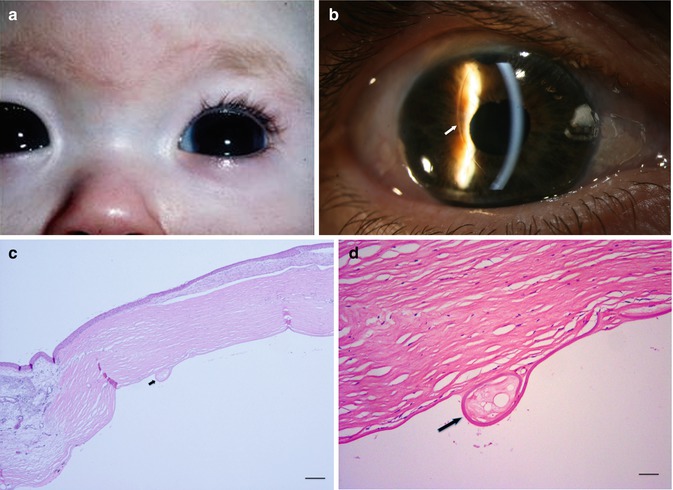
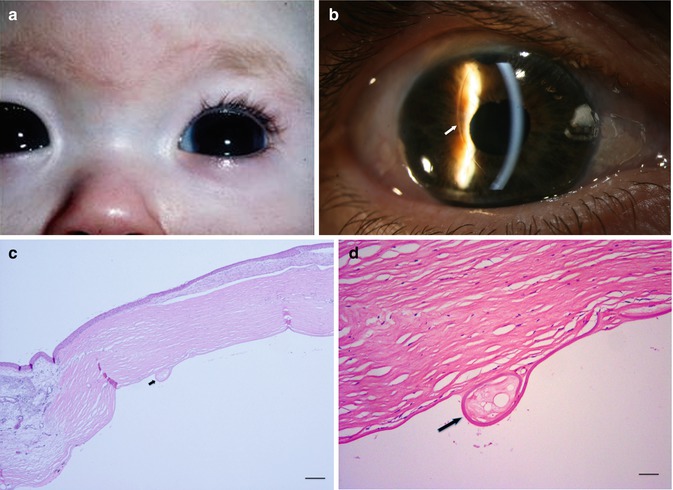
Fig. 6.12
(a) Congenital glaucoma baby with bilateral enlarged cornea (Courtesy of Ingele Casteels, Department of Ophthalmology, Leuven). (b) Clinical picture of Haab’s striae in a 63-year-old adult (arrow), bilateral Haab’s striae, diagnosed as baby with congenital glaucoma. Vertical bended line at the posterior side of the cornea, visible in retrograde illumination; signs of anterior synechiae between the cornea and iris at 5 o’ clock. (c, d) Cornea with image of Haab’s striae as Descemet scroll, after evisceration of blind eye with congenital glaucoma (arrow), HE staining. Bars = 100 and 50 μm, resp
6.3.2 Axenfeld and Rieger Anomaly (AXRA)
In AXRA, tissue strands of variable thickness are bridging the anterior chamber from the peripheral iris to a prominent anteriorly displaced Schwalbe’s line (white line on the posterior aspect of the cornea); there is thinning of the iris with eventual hole formation in the iris [19]. The disorder in which this Schwalbe’s line is markedly anteriorly displaced and thickened is called “posterior embryotoxon” [27, 28]. It may be an isolated finding without glaucoma or seen in the presence of other ocular anomalies, in particular AXRA syndrome [29].
6.3.3 Peters Anomaly
This anomaly is caused by focal absence of corneal endothelium, Descemet membrane and posterior corneal stroma. Usually, this occurs in the central cornea, leading to a central corneal opacity. Iris synechiae extend from the iris collarette to this defect. There are adhesions between the lens and the cornea with corneal opacity. Glaucoma occurs in about 50 % of cases [31]. In more severe cases, the lens, iris and cornea are not separated from each other without an open anterior chamber and forward bulging of the cornea can occur (also known as anterior staphyloma).
This causes glaucoma in 90 % of cases, which is difficult to manage and often refractory to medications. Treatment must be performed in very young age because of the risk of glaucoma damage and amblyopia. The structural defects have their origin in a genetic defect, different genes are responsible; recessive as well as dominant familial cases and sporadic patients are known. The most prevalent gene defects are located in the Pax6, C-Maf and Fox genes [19–21]. In sporadic cases, some environmental influences are present as in foetal alcoholic syndrome. The formation of the anterior segment occurs in the first trimester of pregnancy and babies are born with this congenital defect. There is a broad spectrum of clinical findings, also in the same family with the same gene defect and also a large variation between the right and left eye. Peters anomaly may also be associated with other ocular or systemic abnormalities (trisomy 13–15, Norrie disease). Most cases are bilateral and asymmetric (Fig. 6.13).
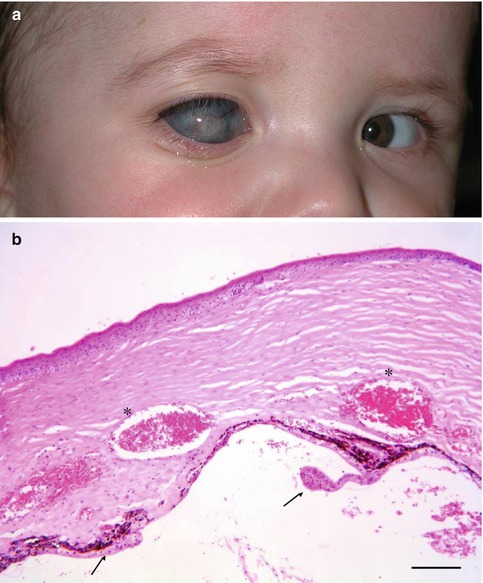
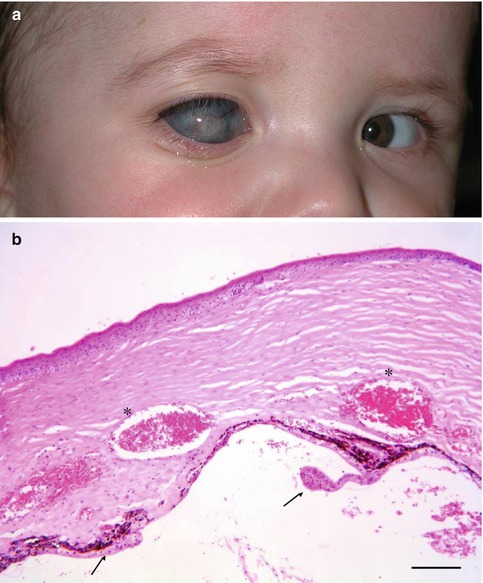
Fig. 6.13
(a) Child with severe form of unilateral Peters anomaly with enlarged and hazy vascularised cornea. (b) Histopathology of cornea after evisceration of eye with Peters anomaly. Irregular corneal stroma with abnormal vascularisation (asterisks); iris and ciliary body remnants on the posterior side of the cornea (arrows). H&E staining. Bar = 100 μm
6.3.4 Aniridia
In aniridia, the iris is only rudimentary developed [32]. The spectrum of this disease varies from a nearly normal thin iris to very small iris tufts at the normal place of the iris root. The gene Pax6 had a wide expression in the developing eye and Pax6 mutations account for most cases of aniridia; the majority of Pax6 mutations are nonsense, splicing, insertions and deletions [16, 18]. The TM changes are variable, which also accounts for a variable glaucoma disease. During embryogenesis, there is a disruption of the trabecular meshwork development and adhesion between the iris periphery and cornea in the juvenile eyes. In a few cases of children examined by Margo, the trabecular meshwork and Schlemm’s canal were not seen. The anterior border of the iris extended anteriorly to the Descemet membrane, the internal sulcus being filled with iris tissue. Later in adult life, there is a complete closure of the iridocorneal angle by a slow and continuous process of contraction; this causes then the IOP rise and degenerative changes in optic nerve axons and to glaucoma [33].
6.4 Primary Open-Angle Glaucoma (POAG)
POAG is the most prevalent form of glaucoma with an incidence of 5–10 % in elderly people [34–36]. The incidence is greater in families, and the presence of genetic factors plays a role in the pathogenesis of POAG; 14 linkage loci have been identified and are designated as GLC1A through GLC1N [37] with myocilin, optineurin genes [38]. Gradual changes in the outflow capacities causes changes in eye pressure and slowly destructive effects on the optic nerve and retinal ganglion cells. In the normal-aging eye, the trabecular beams thicken as a result of thickening of the subendothelial basement membranes and changes of the extracellular matrix in the central core. There is also a continuous loss of cells within the corneoscleral and uveal part of the trabecular meshwork [39, 40] (Fig. 6.14).
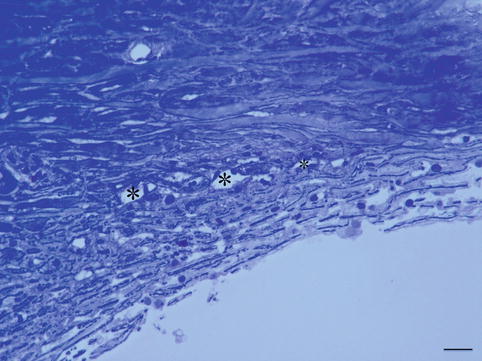
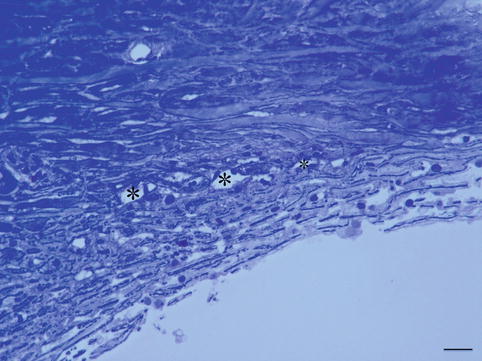
Fig. 6.14
Trabeculectomy specimen of a 79-year-old glaucoma patient. Trabecular meshwork is partially collapsed and contains less cells than normal. Schlemm’s canal is partially closed with partial loss of endothelial cells. Toluidine blue staining, bar = 50 μm
In the cribriform layer, the main change is the development of extracellular material appearing as plaques by long-spacing collagen accumulation. The deposition of plaque material continuously increases with age. These changes cause an increase in outflow resistance. Morphological changes of the outflow pathway in POAG are marked by an extreme exaggerated aging process. The trabecular meshwork endothelium cells diminish in number and the meshwork becomes thicker with diminished capacities of filtrating the aqueous humour [41–43]. The loss of cells causes a fusion of denuded beams and contributes to collapses of the intertrabecular spaces especially in the uveal meshwork. A significant increase of plaque-like material deposited within the cribriform layer and in the wall of Schlemm’s canal is noted compared to age-matched controls [44, 45]. Also, there is a reduction in the dimensions of Schlemm’s canal (Fig. 6.14) [46]. POAG is more prevalent and more severe in black people [47, 48], with more advanced histological changes in the TM and Schlemm’s canal [43, 49].
Optic nerve cupping is typical as described in section anatomy: there is a gradual decrease in nerve tissue fibres at the optic nerve head, with diminishing retinal ganglion cells.
6.5 Primary Angle-Closure Glaucoma (PACG)
6.5.1 Acute Primary Angle-Closure Glaucoma
The predisposing cause of PACG is the anatomy of the eye: a smaller eye with smaller axial length, typical an hyperopic eye, combined with a thicker lens due to aging. The lens and the iris touch each other and the normal flow through this slit is hampered. In these predisposing cases, a dilation of the pupil by darkness, stress or medication causes a thick plicated iris, in a small angle with blockage of the humour flow. If the pressure gradient between anterior and posterior chamber increases, the root of the iris is mechanically pushed against the cornea and trabecular meshwork, hindering the flow entering the TM. The secondary oedema of the iris and forward pushing of the iris raise the pressure very high to more than 60 mmHg in a very short time. This very high eye pressure pushes fluid in the corneal layers exceeding the pumping capacity of the corneal endothelial cells provoking corneal oedema resulting in hazy vision. Extension of the sclera causes extreme pain. The IOP can become higher than the arterial tension and interferes arterial blood entering the eye with ischemia as consequence. Only acute intervention with making a hole in the iris (iridectomy) and pressure lowering medication can stop the damage (Figs. 6.6 and 6.7). Consequences of the acute attack are sustained high IOP, uveal and optic nerve atrophy and cataract.
Light microscopy at the acute moment showed swelling of endothelial cells in the TM and separation of the cells obscuring trabecular beams and almost obliteration intertrabecular spaces; there is accumulation of pigment granules, red blood cells, macrophages, leucocytes and amorphous debris with widening and fusion of adjacent trabecular beams [50].
Light microscopy at a later stage shows fewer and narrower trabecular spaces and fusion of trabecular beams and loss of endothelial cells, with the remaining cells becoming polymorphic (Fig. 6.15a, b). In terminal cases, occlusion of Schlemm’s canal is seen [51]. The optic nerve shows thinning by atrophy without the typical cupping.
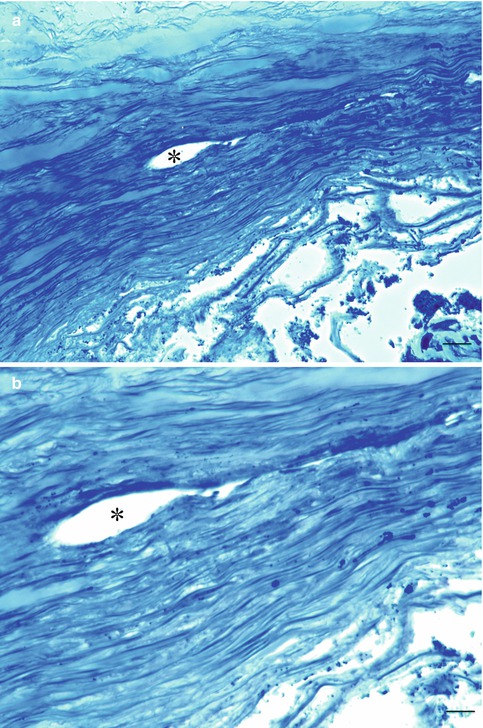
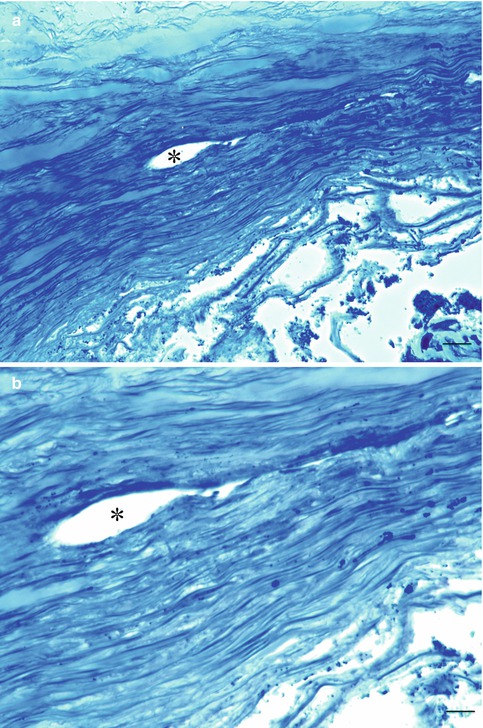
Fig. 6.15
(a, b) Trabeculectomy specimen of end-stage closed-angle glaucoma patient. Trabecular meshwork is totally collapsed without cells. Schlemm’s canal is partially closed with loss of all endothelial cells. Toluidine blue staining. Bars = 50 and 25 μm, resp
6.5.2 Chronic Angle-Closure Glaucoma
This term refers to an eye with a high IOP and with an anterior chamber which is narrow and closed in places by synechiae of the iris and peripheral cornea; these can be caused by an acute attack of chronic angle-closure glaucoma with reopening of the angle or due to intraocular inflammations. The prevalence is higher in East Asian compared to European people [52]. In chronic primary angle-closure glaucoma, TM showed a gross alteration of the architecture and arrangement of the trabecular beams, with fewer, narrower and more irregular trabecular spaces [50]. There is obliteration of Schlemm’s canal and fused beams denuded of endothelium cells with obliteration of endothelium cells [43].
Fan-shaped trabecular beams form peripheral anterior synechiae between the peripheral cornea and iris, together with endothelium cell loss [53].
6.6 Secondary Open-Angle Glaucoma
The secondary open-angle glaucoma with trabecular resistance to the aqueous outflow can be caused either by clogging of the trabecular meshwork by various particle types or by ultrastructural changes of the trabecular meshwork itself.
6.6.1 Pretrabecular
6.6.1.1 Neovascularisation Glaucoma
Neovascular glaucoma, a special form of secondary angle closure (pretrabecular), is caused by synechial angle closure by contraction of fibrovascular tissue. The aqueous outflow is impaired and results in elevation of IOP.
In the early stage, fibrovascular membrane may form on the anterior surface of the iris (neovascularisation of the iris (NVI) Fig. 6.16a–d). At this stage, the IOP is normal and the neovascularisation may regress. In the later state, the fibrovascular membrane grows over the trabecular meshwork and leads gradually to circumferential synechial angle closure; thus, in this stage, it should be classified as secondary angle-closure glaucoma (see below) [54]. The corneal endothelium may then be stimulated to proliferate and migrate over the false angle. A contraction of the membrane on the iris surface causes an anterior bowling of sphincter and iris pigment epithelium (ectropium uveae, Fig. 6.16d) and can lead to an irregular dilatation of the pupil [55].
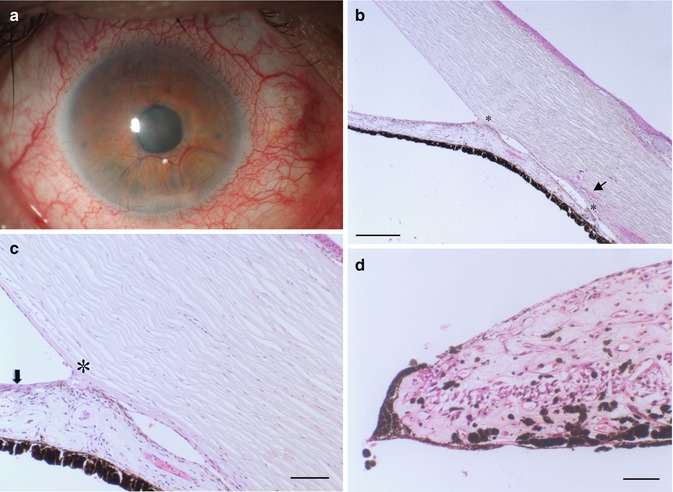
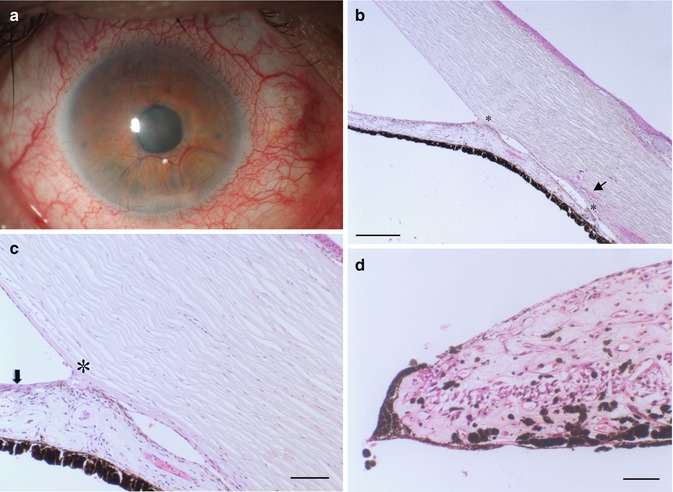
Fig. 6.16
(a) NVI with intense neovascularisation at the iris and lens surface. (b) Anterior synechiae (asterisks) forming in NVI, Schlemm’s canal is narrowed (arrow), HE, bar = 100 μm. (c) Fibrovascular membrane at the anterior surface of the iris containing tiny neovascularisation vessels (arrow), anterior synechia (asterisk) HE, bar = 50 μm. (d) Ectropion uveae with anterior displacement of the pigment epithelium due to traction of fibrovascular membrane at the iris surface, HE. Bar = 50 μm
Neovascularisation occurs when larger segments of the retina lack adequate perfusion. It has been postulated that the ischemic retina produces angiogenic factors, especially VEGF that enters the anterior chamber triggering the growth of new blood vessels on the retina and on the iris surface as well. This is a dreaded complication of ischemic central retinal vein occlusion, proliferative diabetic retinopathy, chronic intraocular inflammation and intraocular tumours.
Management during the late state of neovascular glaucoma is very difficult. To prevent this complication or progression of early-detected NVI, an adequate treatment of the underlying cause of posterior segment hypoxia (panretinal photocoagulation) is mandatory [56].
6.6.1.2 Endothelial Layer
Iridocorneal Endothelial Syndrome
Iridocorneal endothelial syndrome (ICE) includes progressive essential iris atrophy, Cogan-Reese syndrome or iris nevus syndrome, Chandler’s syndrome and mixed forms [55]. This very rare disease typically occurs unilateral in middle-aged women. The aetiology is still unknown, but an abnormal individual reaction to a virus infection of the herpes genus (herpes simplex, Epstein-Barr) has been proposed [57, 58]. Different names are given for a wide spectrum of the same disease with different clinical characteristics and common underlying pathogenic mechanism.
Migration of an abnormal corneal endothelial cell layer across the anterior chamber angle and at the anterior surface of the iris is responsible for corneal oedema, secondary glaucoma, noduli and atrophy of the iris and pupillary distortion (Fig. 6.17a). The contraction of the migrated membrane-like ICE tissue produces holes in the iris, usually unilateral. In young patients, glaucoma and oedema of the cornea are the main therapeutic problems.
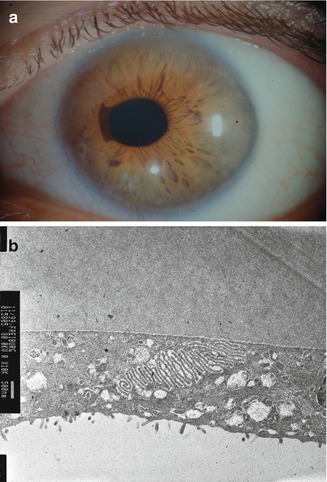
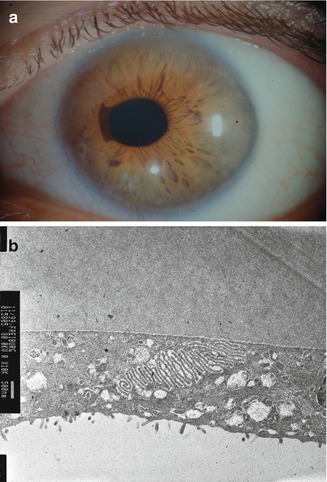
Fig. 6.17
(a) Corectopia of the pupil in iridocorneal syndrome, peripheral corneal opacification due to oedema. (b) Microvilli of corneal endothelial cells (epithelialisation) in iridocorneal syndrome of a 45-year-old patient
Histopathology shows a monolayer of endothelial-like cells with Descemet-like membrane which covers the anterior chamber angle and the anterior border of the iris. This membrane is contracting and causing closure of the angle; on the iris surface, it causes holes in the iris stroma [59–61]. The diseased endothelial layer gets epithelial characteristics with cellular proliferation [62]. Progress over time is going through any degree of change from normal iris through marked corectopia and hole formation.
6.6.2 Trabecular
6.6.2.1 Degenerative
Two degenerative disorders causing trabecular problems are the pseudoexfoliatio lentis and the pigmentary glaucoma.
Pseudoexfoliation Glaucoma
The name pseudoexfoliatio derives from the fact that the dandruff-like material deposited on the lens reminded on heat-associated genuine exfoliation of the anterior aspect of the lens (Fig. 6.18). The cause is not known; there are hints that the disease is inherited (see below). The pseudoexfoliation material is composed by various glycoproteins forming a fibrillary protein coated by amorphous material and is also found elsewhere in the body like the skin, lungs, heart, liver, kidney and the cerebral meninges. Intraocularly, it seems to be produced by endothelial cells of the trabecular meshwork, lens epithelium, ciliary body epithelium or iris cells. The pseudoexfoliation material clogs the trabecular meshwork and is phagocytosed by the trabecular meshwork endothelial cells and thus causes increase of the outflow resistance (Fig. 6.19). Pseudoexfoliation is associated with vascular abnormalities of the iris with deposition of the material within the iris vessels and increased pigment loss causing pupillary ruff atrophy and additional clogging of the trabecular meshwork by the pigment particles. Mydriasis can cause extensive pigment liberation from the histologically sawtooth-like configurated pigment epithelium at the posterior side of the iris (Fig. 6.19) causing hyperpigmentation of the trabecular meshwork and possibly also IOP elevation in many cases. Pseudoexfoliation is not confined to the trabecular meshwork but is also associated with changes of the cornea, ciliary body (Fig. 6.19) and lamina cribrosa. The cornea shows less epithelial and endothelial cells, less subbasal nerve plexus causing decreased corneal sensitivity and increased corneal morbidity, e.g. after cataract surgery [63]. It is known that eyes with pseudoexfoliatio lentis are more susceptible to glaucoma damage in case the intraocular pressure rises. The morphological correlate was shown by Schlötzer-Schrehardt et al. demonstrating a pseudoexfoliatio specific elastinopathy of the lamina cribrosa with decreased expression of LOXL1 protein and decreased amount of elastic fibres. It was also shown in vitro that the assembly of elastic fibres by the optic nerve head astrocytes was impaired by the inhibition of LOXL1 [64]. Single-nucleotide polymorphisms of the LOXL1 gene are known to increase the susceptibility for pseudoexfoliation [65]; hints towards association with other genes like clusterin, adenosine receptor A3, matrix metalloproteinases, glutathione transferase, contactin-associated protein-like 2 and tumour necrosis factor alpha gene [66] are found though not associated with the strong correlation shown by LOXL1. In contrast to POAG, the optic nerve in pseudoexfoliation glaucoma does not show increased connective tissue in the septa [67].
