Cell type/preparation
Auditory neural markers detected
Electrical activity detected ✓ or ✕
New synapses detected ✓ or ✕
References
Embryonic stem cells
HESC
Pax2, Brn3a, GATA3, βIII tubulin, peripherin
✕
✓ Synapsin +ve new synapses on hair cells
Shi et al. (2007)
HESC
Pax2, Sox2, NeuroD1, Islet1, Brn3a, GATA3, NF, peripherin, VGLUT1
✕
✓ Synapsin1 +ve new synapses on hair cells and cochlear nucleus neurons
HESC
Pax2/8, Sox2, Six1, NeuroD1, Islet1, Brn3a, GATA3, βIII tubulin, NF, NTRK2
✓
✓ New synapses detected in vivo
Chen et al. (2012)
HESC
Pax2, Brn3a, peripherin, NF
✓
✓ Synapsin1 +ve new synapses on hair cells
Nayagam et al. (2013)
HESC
NeuroD1, Brn3a, VGLUT1, NFM,
✓
Not examined
Needham et al. (2014)
Induced pluripotent stem cells
HIPSC
Pax2/7, Sox2, NeuroD1, Islet1, Brn3a, GATA3, NF, peripherin, VGLUT1
✓
✓ Synapsin1 +ve new synapses on hair cells
Gunewardene et al. (2014)
Inner ear stem cells
IESC
Pax2, Brn3a, GATA3, Islet, peripherin, calretinin, NTRK2/3
✓
Processes extended to hair cells
Martinez-Monedero et al. (2008)
HFASC
Pax2, Sox2, Ngn1, Nestin, Brn3a/c, GATA3, βIII tubulin
✕
✕
Chen et al. (2007)
HFASC
Pax2, Sox2, Ngn1, Nestin, Brn3a/c, GATA3, βIII tubulin, NF
✓
✕
Chen et al. (2009)
Mesenchymal stem cells
HMSC
Nestin, Tuj-1
✕
✕
Bas et al. (2013)
9.3.1 Directed Differentiation of Stem Cells to Auditory Neurons
Directed differentiation of cells toward an auditory neural-like lineage has taken two approaches; either the application of soluble factors in the media or the genetic modification of stem cells to express relevant transcription factors or proteins essential for normal auditory neural development. Soluble factors have included retinoic acid (Coleman et al., 2007a), bone morphogenetic proteins (Shi et al., 2007; Nayagam et al., 2013), basic fibroblast growth factor, insulin-like growth factor 1 and epidermal growth factor (Chen et al., 2007, 2009), fibroblast growth factors 3 and 10 (Chen et al., 2012), conditioned medium (Coleman et al., 2007a; Kondo et al., 2011), or small molecules such as Y27632 (Nayagam et al., 2013; Gunewardene et al., 2014). Alternatively, the forced expression of transcription factors relevant for auditory neural differentiation such as Ngn1 (Reyes et al., 2008; Purcell et al., 2013) and Tlx3 (Kondo et al., 2011) has generated sensory neurons from mouse embryonic and human mesenchymal stem cells, respectively. Although the aforementioned protocols can produce auditory-like neurons, it has been challenging to derive this particular cell population conclusively in the absence of a single specific marker to define the lineage. As a result, these studies have relied on groups of relevant markers to characterize the lineage, together with electrophysiological characterization of ion channels underlying their electrical activity.
More specifically, auditory-like neurons derived from embryonic stem cells have been reported to express several characteristic proteins and transcription factors to their endogenous counterparts including (but not limited to) Ngn1, NeuroD1, Brn3a, GATA3, and neurofilament. A summary of key findings in relation to this is provided in Table 9.1. Although these cells cannot be defined as auditory neurons, in many instances they show a bipolar morphology and neurosensory protein and/or transcription factor expression (Shi et al., 2007; Chen et al., 2012; Nayagam et al., 2013). Ultimately, these auditory-like sensory neurons could provide a source for functionally relevant replacement of cells in a deaf ear.
Recent experiments have shown that human and mouse embryonic stem cells after appropriate differentiation function as replacement cells in the auditory system (refer to Tables 9.1 and 9.2). The use of induced pluripotent stem cells has opened a new avenue of investigation for pluripotent stem cell research, given that these cells facilitate the derivation of patient-specific cells for transplantation. Initial experiments differentiated murine-derived iPSC lines into a neural lineage using stromal cell coculture (Nishimura et al., 2009, 2012). This culturing method has been used to produce dopaminergic neurons from the midbrain (Kawasaki et al., 2000, 2002). More recent experimentation has compared the efficiency of deriving neurosensory progenitors from several human iPSC lines using noggin, epidermal growth factor, basic fibroblast growth factor, and the small molecule and Rho kinase inhibitor Y27634. Human iPSC lines were shown to express sequentially many of the key proteins and transcription factors found in the differentiating otic placode and auditory neurons in situ, including (but not limited to) NeuroD1, Islet1, Brn3a, GATA3, neurofilament, and VGLUT1 (Gunewardene et al., 2014). Although the expression of these markers was more variable in the human iPSC lines examined (in comparison to human embryonic stem cells grown under the same conditions), these data illustrated that auditory neuron-like cells could be generated from several human iPSC lines. How these progenitors survive and integrate into the deaf ear remains to be demonstrated.
Table 9.2
Summary of stem cell transplantation studies for auditory neural replacement
Cell type/preparation | Cell survival time (weeks) | Reference |
---|---|---|
Mouse stem cells | ||
Mouse ESCs | 1–14 | |
Mouse IPSCs | 1–4 | |
Mouse ASCs/MSC | 1–4 | |
Mouse NSCs | 1–9 | |
Rat stem cells | ||
Rat NSCs | 2–9 | |
Guinea pig stem cells | ||
Guinea pig ASCs | 4 | Ogita et al. (2010) |
Chinchilla stem cells | ||
Chinchilla ASCs | 3 | Naito et al. (2004) |
Human stem cells | ||
Human ESCs | 8.6–10 | |
Human ASCs/MSCs | 2–8.5 |
Mesenchymal stem cells, have been differentiated into auditory-like sensory neurons that express a number of relevant genes including Ngn1, NeuroD1, Brn3a, GATA3, and GluR4 (Kondo et al., 2011). Similar to iPSCs, mesenchymal stem cells are a potential source of autologous donor cells capable of avoiding issues of immune rejection. In addition to deriving cells with an appropriate phenotype, replacement neurons would also need specific physiological characteristics, and auditory-like neurons derived from mesenchymal stem cells have been shown to possess similar markers but not electrophysiological properties of auditory neurons.
9.3.2 Are Stem Cell-Derived Auditory Neurons Electrically Active?
In addition to their characteristic biochemistry, the primary auditory neurons of the cochlea are glutamatergic and capable of firing at high rates. The spike rate in response to acoustic stimulation is on the order of 200–400 spikes/s (Kiang et al., 1965; Javel & Viemeister, 2000); however, rates up to 1000 spikes/s are evinced by electrical stimulation (Shepherd & Javel, 1997; Javel & Viemeister, 2000). This raises important considerations for any cell type that may be used in a cell replacement therapy, including the following: Are stem cell-derived neurons electrically active? Do they possess an appropriate compliment of ion channels? Can they fire action potentials? Will they respond to the high stimulus rate provided by the cochlear implant?
A smaller number of studies have investigated the ability of stem cell-derived auditory-like neurons to generate action potentials in response to intracellular current injection (Martinez-Monedero et al., 2008; Nayagam et al., 2013; Purcell et al., 2013). These studies have illustrated that stem cell-derived auditory-like neurons possess key potassium and sodium currents necessary for neural communication. Among these are the inward sodium currents (I Na) and sustained outward potassium currents (I K). These are arguably the most basic currents necessary to instigate action potentials, and therefore communicate meaningful signals to their target(s).
Although capable of basic electrical communication, stem cell-derived auditory- like neurons do not fire action potentials with the same efficiency as adult mammalian auditory neurons (Needham et al., 2014). Their firing profile resembles that of mammalian embryonic auditory neurons in situ (Marrs & Spirou, 2012), with increased latency and broader action potentials when compared to early postnatal mammalian auditory neurons (Needham et al., 2014). Despite extended culture in vitro, these stem cell-derived auditory-like neurons did not mature into more electrically mature phenotypes, suggesting that additional factors, and potentially synapse formation, might be necessary to reach functional maturity. This idea is consistent with other reports that detail the importance of synaptogenesis between hair cells and auditory neurons (and thus spontaneous activity), in producing electrically mature auditory neurons in situ (Lippe, 1994; Marrs & Spirou, 2012). These findings set the stage for new in vitro models that incorporate both differentiation and synapse formation to determine whether more physiologically mature auditory-like neurons can be produced. An important additional feature of auditory neural physiology is the ability to respond at high rates to process sound information. This has been investigated recently with interesting findings (Needham et al., 2014).
The afferent neurons of the auditory system can follow high-frequency stimulation, as well as the electrically encoded input from a cochlear implant. Pitch discrimination deteriorates as stimulation levels approach 300 pulses per second (Shannon, 1983; Zeng, 2002; Vandali et al., 2013). Although human stem cell-derived auditory-like neurons can reliably entrain to pulsatile stimulation rates of approximately 20 pulses per second, firing entrainment fell to 50 % at approximately 50 pulses per second. Moreover, entrainment of stem cell-derived neurons became statistically poorer than auditory neurons at stimulation rates of 67 pulses per second. A reduction in firing entrainment would likely affect the amount of information encoded in the signal. Thus, an effective auditory neural replacement strategy, irrespective of which stem cell type was ultimately chosen, would likely incorporate the transplantation of functional neurosensory progenitors that were capable of firing entrainment of 300 pulses per second.
9.3.3 Can Stem Cell-Derived Auditory Neurons Make Functional Connections on Appropriate Tissues In Vitro?
As adult spiral ganglion neurons do not appear to regrow and form synapses with hair cells, assays for afferent synaptogenesis have been developed using newborn cochlear tissues where regrowth can be studied (Flores-Otero et al., 2007; Nayagam et al., 2013; Brugeaud et al., 2014). Synaptogenesis has been studied using both stem cell-derived neurons (Matsumoto et al., 2008; Nayagam et al., 2013) and newborn spiral ganglion neurons (Flores-Otero et al., 2007; Brugeaud et al., 2014; Tong et al., 2013). In vitro synaptogenesis assays have examined whether the peripheral and central tissues of the postnatal auditory system are reinnervated by stem cell-derived auditory neurons.
Synaptogenesis has been examined after toxin-induced or mechanical denervation of the organ of Corti (Martinez-Monedero et al., 2006; Flores-Otero et al., 2007). Mouse spiral ganglion neurons grew to the denervated hair cells and formed synapses that were immunopositive for the synaptic marker, synapsin (Martinez-Monedero et al., 2006). Terminals formed by stem cell-derived neurons were similar to those formed by the spiral ganglion neurons (Fig. 9.1b; Flores-Otero et al., 2007; Martinez-Monedero et al., 2008; Nayagam et al., 2013): Human stem cell-derived auditory-like neurons extended processes toward the hair cells where they formed synapsin-positive synapses (Shi et al., 2007; Nayagam et al., 2013; Gunewardene et al., 2014); neurons derived from mouse embryonic stem cells formed synapsin- and/or synaptophysin-positive terminals with hair cells after 7 days in vitro (Matsumoto et al., 2005, 2008). The staining of presynaptic markers in the neurons suggests that the polarity of the synapse was initially reversed (Martinez-Monedero et al., 2006). Importantly, the pattern of innervation between stem cell-derived neurons and hair cells is disorganized and new synapses are en passant-like, rather than bouton-like in ultrastructure (Fig. 9.1e; Nayagam et al., 2013). Rewiring of hair cells will need to be carefully controlled to regenerate a functional system, which may be possible by the careful application of guidance molecules (refer to Sect. 9.5).
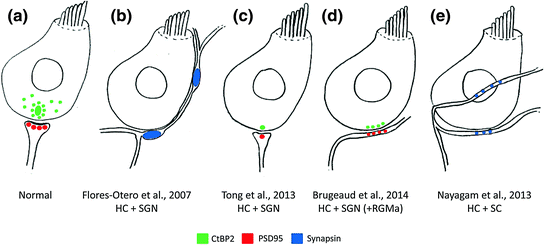
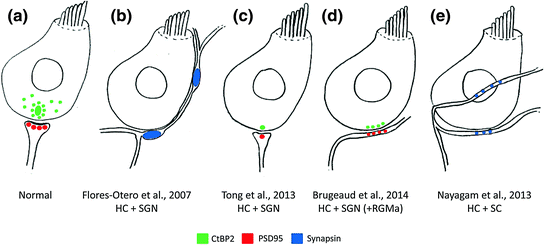
Fig. 9.1
Schematic overview of in vitro auditory synaptogenesis assays and their major findings. a Normal afferent innervation in the mammalian cochlea illustrating close association between hair cell (HC) CtBP2-positive (green) ribbon synapses and PSD95 positive (red) afferent terminal from type I spiral ganglion neurons (SGN). b Following denervation, early postnatal SGNs reinnervated HCs and their neurites made large, putative, presynaptic (synapsin-positive; blue) terminals on contact with the HCs. c Using the same experimental design as (b), single, correctly opposed synapses were observed between HCs (CtBP2-positive puncta, green) and SGNs (PSD95-positive puncta, red). d The reinnervation described in C was enhanced by the application of RGMa to the cell culture media, to give greater numbers of new, correctly opposed synaptic connections. e Experimentation using the same in vitro model, but replacing SGNs with stem cell (SC)-derived neurons, showed extensive reinnervation of HCs by SC-derived neurites as illustrated by synapsin-positive puncta (blue) at sites of HC innervation. CtBP2, C-terminal binding protein 2; HC, hair cell; SC, stem cell; SGN, spiral ganglion neuron; PSD95, postsynaptic density protein 95; RGMa, repulsive guidance molecule for retinal axons
Although these initial reports of synaptogenesis are promising, it will be important to demonstrate that these apparently presynaptic terminals ultimately mature into postsynaptic densities. Small numbers of postsynaptic densities have been reported in recent experimentation using human embryonic stem cells (Nayagam et al., 2013). These postsynaptic densities need to be correctly aligned with hair cell ribbon synapses for fast neural transmission. Early postnatal auditory neurons developed postsynaptic densities that stained for PSD95 (Fig. 9.1c, d; Tong et al., 2013; Brugeaud et al., 2014). Functionally immature stem cell-derived neurons (Nayagam et al., 2013; Needham et al., 2014) may not be capable of making mature synapses with hair cells with the same propensity as early postnatal auditory neurons, which are already specified and fully differentiated (Tong et al., 2013; Brugeaud et al., 2014). The afferent synapse may mature once connections are made to hair cells and may be assisted by the application of appropriate soluble guidance molecules, as recently reported (Brugeaud et al., 2014; see also Sect. 9.5).
In addition to peripheral innervation, a smaller number of central innervation assays have been used to examine whether stem cell-derived neurons form synapses with neurons in slices of developing mammalian cochlear nucleus (Glavaski-Joksimovic et al., 2009; Hyakumura et al., 2012). A study using murine embryonic stem cell-derived neurons reported the presence of synaptic vesicles in stem cell processes contacting the edge of the auditory brain stem slice. More recently, auditory-like neurons derived from human embryonic stem cells were found to innervate the auditory brain stem extensively and to make synapsin1-positive glutamatergic terminals on neurons in the cochlear nucleus. Like their peripheral counterparts, central processes must form appropriate tonotopic connections with neurons located in the anatomically correct regions of the brain stem. To date, there are no published in vitro or in vivo studies describing the cochleotopic innervation of the cochlear nucleus by stem cells. Given the difficulty in tracing central stem cell-derived neural processes in vivo, assays for central innervation may prove useful in screening different stem cell types (and different stages of differentiation), in addition to various compounds and guidance molecules for their ability to improve brain stem innervation.
9.4 The Current Status of Stem Cell Transplantation for Replacement of Auditory Neurons in the Deafened Ear
Although it is promising to be able to generate appropriate and functional sensory progenitors in vitro, the ultimate challenge in stem cell therapy for hearing loss is the successful delivery and functional integration of the progenitors with residual elements in the deafened ear. Substantial loss of auditory neurons is often found in ears that are profoundly deaf. If hair cells are severely depleted, stem cell therapy could potentially be combined with a cochlear implant. Alternatively, if hair cells are intact, as in auditory neuropathy, stem cell-derived neurons might replace the link between the sensory organ and the brain stem. In either case, challenges remain because the transplantation of stem cells into the inner ear requires precise surgery to reach the appropriate anatomical compartments (the modiolus or auditory nerve). In addition, stem cell-derived neural processes must grow or be guided peripherally toward residual hair cells and centrally into the brain stem. These new neurons must also be capable of synaptogenesis in the periphery and the brain stem, if they are to recapitulate the tonotopic wiring of the cochlea and cochlear nucleus long term. When combined with a cochlear implant, stem cell-derived neurons must respond to high stimulation rates (Needham et al., 2014). As described in Sects. 9.3.1 and 9.3.3, substantial progress toward these challenges has been made in vitro. Additional advances have been described in vivo over the last decade, and key findings from these studies are described in detail in the following section.
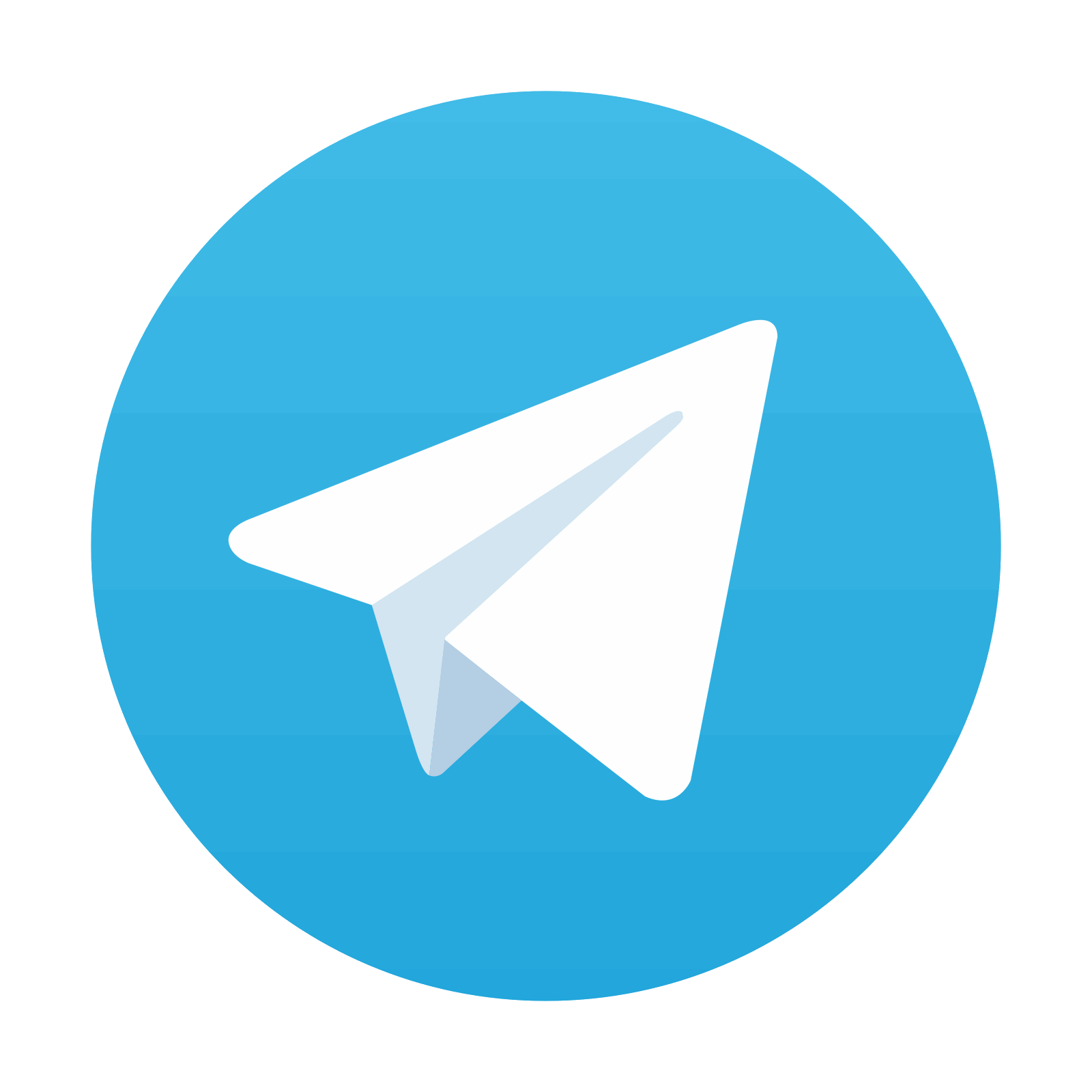
Stay updated, free articles. Join our Telegram channel

Full access? Get Clinical Tree
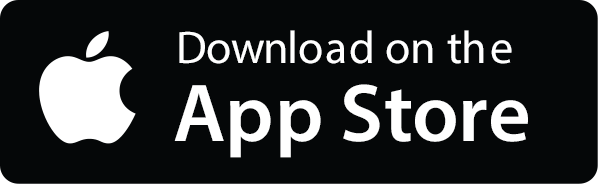
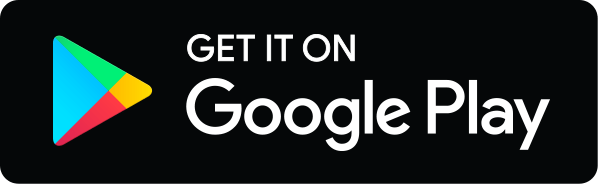