CHAPTER 179 Stereotactic Radiation Treatment of Benign Tumors of the Cranial Base
Since the late 1990s, stereotactic radiation therapy has become an increasingly popular treatment for benign tumors of the cranial base, including vestibular schwannomas. After its introduction for clinical use in 1967 by Lars Leksell, the initial acceptance of this modality waned as early complications included brainstem radiation damage, hydrocephalus, and cranial neuropathies.1 However, as delivery has become more precise through improved stereotactic guidance, and as the tolerance levels of cranial nerves for radiation have become better elucidated, the technique has gained popularity and now offers an alternative to surgical intervention or observation for many cranial base tumors. Additionally, the combined use of radiation and surgical treatment may be considered for tumors for which incomplete excision may be planned to reduce the danger to adjacent cranial nerves. The goal of stereotactic irradiation for the treatment of benign tumors generally is considered to be growth arrest.
Principles of Stereotactic Radiation Therapy
With gamma knife radiotherapy techniques, well-collimated beams of radiation from 201 cobalt-60 sources are directed through ports in a semispherical helmet, allowing the beams to converge and deliver a much higher dose to the target than to the surrounding structures. The precise delivery of the beam is increased by direct fixation of a head frame attached with surgical pins to the cranium (Fig. 179-1). Although the procedure commonly is referred to as stereotactic “radiosurgery,” this is a misnomer, because the therapy is accomplished by the delivery of external radiation without a surgical incision. Today most teams are composed of neurosurgeons or neuro-otologic surgeons, a radiation oncologist, and a radiation biologist. The advantage of gamma knife techniques at present is the capability for highly controlled delivery of radiation by way of multiple isopoints to the tumor target. Additionally, newer units have more fully automated coordinate adjustments, allowing more rapid dosing. A disadvantage is that tumors located much below the level of the cranial base are not readily accessible for stereotactic radiation delivery by the gamma knife. Rigid fixation of the skull to a metal frame that locks into the radiation chamber allows precise, submillimeter stereotactic radiation delivery, but the gamma knife does not lend itself to multiple fractionated treatments.2
An alternative to the gamma knife was developed through the application of linear accelerators (LINACs). Instead of multiple sources aimed at a central designated target, the LINACs rotate the treatment beam of the unit around the target in a variable number of rotational arcs.3 Although the tumor margin doses are similar to those achieved with the gamma knife, LINAC delivery typically uses fewer isocenters, and the dose to the tumor may be less precisely applied. Thermoplastic molded mask systems to limit positional variation in the radiation delivery allow for the potential of fractionated schedules without the placement of pins into the skull but may not restrict movement of the target as surely as rigid fixation. Controversy exists regarding the potential benefit of fractionated stereotactic radiation protocols. Some investigators maintain that a fractionated regimen, given over days or weeks, takes advantage of radiobiologic principles to reduce late toxicity while maintaining tumor control. However, in the case of vestibular schwannomas, both the tumor and the surrounding cranial nerves are late-reacting tissues; therefore, fractionating theoretically reduces the post-irradiation complications to the surrounding cranial nerves but also reduces the likelihood of desired tumor damage, DNA disruption, and apoptotic cell death.
Radiobiology
Protons are heavy charged particles that cause damage directly. They are positively charged and of a much higher mass than electrons. When a proton beam strikes its target, it delivers almost all of its energy at its delivery range, which results in a rapid dropoff of energy level, thereby limiting tissue damage past the target of interest. This characteristic is exploited to deliver a high dose of radiation directly to the target tissues with sparing of tissues deep to the delivery area. Dose inhomogeneity may increase the risks of complications for large target volumes treated with stereotactic radiation when the target area is less than a perfect match for the radiation delivery field. Complications were fewer with conformal multiple-isocenter gamma knife treatment plans than with less elaborate two-isocenter LINAC stereotactic radiation plans, which were associated with a higher risk of complications, with possible high-dose overlap regions extending into normal tissues.4 The newer, more precise delivery systems are less likely to expose tissues out of the target regions to high doses of radiation.
Dose Selection
Dose selection in stereotactic radiotherapy has been evolving over the past decade as experience has been gained. Early animal studies by Kondziolka and associates4a demonstrated that xenograft vestibular schwannomas in the renal capsule of athymic mice showed significant reductions in tumor volume following stereotactic radiation with 40 Gy at 2 weeks and after 1 month in a 20-Gy group. Tumor vascularity was reduced in the 20- and 40-Gy groups respectively, but not in the 10-Gy group at 3-month follow-up evaluation. In humans today, however, in order to decrease facial nerve neuropathies, 17 Gy or less is delivered to the 50% isodose line (Fig. 179-2). Also, the auditory portion of the eighth cranial nerve appears to have a tolerance for approximately 11 Gy, with an 50% hearing preservation rate.4b Simply put, the lower the dose, the lower the complication rate—but the lower the likelihood of tumor control over the long-term follow-up period of observation.
Benign Tumors of the Cranial Base: Vestibular Schwannoma
Vestibular schwannomas have increasingly been treated with stereotactic radiation. Some centers favor this therapy over conventional surgical techniques or observation. In reporting the outcomes with gamma knife, LINAC, and fractionated stereotactic irradiation, “tumor control” has been defined in two ways. Some investigators define tumor control as no growth, whereas others report the percentage of tumors that required no further treatment. The latter definition may overestimate the true control rate, because cases in which tumors may have been growing but not requiring surgical intervention would be counted as those with successful outcomes. The most useful information that can be gleaned from the literature at this time is a compilation of reported outcomes. The lack of well-controlled studies has been duly noted. The longer the follow-up, the more valuable the study. Outcomes data extracted from the recent literature, including reports on tumor control, facial nerve function, hearing preservation, and trigeminal neuralgia, are summarized in Tables 179-1 to 179-3.
Fractionation versus Nonfractionation
Considerable debate is ongoing regarding whether or not fractionated stereotactic radiation is more favorable than single-dose stereotactic radiation for cranial nerve functional preservation in vestibular schwannoma treatment. Although fractionated radiation may give less immediate damage to the surrounding cranial nerves, damage to the slowly growing schwannoma cells also is less likely, thereby increasing the likelihood of growth. Flickinger4c argues that the available data in the literature are simply inadequate to allow definitive conclusions regarding which of the two delivery plans is better for cranial nerve preservation and tumor control; carefully planned, prospective controlled longitudinal studies are needed to address this concern. Indeed, the data shown in Tables 179-1 to 179-3 seem to confirm his opinion.
Traditionally, in our own practice, stereotactic radiation has been reserved for a select patient subset for the treatment of vestibular schwannoma. Elderly patients with small to medium-sized tumors with proven growth or those not fit for a surgical resection have been recommended to receive stereotactic radiation treatment. Chen, reporting data for three 5-year periods between 1990 and 2005, noted that vestibular schwannomas were being diagnosed at statistically higher ages, at smaller sizes, and in a greater proportion of patients older than 65 years of age.5 During these periods, the frequency of observation as a treatment choice for vestibular schwannoma rose from 12% to 37%, microsurgery was performed in 59% of patients, and frequency of use of stereotactic radiation therapy rose to 4% of cases. As life expectancy is increasing, it seems likely that vestibular schwannomas under expectant management will be more likely to show growth when observed over longer periods of time, as demonstrated by Charabi and associates.6 Smouha and colleagues, in a retrospective meta-analysis of patients managed without intervention, demonstrated that 43% of 1244 vestibular schwannomas showed growth over a mean follow-up period of 3.2 years.7 Hearing loss occurred in 51% of 347 patients. Only 20% of 1001 patients from 15 studies eventually failed observation, showing growth sufficient to warrant active treatment. Smouha’s data are similar to our own prospective findings, which demonstrated that 66% of tumors grew more than 15% when measured volumetrically after a mean follow-up period of 3.8 years.8
Outcome Assessment
Because stereotactic radiation treatment of vestibular schwannomas is still evolving, results are not easily comparable on a study-to-study basis, nor are they easily grouped to add power to institutional reporting by meta-analysis. Dosing parameters have changed over time and differ among patients, among doctors, and among institutions within studies.9–11 This variability in retrospective studies is problematic, making optimal dosing parameters difficult to discern. Dose planning is enhanced with improved imaging shot selection, and blocking strategies that safeguard neural structures. Automation in delivery systems and better understanding of the biologic effect of radiation also should result in improved outcomes.10,11
After irradiation, tumors show signs of treatment effect by loss of central enhancement within the first 6 to 12 months.12,13 Approximately one fourth (23%) will show expansion, or transient tumor swelling (on average 2 to 4 mm but as much as 10 mm) generally 6 to 24 months after treatment, but swelling can be seen up to 5 years after treatment. This temporary expansion may take 6 months to 5 years to resolve.2 Fifty percent of these tumors will undergo a loss of central contrast enhancement that may correlate histopathologically with tumor necrosis.14 Eventually, residual tumors show return of enhancement and possibly further shrinkage. Few show peritumoral edema. More recent studies recognize these signs of radiation effect, and continued observation often validates eventual stability or regression.2 This understanding alone has prevented surgery for what was thought to be tumor progression in earlier studies and is manifested in improved control rates in newer data. Tumors that were excised, possibly for mistaken post-irradiation progression, have shown histopathologic evidence of radiation-induced vascular changes, including intimal thickening, perivascular inflammation, and focal hemorrhage.14 Profuse interstitial fibrin deposits also were seen. Schwannomas surgically removed after stereotactic radiation failure also have shown typical and viable schwannoma cells with increased vascularity, as well as other foci showing delayed radiation changes such as nucleolar and cytoplasmic enlargement, proliferation of endothelial cells, and necrosis.15–17
In 2006, Weil and colleagues published a thorough review of the English language literature regarding single-fraction stereotactic radiation.10 Similarly, Backous and Pham and Likhterov and associates reviewed the current literature and summarized the stereotactic irradiation data, including outcomes of fractionated stereotactic radiation protocols, through 2005.2,3 Tables 179-2 and 179-3 present outcomes data from these reviews, along with those from the most recent studies on stereotactic irradiation.10,12,14,15,18–39 Studies with fewer than 15 patients or less than 12 months of follow-up were excluded.
Hearing Outcomes
Hearing outcomes have steadily improved since the early 1980s as a result of decreasing marginal doses, improved conformality and shielding of important structures, or better patient selection, or a combination of these factors. Dose tolerances of the cochlea, cochlear nerve, and cochlear nucleus are still under investigation.2,22,33 In a prospective follow-up study of 34 patients, Thomas and colleagues found the cochlear dose to be the only variable that was statistically predictive of hearing preservation.33
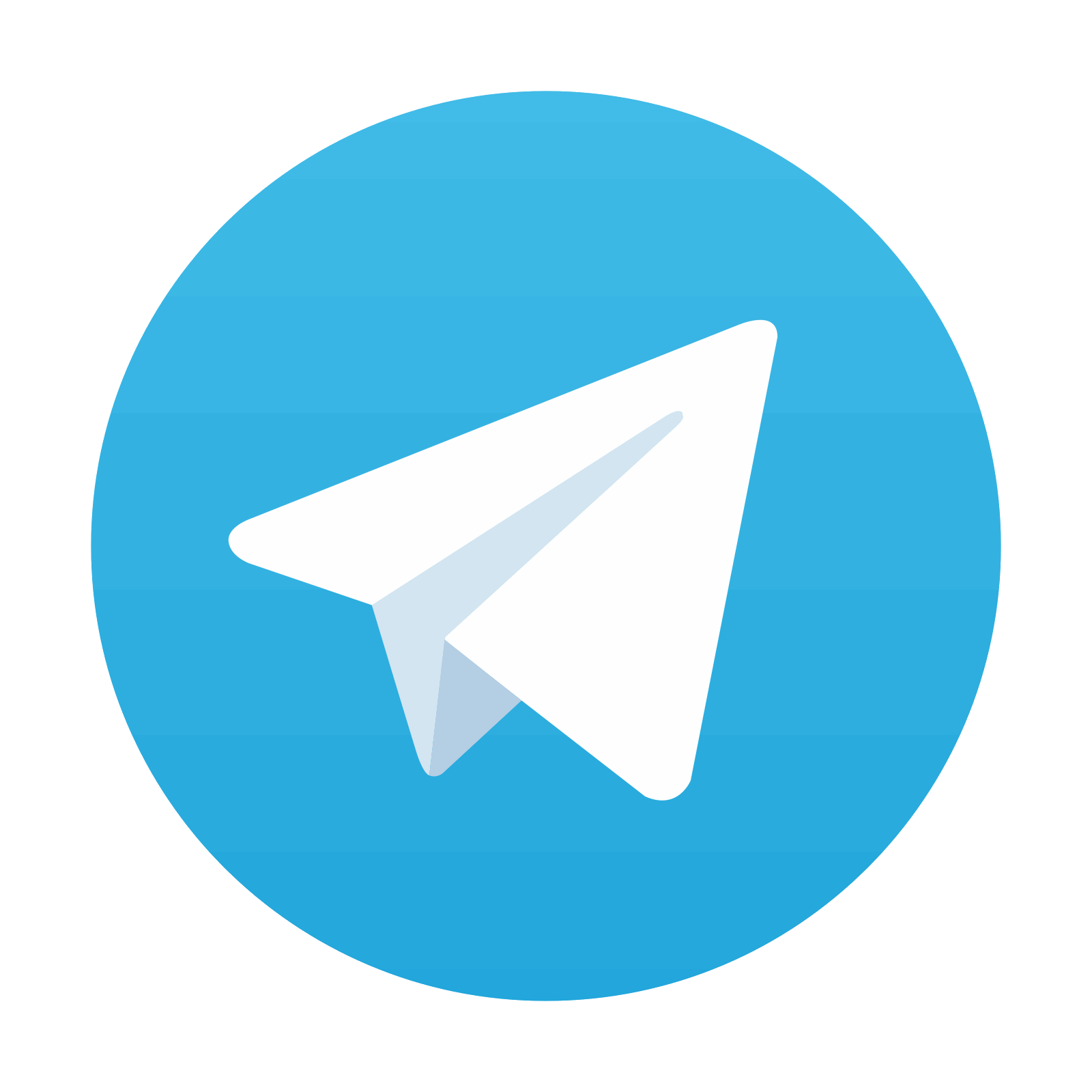
Stay updated, free articles. Join our Telegram channel

Full access? Get Clinical Tree
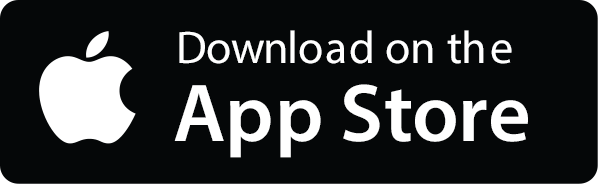
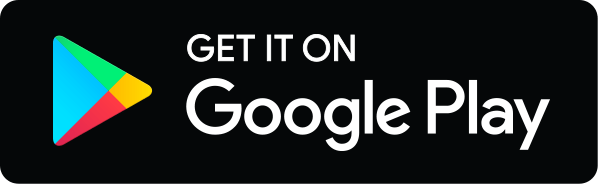