Author
No. eyes
Regimen
Cycles
V eyes
EBR
Enuc
None
F/U (months)
Gallie [9]
40
VRES
18
4
1
13
3
Kingston [26]
24
VRE
2–4
24
20
6
18
60
Murphree [17]
35
VRE
3
21
7
17
0
?
Shields [32]
31
VRE
2
22
9
0
13
6
Greenwald [18]
11
RE
6–7
6
5
3
1
23
Bornfeld [11]
12
VRES
3
7
2
1
4
7
Shields [27]
52
VRE
2.6
36
19
8
9
17
Gunduz [14]
27
VRE
2.6
27
16
10
5
25
Friedman [13]
75
VRE
6
30
13
9
14
13
Beck [10]
33
RE
2–5
13
7
5
2
31
Wilson [20]
36
VR
6
14
8
5
5
19
Shields [29]
158
VRE
6
75
32
32
27
28
Brichard [12]
24
VRE
2–6
12
0
2
10
21
Rodriguez [21]
43
VR
8
15
8
6
4
32
Schiavetti [19]
58
RE
4–8
17
4
11
1
53
Antoneli [99]
145
VRE
2–6
74
?
?
30
?
Totals
804
411
156
The presence of subretinal or vitreous seeds is a common cause of treatment failure in eyes undergoing intravenous chemotherapy. Systemic chemotherapy definitely causes regression of some tumor seeds in the vitreous cavity and subretinal space, although the response is variable, unpredictable, and typically not complete [18, 27, 32]. In 1996, Shields reported that calcification occurred in 50 % of vitreous seeds and 78 % of subretinal seeds following 2 cycles of chemotherapy, similar to the findings after external beam radiation [32]. Overall, eyes with vitreous and subretinal seeds developed tumor recurrence at a rate of approximately 46 and 62 % at 3 years and 5 years of follow-up, respectively [29]. If some vitreous or subretinal seeds are viable after 6 cycles of chemotherapy, they will inevitably cause new retinal tumor recurrences. Wilson has also pointed out that seed dispersion can be induced or worsened by chemotherapy: as tumors regress during the initial cycles of intravenous chemotherapy, tumors can fragment and release seeds into the vitreous cavity [20]. Persistence of seeds may also represent inadequate penetration of the chemotherapy to the avascular sites in the vitreous cavity and subretinal space. Another possible cause of treatment failure with intravenous chemotherapy is the development of new tumors. In Lee’s study of single-agent carboplatin, 47 % of eyes developed additional tumors during the period of follow-up, and 37 % of eyes had new tumors only 1 month after the initial cycle of chemotherapy [22]. The risk of new tumor formation was more than twice as likely if the child was treated before the age of 6 months of age, and nearly all new tumors occurred in the first 2 years of life. These findings confirmed that systemic chemotherapy does not appear to have a protective or prophylactic effect against the development of new tumors, even in the immediate posttreatment period. Therefore, patients undergoing intravenous chemotherapy need to be monitored for the development of new tumor foci in both eyes before, during, and after the completion of intravenous chemotherapy.
9.3.4 Complications
Short-term systemic side effects of chemotherapy are common, including fatigue, nausea and vomiting, and hematologic problems such as leukopenia, thrombocytopenia, and anemia. Occasionally, patients require admission for transfusions or work-ups for neutropenic fevers, but hematologic suppression rarely requires a delay of chemotherapy doses [9]. Ototoxicity has been reported in 0–17 % of patients with therapeutic doses of carboplatin, with the risk being the highest in children below 6 months of age [31, 33]. Baseline hearing testing is encouraged in all patients [31]. Several ophthalmic complications have been reported in patients undergoing intravenous chemotherapy and focal therapy for retinoblastoma, although in general these cases are rare. Anagoste reported three cases of rhegmatogenous retinal detachments and Gombos reported a case of intraocular cholesterosis following intravenous chemotherapy [34, 35]. Parents should be warned, however, that iatrogenic iris injury is a remote possibility in eyes that undergo repeated laser treatments.
A rare but potentially life-threatening complication of intravenous chemotherapy is the development of secondary nonocular cancers, particularly hematologic malignancies such as leukemia. Acute myelogenous leukemia (AML) has been reported following the use of etoposide with relatively short latency periods of 1–7 years [36]. In lymphoblastic leukemia patients undergoing chemotherapy, the long-term risk of AML has been reported to be 2–3 % for intensive weekly or twice weekly schedules of teniposide or etoposide [36]. A recent survey by Gombos et al. identified 12 cases of AML in retinoblastoma patients undergoing intravenous chemotherapy [37]. This survey included a questionnaire of retinoblastoma specialists practicing throughout the Americas and Europe, as well as a database of 1,601 patients from the National Institutes of Health, Department of Health and Human Services, and the Ophthalmic Oncology Service at the Memorial Sloan-Kettering Cancer Center. Among the 12 identified cases, 9 patients had bilateral or multifocal retinoblastoma, and 8 patients had received an epipodophyllotoxin (etoposide or teniposide). Although a causative link between AML and epipodophyllotoxin therapy in retinoblastoma patients has not been established, it is concerning that prior to the intravenous chemotherapy era, the development of leukemia was thought to be a rare event. In a study published in 1984, Abramson et al. observed only one case of leukemia among 1,900 survivors of retinoblastoma [30]. Without knowing the total number of retinoblastoma patients treated with intravenous chemotherapy in the modern era, it is not possible to calculate the risk of developing AML in this population of patients or even to conclude that a definite association exists. However, this recent report by Gombos et al. suggests that further investigation will be required to fully assess the validity of this risk.
9.4 Periocular Chemotherapy (Injections and Exoplants)
There is a great need to consistently achieve therapeutic levels of therapeutic agents on a regimen that is not limited by systemic toxicity. The chemotherapeutic agents currently used (carboplatin, etoposide, and vincristine) are small molecules and should enter the eye easily in an appropriate trans-scleral delivery system. Carvalho and colleagues have described a promising closed trans-scleral delivery system that consists of a small, impermeable refillable silicone reservoir that can be firmly attached to the episclera with minimally invasive conjunctival surgery (Fig. 9.1) [38]. Once in place the reservoir can be filled and refilled as often as necessary by simple transconjunctival injections. These authors have demonstrate the superiority of this trans-scleral protected delivery system in delivering agents to the posterior vitreous and retina when directly compared to agent delivery via subtenon injection. In addition, much less delivered agent gains access to the plasma. As many as four of the reservoirs can be attached to the episclera of a single eye, allowing the concurrent delivery of multimodality therapy. The simplicity of the placement and recharging of the reservoir, the sustained delivery of high levels of agent to the vitreous and the posterior retina, and the potential for an inexpensive route for delivering tumor-targeted biotherapies make this type of trans-scleral delivery very promising. The current development of exoplants is still in preclinical stages.
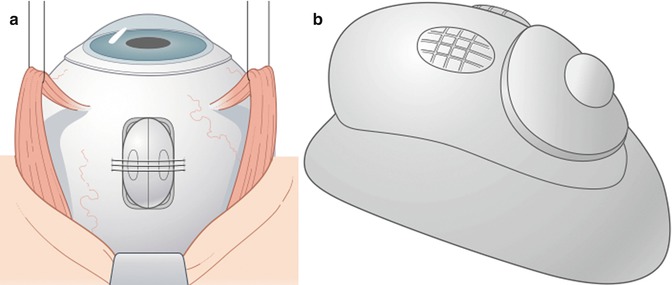
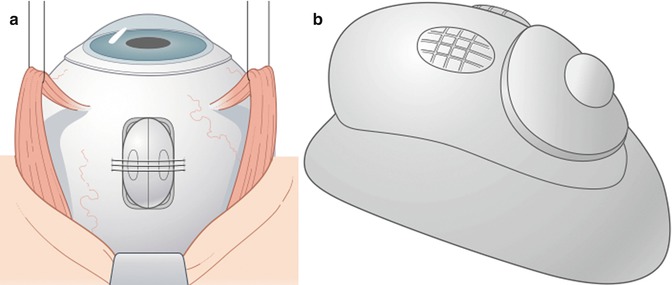
Fig. 9.1
Schematic of the eye (not to scale) that shows the positioning of the episcleral reservoir (a). This image shows a rigid reservoir held in place by scleral sutures. The most current version of the reservoir is made of flexible silicone. Indenting the reservoir creates a suction that securely attaches the implant to the sclera. Tissue adhesive can also be used to assist in maintaining its position. A higher magnification of the implant (b). The round soft refill port can be palpated through the overlying conjunctiva for refilling of the reservoir with a small 30-gauge needle
In 1998, Mendelsohn and Abramson showed that peribulbar and episcleral injection of carboplatin could achieve higher vitreous concentrations than intravenous administration in primates [39].
9.4.1 Efficacy
Murray and colleagues showed a dose-dependent inhibition of tumor growth with subconjunctivally delivered carboplatin in transgenic retinoblastoma mice [40]. The first clinical trial was performed by Abramson on 11 children with bilateral retinoblastoma, using a median of three injections per eye with an interval of 21 days between injections [41, 42]. In that trial, a major clinical response was observed in three of five eyes with vitreous seeds and two of five eyes with retinal tumors. Periorbital edema and redness after injection were observed in 4 of 13 eyes and one patient developed optic neuropathy (Fig. 9.2).
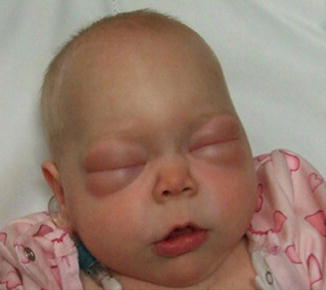
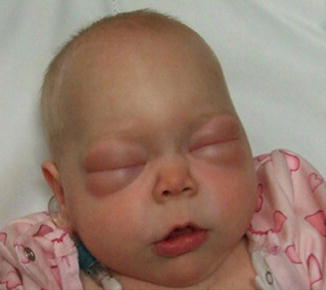
Fig. 9.2
Periocular edema 2 days after bilateral periocular (subtenon injection of 10 mg/ml carboplatin x 2 sites). The edema resolved within a week with a short course of oral steroids without residual deficit
9.4.2 Complications
Although the early clinical experience with periocular injection has been encouraging, there have also been reports of local complications with this delivery method. Schmack and colleagues reported four cases of optic nerve atrophy in eyes that had been enucleated following periocular carboplatin injection [43]. The enucleated eyes had received between 3 and 7 periocular carboplatin injections. Histopathologic examination showed focal areas of ischemic necrosis and atrophy in the retrobulbar optic nerve along with dystrophic calcification and inflammation in the surrounding fibrovascular tissue. Mulvihill and colleagues reported ten patients with ocular motility restriction following subtenon carboplatin injection, diagnosed by forced duction testing [44]. They reported that subtenon carboplatin injection was associated with significant fibrosis of orbital soft tissues, restricting eye movement and making subsequent enucleation difficult. Because of the potential for local scarring and toxicity, most centers no longer routinely perform periocular carboplatin injections.
9.5 Selective Intra-arterial Chemotherapy (IAC)
As early as 1953, Kupfer described a case of retinoblastoma treated with nitrogen mustard injected directly into the periocular circulation [48]. Later, in the 1960s and 1970s, Reese and Ellsworth combined external beam radiotherapy with intracarotid chemotherapeutic agents [49]. In the 1980s, Kaneko at the National Cancer Institute in Tokyo, Japan, began working on a new method to administer ocular chemotherapy – he described it as selective ophthalmic arterial infusion (SOAI) [50]. With this approach, developed primarily to avoid enucleation, a balloon catheter was inserted in the femoral artery, past the internal carotid, and guided just past the origin of the ophthalmic artery. The balloon was then inflated and melphalan injected into the arterial vasculature. Often adjuvant treatments were also administered but more than half of the treated eyes were preserved. In 2008, Abramson and colleagues modified this technique with direct insertion of the cannula just past the ostium of the artery (Chap. 12) [42].
Over the past 5 years, selective intra-arterial infusion of chemotherapy (IAC) has emerged as an important new modality for treating eyes with advanced intraocular retinoblastoma [51–56]. IAC is currently used as salvage therapy in most modern retinoblastoma centers and used as primary therapy at several centers including MSKCC [53]. Doses used for IAC have ranged between 3.0 and 7.5 mg of melphalan per treatment in primary cases and a multidrug regimen of carboplatin, melphalan, and topotecan in salvage cases.
9.5.1 Efficacy
The initial phase I/II trial of 10 patients with group V retinoblastoma salvaged seven eyes that would have otherwise been enucleated [42]. While the initial series used melphalan, additional follow-up reports have infused other agents including carboplatin and topotecan (alone or in combination) with good results. The technique has been used successfully in unilateral and bilateral cases and as both a primary and salvage approach. Follow-up electroretinogram (ERG) data suggests improved ERG findings in some very advanced cases with the resolution of the retinal detachment [57]. Defining an event as “enucleation or need for radiotherapy,” 4-year data from the Abramson group demonstrated a 81.7 % event-free survival for eyes that received intra-arterial chemotherapy as primary treatment and 58.4 % for eyes that had previous treatment failure with intravenous chemotherapy and/or external beam radiation therapy [53].
9.5.2 Complications
Despite the encouraging initial data for IAC, there is also growing evidence for potential ocular toxicity with this therapy, ranging from minor side effects (periocular edema, transient lash loss, forehead hyperemia) to more serious complications such as retinal artery occlusion and vitreous hemorrhage [58–60]. Fortunately, neurologic complications related to the catheterization process appear to be extremely rare with this technique. However, systemic neutropenia has been reported in a minority of children with IAC [53]. Concern has also been raised regarding the clinical significance of low-dose radiation exposure from the fluoroscopy used during the IAC procedure [61–63]. Finally, IAC is not widely available even in developed countries and clinical success rates appear to vary between centers, perhaps related to the technical proficiency of the interventional neuroradiologist performing the procedure. When IAC is used as primary therapy, the benefit of avoiding systemic chemotherapy in children with retinoblastoma must be weighed against the higher risk of local complications and the complexity of the catheterization procedure. When considering its use as salvage therapy, the different set of potential side effects between IAC and EBR must be carefully weighed in an individual case, taking into account the patient age and whether the recurrent disease is unilateral or bilateral.
9.6 Intravitreal Chemotherapy
Intravitreal chemotherapy (IVC) injection has recently emerged as a potential new modality to salvage eyes with residual vitreous seeding after systemic or intra-arterial chemotherapy (Chap. 12). For many years, the field of retinoblastoma management has avoided intraocular injection due to widespread concerns that a needle entering an eye with active retinoblastoma would lead to the extraocular spread of cancer cells. There is histopathologic evidence of tumor cells in needle tracks of eyes with active retinoblastoma, although documented cases of clinical extraocular relapse after fine needle aspiration biopsy are rare [64, 65]. There are, however, documented cases of extraocular spread after the performance of vitrectomy (with positive-pressure infusion) in eyes with unsuspected retinoblastoma [66].
9.6.1 Efficacy
Seregard initially reported on a series of three children with recurrent retinoblastoma being treated with IVC in 1995 [67]. Since then, there have been other reports of IVC being used successfully for children with active vitreous seeding. Suzuki and Kaneko reported on 237 eyes of 227 patients treated with 896 IVC injections of melphalan, with only a 0.4 % rate of extraocular spread with a mean follow-up of 91 months [50, 68]. Munier used 135 IVC injections of melphalan in 30 eyes of 30 children who had failed systemic chemotherapy. In his series, IVC injections were given with a 32-gauge needle using several important safety measures including: (1) ultrasound biomicroscopy (UBM) to rule out pars plana involvement at the site of injection, (2) pre-injection paracentesis, and (3) postinjection cryotherapy at the site of injection [69]. Importantly, no child in the Munier series had evidence of extraocular spread during the period of follow-up and approximately 80 % of eyes with vitreous seeding were salvaged [69, 70]. Even with these precautions, repeated injections into the vitreous cavity does carry the potential for tumor spread and it is important to use this technique selectively until there are data on its long-term safety and efficacy.
The candidates for IVC melphalan injections are those patients with isolated vitreous seeding and minimal tumor load after chemotherapy and/or radiation. Our initial experience in Los Angeles with intravitreal injection of melphalan has been very encouraging with a high salvage rate for eyes with vitreous seeding, with minimal side effects. Intravitreal melphalan injections do not appear to be effective for retina-based tumors. Based on the evidence to date, external beam radiotherapy is probably a more effective modality for treating vitreous seeding than IVC, but radiotherapy is associated with serious side effects, especially in children younger than 12–18 months of age (see section on EBR).
9.6.2 Complications
To avoid complications with this technique, it is important to carefully follow the protocol published by Munier [69]. By using small 32- or 33-gauge needles, performing a paracentesis, and applying cryotherapy at the site of injection, the risk of extraocular spread should be remote. In our experience, peripheral chorioretinal atrophy commonly occurs at the site of injection but no serious vision-limiting side effects have been encountered with doses up to 40 mcg. Rare cases of ocular phthisis have been reported with IVC, and the risk seems to be correlated with higher doses [71]. Although more investigation is needed, intravitreal chemotherapy appears to be a viable option for salvaging selected eyes with isolated vitreous seeding, as long as certain precautions are followed.
9.7 Laser Therapy
Laser therapy is used for the following indications in the management of intraocular retinoblastoma: (1) for accomplishing consolidation of large tumors after systemic chemotherapy (i.e., chemoreduction), (2) for treating small peripheral or posterior tumors as the sole modality, and (3) for eradicating small tumor recurrences within or adjacent to scars following chemotherapy and/or radiotherapy (Chap. 10). When used in conjunction with primary chemotherapy for intraocular retinoblastoma, focal consolidation can be accomplished with either the green 532 nm argon or the 810 nm diode infrared laser. The shorter wavelength green 532 nm argon laser is more readily absorbed in the relatively nonpigmented retinoblastoma tumor, while the longer wave length 810 nm diode infrared laser achieves deeper penetration in the presence of intact retinal pigment epithelium. The technique we find useful with the argon 532 nm is essentially the same for both primary treatment of group A lesions and consolidation following primary chemotherapy in groups B–D. In general, focal consolidation begins after the first or second cycle of systemic chemotherapy after the tumor volume has been reduced.
9.7.1 Treatment Parameters
The goal of the therapy is to completely cover each lesion with 30 % overlap during at least three different sessions. We choose initial power settings of 250–300 mW, with durations of 300–500 ms. The power and time settings are kept low to prevent tumor disruption and hemorrhage that may be associated with excessive energy delivery. The first burns are placed at the edge of the lesion with the spot half on and half off the tumor. The power and/or duration can be adjusted to achieve gentle whitening of the tumor. We do not recommend exceeding 500–600 mW and 700 ms with the 532 mm laser. Once the lesion is outlined, the entire lesion including any type I regression-associated calcium is covered with overlapping rows of burns. Larger lesions undergoing chemoreduction may require 200–400 burns for good coverage. The burns over the thicker areas of the tumor may be virtually invisible compared with those placed at the edge of the lesion. The power or duration should not be increased to compensate for the decreased absorption over the thicker parts of the lesion. Repeat the laser coverage at 2–4 week intervals during and/or after the administration of systemic chemotherapy until the entire lesion has been covered on at least three different occasions.
Because the infrared 810 nm diode laser has a longer wavelength than the argon laser, it penetrates deeper and is absorbed mainly by the retinal pigment epithelium. Therefore, it is particularly useful if retinal pigmented epithelium (RPE) is intact under the lesion to be treated. Another advantage of the diode laser is that its larger spot size allows more rapid coverage of the lesion and a lower risk of delivering excessive energy that might cause bleeding or tumor disruption. The diode laser can also be used for photocoagulation or transpupillary thermotherapy, depending on the settings utilized (Chap. 10). The endpoint of energy application is, like that for the argon laser, a gentle whitening of a spot placed half on and half off the tumor. Because of the larger spot size, the power is generally set initially at between 300 and 500 mW for 500 ms. The power can be adjusted upward to 700–800 mW if required to achieve the desired endpoint. If the active tumor focus demonstrates growth after first session of laser treatment, a second application should be attempted at a high-power level. Persistent growth after the second laser session is an indication that another modality will be needed to eradicate the tumor.
9.7.2 Efficacy
Laser photocoagulation is an appropriate method of management in cases where the tumor is located posteriorly, the media are clear, and the tumor is 3.0 mm or less in diameter and 2.0 mm or less in thickness without seeding into the adjacent vitreous.
In a series of 188 tumors that had mean tumor diameter of 3.0 mm and thickness of 2.0 mm, tumor regression was achieved in 86 % with a recurrence rate of 14 % [72]. Using the diode laser on a continuous mode (i.e., thermotherapy), Abramson was able to achieve complete regression in 84 out of 91 tumors (92 %) [73]. Larger tumors are at greater risk for complications such as focal iris atrophy and focal paraxial lens opacity because they require more intense therapy as compared to smaller tumors.
9.7.3 Complications
Complications of focal laser consolidation include burns of the iris at the pupillary margin and focal lens opacities, both of which are rare in experienced hands. Other complications that are associated with excessive energy delivered to the tumor include subhyaloid and vitreous hemorrhage. Theoretically, it is possible to mechanically disrupt the tumor and create vitreous seeding of the tumor by using excessive energy (power Å ~ time) levels but that complication has also been a very rare event in patient care if the above cautions are exercised. In approximately 1,000 lesions in more than 300 eyes treated in Los Angeles, we have seen tumor disruption by the laser on only one tumor. In that case, early in the series, treatment was done before chemotherapy was given and the laser power was increased to approximately 900 mW. Vitreous hemorrhage and tumor seeding ultimately resulted in loss of the eye. The most significant long-term complication of focal consolidation is decreased vision from RPE scar migration or “creep” in lesions near the foveola. A judicious approach is required when applying laser on the foveal side of a tumor near fixation to minimize the resulting scotoma. Lee and colleagues demonstrated an increase in the size of laser scars following red diode laser application [74]. It is reasonable to consider close observation after sufficient primary chemotherapy of a small tumor located near the fovea until documented growth is seen. In some instances, central vision can be spared if regrowth does not occur. Tumors that exist in the maculopapillary bundle can be managed in this fashion, especially if the contralateral eye has been enucleated or has poor visual prognosis.
9.8 Cryotherapy
Destruction of retinoblastoma tumors by cryotherapy results from disruption of cellular membranes following the freeze–thaw cycle. It can also have a local vasoocclusive effect on the tumor and nearby retina/choroid. Cryotherapy is useful for small peripheral tumors and can be used successfully for lesions up to 3.0 mm in diameter and 2.0 mm in thickness (Chap. 10). Cryotherapy can also be used to eradicate small tumors with localized vitreous seeding near the apex, assuming that the ice ball from the treatment can encompass both the tumor and the seeds. The difficulty in using cryotherapy as local treatment for posterior pole tumors is that a surgical procedure is required to open the conjunctiva so that accurate placement of the probe can be achieved. In addition, because the probe tip cannot be visualized while the freezing is taking place, it is theoretically possible to freeze the macula or optic nerve. An important consideration to keep in mind is that cryotherapy routinely destroys a great deal of normal retina surrounding the lesion, thereby increasing the visual deficit from the resulting chorioretinal scar. Therefore, the location and size of the tumor are important considerations when using cryotherapy for retinoblastoma.
9.8.1 Treatment Parameters
The treatment begins by confirming that the cryotherapy probe and foot pedal are functioning properly, Using indirect ophthalmoscopy, the probe of the cryotherapy unit is used to localize and elevate the tumor on the tip of the probe. Once the probe is directly beneath the tumor, freezing is initiated and the ice ball maintained until it encompasses the entire tumor mass. After the treatment covers the apex of the tumor for 2 mm, the ice ball is allowed to thaw, and this freeze–thaw cycle repeated for a total of two or three applications. To avoid iatrogenic injury to the globe, it is important not to move the probe on the sclera until the ice ball has completely resolved.
9.8.2 Efficacy
Cryotherapy is indicated for anteriorly located tumors with clear media, and the highest success rate is achieved for primary, small tumors without seeding. Proper patient selection and utilization of careful technique are important factors in achieving a high success rate. In a series of 138 tumors treated with cryotherapy by Abramson, 70 % of tumors overall were cured with cryotherapy [75]. For primary tumors without previous therapy, the cure rate was 95 %, but all of the tumors at the vitreous base with seeding failed [75].
9.8.3 Complications
Complications of cryotherapy include vitreous hemorrhage, development of subretinal fluid, and retinal holes. Very rarely, retinal breaks from cryotherapy can result from a combination of the atrophic retina and vitreous traction, particularly at the edges of the treated area. We have observed several cases of rhegmatogenous retinal detachment when large superior, partially calcified tumors were treated extensively with cryotherapy. Extensive cryotherapy can also cause atrophy of the sclera, with formation of a pseudocoloboma of the sclera. The presence of preexisting subretinal fluid in the region of proposed cryotherapy is a relative contraindication. The use of proper technique is critical in avoiding these complications, and it is particularly important to not move the cryotherapy probe until there is visual confirmation through the indirect ophthalmoscope that the ice ball has completely dissipated.
< div class='tao-gold-member'>
Only gold members can continue reading. Log In or Register a > to continue
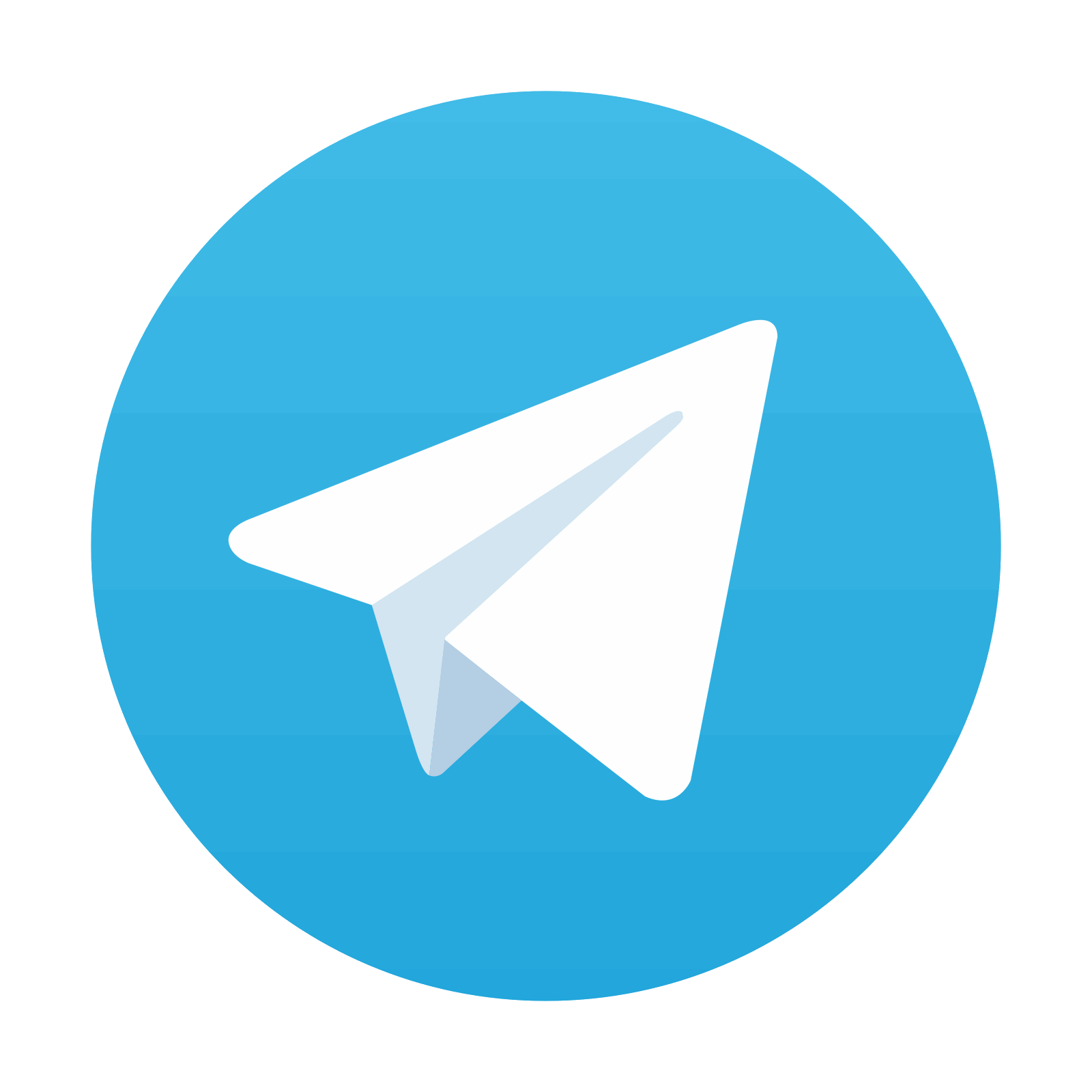
Stay updated, free articles. Join our Telegram channel

Full access? Get Clinical Tree
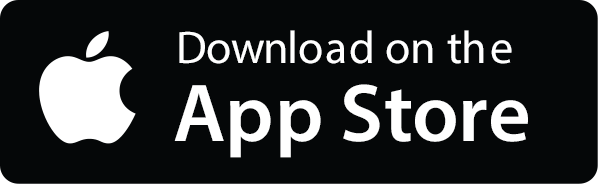
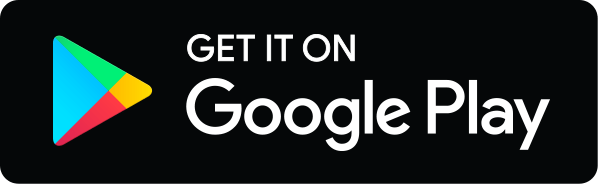