Chapter 51 Retinal Artery Obstructions
CRAO was first described in 1859 in von Graefe’s report of multiple systemic emboli in the setting of endocarditis causing obstruction of the central retinal artery.1 Since then significant literature has been published on retinal arterial occlusions and their classification, epidemiology, presentation, prognosis, and treatment. Here we use an arbitrary classification, as indicated below, to discuss these disorders in sequence:
• Central retinal artery obstruction (CRAO)
• Branch retinal artery obstruction (BRAO)
• Cilioretinal artery obstruction (CLRAO)
• Combined retinal artery and vein obstruction
Central retinal artery obstruction
Epidemiology
The true incidence of CRAOs is unknown. The estimated incidence of CRAO is reported to be roughly 1 in 10 000 cases at tertiary referral centers.2,3 The incidence may be even lower for the general population, at approximately 8.5 cases per 100 000.3 Similar to other vascular disorders, this condition is largely seen in older adults but cases in children and young adults have also been reported.4,5 The average age at presentation is in the early sixties, with greater than 90% of patients presenting at over 40 years of age. Men are affected more frequently than women. No predilection for one eye over the other has been reported; however, 1–2% of cases may manifest bilateral involvement.6
Clinical features
Typically, patients with acute CRAO present with monocular, painless, severe loss of vision which occurs acutely, possibly over the span of a few seconds. In some cases, premonitory episodes of amaurosis fugax may be reported. Amaurosis fugax represents transient acute retinal ischemia and typically suggests an embolic source of occlusion; however, it has also been reported in association with giant cell arteritis.7 Presence of amaurosis fugax also has a higher correlation with stroke compared with retinal emboli alone.8 The risk of CRAO after amaurosis fugax is estimated to be only 1% per year.9 Although CRAO rarely presents simultaneously in both eyes, it may occur sequentially.10
Visual acuity at the time of initial presentation ranges from counting fingers to light perception in 74–90% of eyes.6,11 Central visual acuity may be near normal in patients who have a transient CRAO or a cilioretinal artery providing sufficient vascular supply to the fovea. Connolly et al. reported a trend toward better visual acuities in patients with monocular CRAO secondary to giant cell arteritis as compared with those with CRAO due to other, largely embolic causes.12 The presence of an embolus is usually associated with poorer vision. The absence of light perception is rare; therefore, in such cases, concomitant choroidal circulation deficit (e.g., due to ophthalmic artery occlusion) or optic nerve involvement should be considered.6 Visual acuity tends only to improve within the first week of onset with minimal chance for appreciable improvement subsequently.11 Visual recovery after treatment has been shown to correlate with presenting visual acuity and the duration of visual impairment.13 Although visual acuity may spontaneously improve in up to 22% of patients with nonarteritic CRAO,11 less than 10% of patients report a meaningful recovery of vision.14
Typically an afferent pupillary defect develops within seconds following obstruction of the central retinal artery regardless of macular sparing.4 Intraocular pressure is often normal at presentation but may become elevated in the setting of rubeosis iridis.
In a majority of cases of acute CRAO, the anterior-segment exam is normal initially. If rubeosis iridis is present acutely, ocular ischemia secondary to the presence of a concomitant carotid artery obstruction should be considered. The incidence of rubeosis in CRAO is 16.6–18.8%.15–17 As compared to central retinal vein occlusions (CRVO), iris rubeosis tends to occur earlier after CRAO – at a mean of 4–5 weeks after onset compared to 5 months after onset in CRVO. Not surprisingly, rubeosis is more common in more severe and complete obstructions with extensive nonperfusion. The risk of developing iris neovascularization is greater for obstruction lasting greater than 1 week versus those lasting only a few days after onset.15–17 In the case of carotid obstruction, rubeosis iridis can cause elevated intraocular pressure to the point of exceeding the perfusion pressure in the central retinal artery and lead to an obstruction via this mechanism. Laser panretinal photocoagulation causes successful regression in approximately 65% of cases.18
In his 1891 report, Nettleship described in detail the ophthalmoscopic appearance of CRAO. “The classic dense, white haze of the central region in the retina with a well-marked clear patch at the yellow-spot was very well shown (Fig. 51.1); there were no hemorrhages; the arteries and veins were of about normal size, but no pulsation could be produced in any of them by pressure with the finger upon the globe.”19 Hayreh and Zimmerman investigated fundus changes in CRAOs in a large retrospective review in 2007 of 248 eyes of 240 patients. In the acute phase, his group noted a cherry-red spot (90%), posterior pole retinal opacity or whitening (58%), box-carring of retinal arteries and veins (19% and 20% respectively), retinal arterial attenuation (32%), optic disc edema (22%), and optic nerve pallor (39%). The retinal findings were predominantly located in the posterior pole with a normal-appearing periphery.20
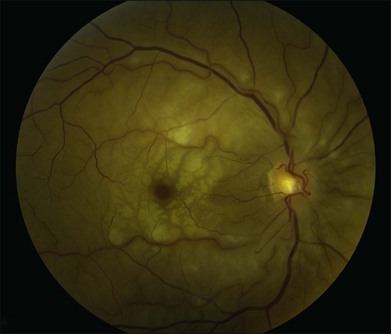
Fig. 51.1 Acute central retinal artery obstruction. Superficial retinal whitening or opacification is noted in the posterior pole with evidence of a central cherry-red spot. The opacification is most prominent in the peripheral fovea.
(Courtesy of Dr Steven Yeh, Emory Eye Center, Atlanta.)
Typically, retinal whitening in the posterior pole and a cherry-red spot are the earliest characteristic changes in CRAO. Both of these findings are clinical signs for which ophthalmologists have a high degree of clinical agreement.21 The retinal whitening corresponds to ischemic damage to the inner half of the retina and is due to opacification of the retinal nerve fiber and ganglion cell layer as a result of cessation of axoplasmic transport caused by the acute ischemic insult. The opacification is visible ophthalmoscopically where the ganglion cell layer is more than one cell thick, i.e., the macula, except in the foveal region, where a cherry-red spot is seen. The outer nuclear and plexiform layers and photoreceptors remain intact, as demonstrated in histologic studies.22,23 The size of the cherry-red spot is variable and is dependent on the width of the foveola. The cherry-red spot is actually normal-appearing retina and is observed in high contrast against the surrounding opacified retina because the thin retina in this location is nourished by the underlying choroidal circulation and as a result does not become hypoxic or opacified, permitting continued visualization of the underlying retinal pigment epithelium (RPE) and choroid.24 Similarly, the retinal periphery in CRAO cases appears normal because the retina is also thinner with a single layer of ganglion cells, such that the nutrition of the inner retinal layers can be maintained by the choroidal circulation alone. In experimental primate models of transiently induced CRAOs, retinal opacification was observed as early as 7 minutes after complete occlusion. The probability of a cherry-red spot still being present decreases with increasing duration from the onset of CRAO: 88% after 1 week, 59% after 2 weeks, 47% after 3 weeks, and 19% after 4 weeks after onset. Typically, the retinal opacification resolves over a period of 4–6 weeks, although at least some retinal whitening is noted in 17% of patients with complete, nontransient CRAO after 1 month.20 Pathologically, this evolution corresponds to a resolution of initial acute ischemia-induced intracellular edema with subsequent loss of neuronal cells and the development of an acellular scar of the inner retinal cell layers.25
A patent cilioretinal artery supplying some or all of the papillomacular bundle is seen in approximately one-third of cases. In cases of cilioretinal sparing, retinal whitening will be clearly demarcated around the area of preserved macula perfused by the cilioretinal circulation (Fig. 51.2A). In these cases, the visual acuity will be dictated by the location and extent of the area of the papillomacular bundle perfused by the patent cilioretinal artery.11,26–28 Sparing of the fovea may be associated with excellent visual acuity, albeit with a significant visual field deficit corresponding to the topography of the occlusion.
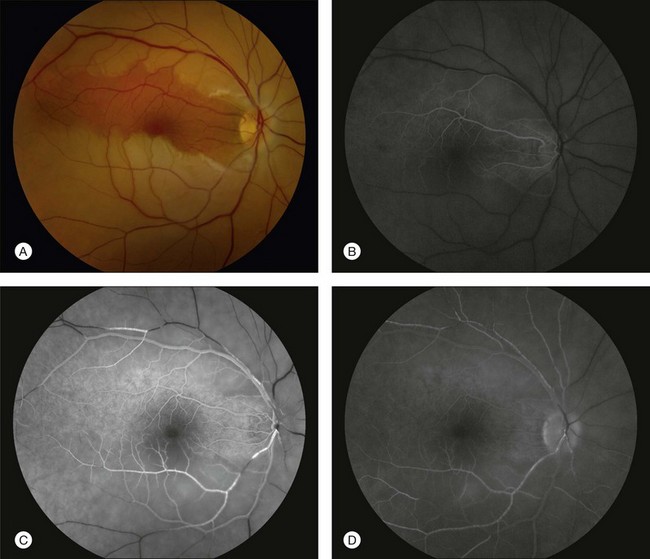
Fig. 51.2 Acute central retinal artery occlusion with cilioretinal artery sparing. (A) Retinal opacification is clearly demarcated around the preserved macula perfused by the patent cilioretinal artery. The perfused area includes the fovea; therefore the patient’s presenting visual acuity was good. (B) At 19 seconds, the cilioretinal artery is perfused with retrograde flow into the retinal veins. (C) At 47 seconds, incomplete appearance of fluorescein dye is noted in the retinal arteries. (D) At 9 minutes the arterial circulation is still not completely filled, demonstrating a severely delayed arteriovenous transit time.
(Courtesy of Dr John Payne, Emory Eye Center, Atlanta.)
The appearance of the retinal vasculature can be quite variable soon after the onset of CRAO, and the presence of a normal-appearing vasculature should not exclude the diagnosis. In Nettleship’s 19th-century description of CRAO, he noted the appearance of the retinal vasculature to have a stagnated arterial blood column without attenuation.19 In the natural history study by Hayreh and Zimmerman,20 only 15% of acute CRAO had normal-appearing retinal arteries. Box-carring or segmentation of the blood column of both the arteries and veins can occur secondary to separation of blood serum from erythrocytes in a stacked or rouleaux formation.
Retinal emboli are visible in 20–40% of eyes with CRAO.4,21 Retinal emboli are the most common cause of nonarteritic CRAO and BRAO.29 The most common variant is a yellow, refractile cholesterol embolus (Hollenhorst plaque) (Fig. 51.3). According to a study by Arruga and Sanders, retinal emboli consist of cholesterol in 74% of cases, calcified material in 15.5%, and platelet and fibrin in 15.5%.30 These cholesterol emboli typically originate from the carotid arteries in the setting of atherosclerotic disease, but can also arise from the aortic arch, ophthalmic artery, or proximal central retinal artery. Cholesterol emboli are often small, do not completely obstruct retinal arterial blood flow, and are frequently found at bifurcation sites (Fig. 51.3). Emboli can often be asymptomatic and migration with disappearance of retinal emboli is common.20 Calcific emboli are less common than cholesterol emboli but are typically larger and cause more severe or complete obstruction. They most commonly originate from the cardiac valves.24,31
The optic nerve is acutely edematous in nearly all cases of arteritic CRAO as a result of the associated anterior ischemic optic neuropathy that is typically observed in these patients. In the acute phase of nonarteritic CRAO, the disc may be normal, hyperemic, edematous, and, rarely, pale. Acute-phase optic nerve pallor is due to ischemic opacification of the surface nerve fiber layer since this layer of the optic nerve is supplied by retinal circulation.32 Neovascularization of the disc in acute CRAO is rare but has been reported, typically in association with a chronically hypoxic retina (e.g., concomitant diabetic retinopathy, ischemic CRVO, or ocular ischemia).19,20
The most frequent findings in the chronic stage of eyes with CRAO are optic atrophy (91%), retinal arterial attenuation (58%), cilioretinal collaterals (18%), macular RPE changes (11%), and cotton-wool spots (3%) (Fig. 51.4). In experimental studies in rhesus monkeys, the extent of optic nerve and nerve fiber layer damage has been correlated with the duration of CRAO.33 Chronic-phase optic nerve pallor is due to optic atrophy and nerve fiber loss (Fig. 51.5). In arteritic CRAO, the associated anterior ischemic optic neuropathy also contributes to the development of pallor.20 In chronic CRAO, neovascularization of the optic disc rarely occurs, presumably because nonviable tissue is less likely to elaborate angiogenic factors compared with chronically ischemic but viable retinal tissue seen in cases of diabetic retinopathy or retinal vein occlusion.34 Incidence of neovascularization of the disc in one retrospective study was only 1.8%.35 Concomitant rubeosis iridis may also occur in the setting of chronic CRAO. Retinal arterial attenuation is more common in the chronic phase of disease than in the acute phase.20 Months after an acute CRAO, cilioretinal collaterals may develop as a result of a compensatory enlargement of capillary anastomoses between retinal capillaries on the surface of the disc and ciliary capillaries in deeper parts of the optic nerve head. The probability of developing cilioretinal disc collaterals was 4% at 1 month and 18% at 3 months from onset of CRAO in one study.20 Macular RPE changes are seen with CRAO but are much less common than in ophthalmic artery obstruction, where the choroidal circulation is also involved.
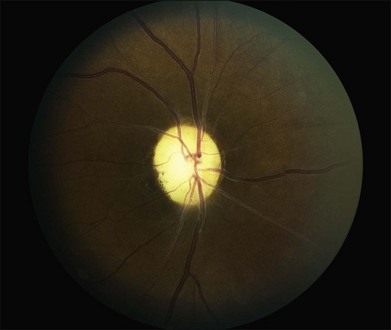
Fig. 51.4 Chronic central retinal artery occlusion. Optic nerve pallor from atrophy and severe arterial attenuation is seen in this patient with a remote central artery occlusion.
Ancillary studies
Fluorescein angiography in CRAO almost always initially shows some variable residual retinal circulation with delayed and sluggish filling of the retinal vasculature. Complete absence of retinal filling is rare. Appearance of dye in the central retinal artery is typically delayed by 5–20 seconds. However, the delay in retinal arterial branches is even more substantial. The fluorescein dye lines the arterial walls in a pattern similar to the laminar flow filling of normal retinal veins. In cases with visible intra-arterial emboli, the arteriovenous transit time can be even further delayed (Fig. 51.2B–D).36 The severity of obstruction and retinal ischemia correlates with less favorable initial visual acuities. Staining of the optic disc can be variable; however, staining of the retinal vessels is rare. Areas of delayed choroidal perfusion, characterized by a delay in filling of more than 10 seconds compared to adjacent normal choroid, may be seen in about 11% of eyes with acute CRAO.6 Leakage of fluorescein dye at the level of the RPE is generally not seen with CRAO unless the choroidal circulation is involved.37 Typically, the retinal circulation is re-established after an acute CRAO but the inner retinal tissue has generally already infarcted by then. Therefore, although the fluorescein angiogram may return to relatively normal appearance, the vision loss, optic nerve atrophy, and arterial narrowing persist.24 For patients with a normalized fluorescein angiogram who do not go on to develop optic atrophy, the diagnosis of a true CRAO should be called into question, though it is possible that some individuals may have reperfusion before the irreversible damage has occurred.
In the acute stage, optical coherence tomography (OCT) shows an irregular macular contour with increased reflectivity of the inner retina. This corresponds to intracellular edema and explains the lack of intraretinal, hyporeflective fluid spaces in cases of CRAO or BRAO. The reflectivity of the outer retinal layers and RPE is blocked by the highly reflective inner retinal layer. No retinal thickening secondary to the accumulation of serous fluid escaping from retinal capillaries into the extracellular space is seen (Fig. 51.6). OCT images of chronic CRAO show thinning and atrophy of the inner retina. OCT can be helpful in cases of chronic CRAO where the fundus may appear featureless but the OCT shows inner retinal atrophy with preservation of the outer retina.38–40
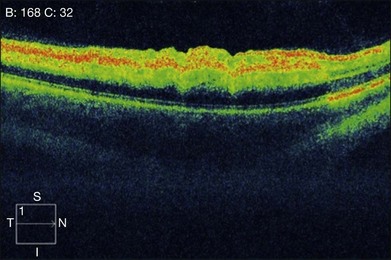
Fig. 51.6 Spectral-domain optical coherence tomography (SD-OCT) of acute central retinal artery occlusion (CRAO). An irregular foveal contour is seen with a highly reflective, thickened inner retina. The outer retina is relatively hyporeflective because of blocking from the thickened inner retina. The inner-segment–outer-segment junction and the external limiting membrane are intact, as would be expected with acute CRAO.
Central scotoma is the most common defect observed on macular visual field testing followed by paracentral scotoma. Patients with cilioretinal sparing show a preserved central island of vision corresponding to the area perfused by the patent cilioretinal artery. Peripheral constriction is the most common visual field deficit noted in these patients.11 A preserved temporal island may be seen in some patients, presumably secondary to choroid-derived perfusion of the nasal retina.26 Visual field defects improve in approximately 28% of patients, remain stable in 57%, and worsen in 7%.11
In a CRAO, electroretinography typically demonstrates more severe attenuation of the b-wave than the a-wave since the inner retinal layers are more affected – this produces a characteristic negative waveform with the scotopic white stimulus (Fig. 51.7). Diminution of the a-wave and b-wave may suggest outer retinal damage secondary to choroidal vascular hypoperfusion in the setting of an ophthalmic artery occlusion in addition to a CRAO.41
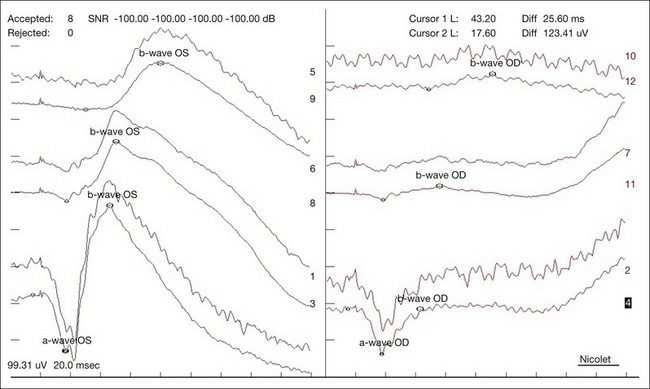
Fig. 51.7 Electroretinogram. The responses from a normal eye are seen on the left. The tracings on the right are from an eye with a central retinal artery occlusion (CRAO). The bottom right tracing shows the maximum response to the scotopic white stimulus. The a-wave is of normal amplitude; however, the b-wave does not reach the baseline, yielding a negative waveform, as is typically seen with a CRAO.
Autofluorescence imaging in the area supplied by the occluded retinal artery acutely shows decreased autofluorescence due to blockage of the normal autofluorescence of the RPE by the thickened inner retina. This blockage resolves over time and may evolve into a window defect with increased autofluorescence in the chronic phase as areas of significant inner retinal thinning develop.39
Systemic associations
The distribution of systemic associations for CRAO varies depending on age. Overall, embolism from carotid artery atherosclerosis is the most common etiology for retinal arterial occlusion; however, carotid disease is relatively rare in patients under the age of 40 in whom cardiac embolism is the most common etiology.4,5,42 The systemic and ocular abnormalities that have been associated with retinal arterial occlusions are summarized in Box 51.1.
Box 51.1
Systemic and ocular abnormalities associated with retinal arterial occlusion
Embolic sources
• Systemic arterial hypertension (via atherosclerotic plaque formation)6,24
• Carotid atherosclerosis8,99,100
• Cardiac valvular disease (rheumatic,49 mitral valve prolapse,49,50 aortic stenosis,49 mitral annular calcification56)
• Left ventricular hypertrophy56 and segmental wall motion abnormalities56
• Thrombus after myocardial infarction
• Carotid artery dissection102
• Intravenous drug use4,103,104
• Lipid emboli (pancreatitis)104
• Purtscher’s retinopathy (trauma)105
• Radiologic studies (carotid angiography,102 cerebral angiography,74 cardiac catheterization,60 lymphography,61 hysterosalpingography64)
• Deep-vein thrombosis (via paradoxical embolus through a cardiac wall defect)67
Ocular conditions
• Prepapillary arterial loops117
• Increased intraocular pressure (from intravitreal injection,91 gas expansion after vitrectomy,119 prone intraoperative positioning,120 retrobulbar hemorrhage,121 orbital emphysema122)

Full access? Get Clinical Tree
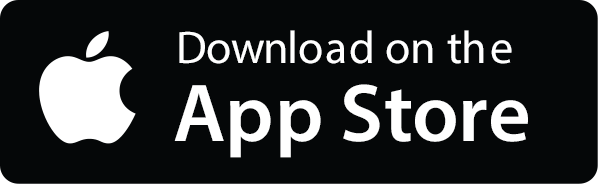
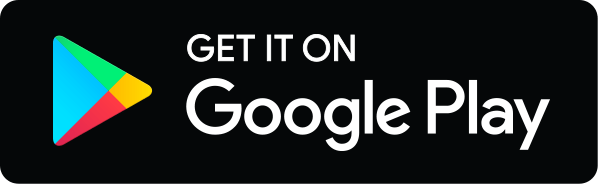