5 Pure Tone Audiometry We often conclude that someone has a hearing loss because we have to talk at a louder than normal level for that person to hear us. Even though we cannot directly experience the degree of that person’s hearing loss, we can appreciate its magnitude in terms of how loud we must speak to be heard. This is certainly not the only manifestation of a hearing impairment, but it does highlight an important point: We can quantify the degree of a patient’s hearing loss in terms of the magnitude of the stimulus needed for him to respond to it. The smallest intensity of a sound that a person needs to detect its presence is called his threshold for that sound. For clinical purposes, we define the threshold as the lowest intensity at which the patient responds to the sound at least 50% of the time. The sounds used to test a person’s hearing must be clearly specified so that his thresholds are both accurate and repeatable. The test sounds used to determine the degree of hearing loss are usually pure tones of different frequencies. Recall from Chapter 4 that the normal threshold value at each frequency is said to be 0 dB Hearing Level (HL). Also recall the actual physical magnitude (in decibels of sound pressure level, dB SPL) needed to produce 0 dB HL is specified in the American National Standard Specifications for Audiometers (ANSI S3.6-2010), which corresponds to several international (ISO) standards (see Chapter 4). Hearing thresholds are thus given in decibels of hearing level or dB HL, which is more completely expressed as “dB HL re: ANSI-2010,” “dB HL re: ANSI/ISO,” or “dB HL re: ANSI-1969” (because the original version was published in 1969). In the most general terms, a person has “normal hearing” if his thresholds are close to the norm and a “hearing loss” if the tones must be presented at higher intensities for them to be heard. In other words, the amount of the hearing loss is expressed in terms of how many decibels above 0 dB HL are needed to reach the person’s threshold. For example, when we say a patient has a hearing loss of 55 dB HL, we mean his ear problem has caused his threshold to be elevated to the extent that it is 55 dB higher than 0 dB HL. Test signals can be presented by air-conduction or bone-conduction, or in sound field. Thresholds are obtained by both air and bone-conduction because a comparison between the two sets of results enables us to distinguish between different kinds of hearing losses, as will be explained later. Sound field testing involves presenting signals from loudspeakers, and is discussed later in this chapter. Air-conduction testing usually involves presenting the test signals from standard audiometric (supra-aural) earphones, as shown in Fig. 5.1. Insert earphones can also be used for air-conduction testing. Recall from Chapter 4 that insert earphones go into the ear canals instead of being worn over the ears. They are useful when facing several problem situations, but the convenience of standard earphones makes them the method of choice under routine conditions. Earrings and most eyeglasses (except contact lenses) must be removed for both comfort and proper fitting of the earphones. It is also necessary to remove any other objects (e.g., headbands or other hair adornments) that could interfere with the placement of the headset (or the bone vibrator that will be used later). Hearing aids should be removed, turned off, and put away during the test. Chewing gum and candy must be disposed of. The audiologist should check to see whether putting pressure on the external ear seems to cause the ear canal to close. This is important because the pressure exerted by the earphones might similarly cause collapse of the ear canals, and give the false impression of a conductive hearing loss. We will return to the issue of ear canal collapse later in this chapter. Finally, the clinician gently places the headset on the patient, being careful that the earphone receivers are located over the entrances to the ear canals. It is not desirable to allow the patient to apply the headset because the fit may not be optimal. If the patient is allowed to do this, then the clinician must check the fit and make any necessary adjustments. Fig. 5.2 Arrangement of the bone-conduction vibrator at the mastoid process for bone-conduction testing. Bone-conduction is tested by applying a vibratory stimulus to the skull, which is transmitted to the cochlea and heard as sound. To do this, a bone-conduction vibrator is placed on the mastoid process or forehead, and is held in place by a spring headband. Even though mastoid versus forehead placement of the bone vibrator is an arguable issue (see below), mastoid placement is recommended here and is the more commonly used method. Fig. 5.2 illustrates the proper placement of a bone-conduction vibrator for the mastoid process. The bone-conduction vibrator itself is encased in a small plastic shell as shown in Fig. 4.3c of Chapter 4. The part that comes in contact with the skin is a disk with a flat surface having an area of 1.75 cm2. Most American audiometers use the Radioear B-71 bone-conduction vibrator, which is the one in the figure. Radioear’s model B-72 is also available but is less commonly used. The B-71 and B-72 vibrators have replaced the older B-70 model, which does not have a disk-shaped contact area. Before putting the bone vibrator on the patient, one must identify any structural aberrations or other problems that would affect the proper placement. Common problems are hair under the vibrator, oily skin, and oddly shaped or narrow mastoids that make it hard to place the vibrator without it slipping. Pathologies and surgically modified structures are less common but equally important. Earrings, glasses, hearing aids, etc., as well as gum and candy, should already have been removed prior to air-conduction testing. The vibrator is gently placed on the mastoid process on one side, and the other end of the spring band is placed gently on the opposite side of the head (usually just anterior to the opposite ear). The vibrator should be placed so that the surface of its disk sits flatly on the skin of the mastoid process without touching the pinna. The vibrator should be held to the head with a force corresponding to a least 400 g to achieve adequate reliability (Dirks 1964) and 5.4 newtons to meet ANSI S3.6-2010 standards. Placement variations on the mastoid can result in threshold differences. For this reason, many audiologists have the patient listen to a readily audible 500 Hz bone-conduction tone while they shift the vibrator around on the mastoid. The vibrator is then kept in the location where the tone sounds loudest. To minimize the chances that the vibrator will shift once it has been placed, it is wise to instruct the patient to keep her head still and not to talk during bone-conduction testing. The patient should also be instructed to tell you if the vibrator moves in any way. It is also a good idea to provide a strain relief so that an unintentional tug on the wire will not dislodge the vibrator. This can be done with a small clip or by looping the wire under the patient’s collar. We have been assuming that the ears are not covered by the earphones while bone-conduction is being tested. This is the typical method, and the results obtained this way are called unoccluded bone-conduction thresholds. In contrast, occluded bone-conduction thresholds are obtained when one or both ears are covered with the earphones. A stronger signal reaches the cochlea when bone-conduction signals are presented with the ears occluded compared with unoccluded. This boost is called the occlusion effect. As a result, occluded bone-conduction thresholds are lower (better) than unoccluded ones, and a given bone-conduction signal will sound louder with the ears covered compared with when the ears are open. An occlusion effect occurs when the cartilaginous section of the ear canal is occluded, but not when the bony portion is blocked. It is also absent when there is a disorder of the conductive system. The occlusion effect can be used clinically to help determine whether a conductive impairment is present in the form of the Bing test (described later in this chapter), as well as to help determine how much noise is needed for masking during bone-conduction testing (Chapter 9). The occlusion effect is discussed further in Chapters 2 and 9. The magnitude of the occlusion effect is found by simply comparing the bone-conduction thresholds obtained when the ears are occluded and unoccluded. Fig. 5.3 shows the mean occlusion effects obtained at various frequencies by various studies. The figure shows that the occlusion effect occurs for frequencies up to ~ 1000 Hz, and that it is largest at lower frequencies. The size of the occlusion effect also varies considerably among individuals. This variability is reflected by the differences among the means shown in the figure. There is a long-standing controversy about whether the best vibrator placement is at the forehead or at the mastoid, and it has not been definitively resolved. Forehead placement has several advantages in terms of higher test-retest reliability and lower intersubject variability; however, the sizes of these benefits are probably too small to be of serious clinical significance (Studebaker 1962; Dirks 1964). A practical forehead advantage has to do with the fact that mastoid vibrators can shift rather easily, and on rare occasions even pop off. In contrast, forehead placement is very stable, provided the vibrator is held in place with a holder that encircles the head. Another advantage of forehead placement has to do with the middle ear component of bone-conduction provided by the inertial lag of the ossicular chain (Chapter 2). Recall that this component involves a relative movement of the ossicles in the right–left direction with respect to the head. At low frequencies, the side-to-side motion of the middle ear bones is activated by the right–left vibrations produced by a mastoid bone vibrator, but not by the front–back vibration caused by a forehead bone vibrator. Thus, the middle ear component makes a bigger contribution to the normal bone-conduction threshold (which we call 0 dB HL) with mastoid placement than with forehead placement. By interfering with the ossicular lag mechanism, some conductive disorders can elevate bone-conduction thresholds to the extent that they depend on the middle ear component. Hence, conductive pathologies have a bigger effect on bone-conduction thresholds with mastoid placement than at the forehead (Link & Zwislocki 1951; Studebaker 1962; Dirks & Malmquist 1969). This is undesirable because we like to think of bone-conduction thresholds as testing the cochlea directly. In spite of various forehead placement advantages, mastoid placement enjoys a practical advantage that is of overwhelming clinical relevance. The strength of the vibration that is needed to reach threshold is smaller when the vibrator is on the mastoid than when it is on the forehead. This was shown in Table 4.5 of Chapter 4, where the reference force levels were smaller for the mastoid than for the forehead. Because it takes less vibratory energy to reach threshold at the mastoid, the maximum hearing level produced by a bone vibrator will also be greater at the mastoid than on the forehead. For example, the highest testable bone-conduction threshold at some frequency might be 70 dB HL at the mastoid but only 55 dB HL at the forehead. Hence, a wider range of bone-conduction thresholds can be tested with mastoid placement. For this reason, we often say bone-conduction has a wider dynamic range at the mastoid than on the forehead. A second advantage of mastoid placement has to do with the concept that there is no interaural attenuation (IA) for bone-conduction, so that the signal reaches both cochleae equally. This is really a half-truth. There is no IA for bone-conduction at the forehead, but the fact is that IA for mastoid bone-conduction is often as much as 15 dB at 2000 Hz and 4000 Hz (see Chapter 9). For example, suppose a patient has 4000 Hz air-conduction thresholds that are 50 dB HL in the right ear and 65 dB HL for the left. The forehead bone-conduction threshold will be 50 dB HL, reflecting the better sensitivity of the right cochlea, but there is still the possibility of a 15 dB air-bone-gap in the left ear. We shall see in Chapter 9 that this situation requires retesting with masking. On the other hand, it is quite possible that the bone-conduction thresholds will be 50 dB HL at the right mastoid and 65 dB HL at the left mastoid. Hence, the existence of IA for mastoid bone-conduction is an advantage for mastoid placement because it can avert the need for unnecessary masking under some conditions. Some audiologists routinely test forehead bone-conduction while the patient is also wearing the air-conduction headset. This technique is called “forehead-occluded bone-conduction.” One argument for this arrangement is that the dynamic range problem that comes with placing the vibrator at the forehead can be overcome by a boost in the bone-conduction signal that results from covering the ears with the earphones, due to the occlusion effect. However, the occlusion effect is simply too variable for this argument to be plausible. This approach does facilitate some aspects of testing because the patient is set up for all tests from the start, but it tends to be quite uncomfortable after a while. You want to establish a working rapport with the patient, but this is certainly not the only reason to talk to him before testing. A case history should be taken at this point. If a case history form was completed in advance, then this is the time to review it with the patient for clarification of pertinent details. Always ask if there have been any changes since the last time he was seen. This is also the time when the clinician observes the patient to develop a clinical picture of his auditory status, communicative strategies, and related behaviors. For example, does the patient favor one ear, watch the talker’s face and lips, lean toward the talker, ask for repetition, respond as though a question was misheard, have aberrant speech or voice characteristics, or look to his wife for clarification? These are only a few of the questions that go through the clinician’s mind during the pretest interview. The information gathered during the interview will be combined with the formal test results to reach a clinical audiologic impression, and will be important in planning the patient’s aural rehabilitation. In addition, the impressions gathered here will be useful when giving the patient test instructions, and in making other decisions about test administration. The clinician should assess whether there are any apparent structural abnormalities and asymmetries of the head in general and the ears in particular, and perform an otoscopic inspection prior to testing. For example, is there evidence of active ear disease, eardrum perforations, atresia, exostoses, external ear abnormalities, or impacted cerumen (see Chapter 6)? Besides leading to appropriate referrals, this information may affect how testing is done and/or interpreted. Test procedures will have to be modified if there is a collapsing ear canal or a mastoid process that cannot properly support a bone-conduction vibrator. The patient should be seated in a reasonably comfortable chair. Armrests are very desirable because they make almost every kind of response method (e.g., hand or finger raising) easier for the patient. Chairs that swivel or lean back should be avoided because the movements are noisy and distracting, and can pose a safety problem for some patients. Whether the patient should be seated facing toward or away from the tester has always been a controversial question. Many audiologists prefer to have the patient seated with her back to the clinician so that she will not receive inadvertent clues about when test signals are being presented. The unintended cues might come from seeing (1) the tester’s behavior (e.g., changes in facial expression or head position while presenting a tone) or (2) the reflections of indicator lamps on the audiometer that light up when the interrupter button is pressed. The other point of view is that the patient should face the clinician for several reasons. (1) Subtle patient behaviors that can affect the test outcome can be observed. For example, the clinician can notice eye movements or the beginnings of incomplete finger motions when the tone is presented 5 dB lower than the level where the patient actually decides to raise her hand. This often means that the patient is being too strict about when to respond, and therefore she should be reinstructed. (2) Many patients need encouragement and/or retraining during the test. Reinforcing correct response behaviors and retraining the patient is more effective and more pleasant when you can see one another. (3) Most speech audiometric tests (Chapter 8) involve verbal responses spoken by the patient. This means that the accuracy of the audiologist’s own hearing can affect the test results. Hence, being able to see the patient’s lips and face makes it more likely that the results are due to the patient’s hearing ability and not due to errors made by the clinician. But what about inadvertent cues? The answer to this question is that indicator lights are easily covered (as is one’s mouth if live-voice speech audiometry is being done), and that controlling one’s own behavior is a valuable skill for clinicians to develop. Moreover, the ability to control the apparent cues provided by one’s facial expressions can be a valuable clinical skill when dealing with patients who have functional hearing losses. Functional, or nonorganic, hearing impairments are discussed in Chapter 14. As a compromise, the patient may be seated so that she faces the clinician at an angle between full face and profile. This permits the clinician to see the patient, but it limits the patient to a peripheral view of the clinician. The patient can indicate that she heard a tone by pushing a response button that causes a response lamp to light up on the audiometer, raising her hand or finger, or verbally (e.g., saying “yes” when a tone is heard). The response is expected to have two components: an on response (e.g., finger is raised) when the tone begins, and an off response (e.g., finger is lowered) when the tone stops. Because test tones are presented in ascending levels starting from below the patient’s threshold, there is often a brief hesitation or latency when the patient responds to the first (faintest) tone. However, there is usually no delay in responding to subsequent, higher-level tones. Silent responses like hand (or finger) raising and pushing a button are preferable for two reasons: (1) They are clear-cut actions that give the tester an unambiguous indication of when the patient heard the tone. (2) They do not interfere with the ability to hear the test tones because they are silent. Of course, modified and special modes of responding are often needed for young children and for difficult-to-test patients of various kinds. Although the ASHA (2005) guidelines do permit verbal responses (e.g., saying “yes”), it is recommended that they should generally be avoided, if possible. The obvious reason for avoiding verbal responses is that test tones will be masked by the patient’s own voice if she happens to respond while a tone is being presented. A second reason is that some patients do not limit themselves to saying “yes” when a tone is heard. Some patients occasionally say “no” when a tone has not been heard for a while. Others add comments like “I’m not sure” or “I think I hear it,” thus increasing the chances that the patient will be talking while the tone is being presented. Comments such as these also force the tester to decide whether the tone was heard instead of keeping this decision with the patient. Some clinicians have the patient indicate the ear in which the tone was heard, others consider this approach to be undesirable, and still others ask the patient to indicate laterality on an ad hoc basis. The simplest approach for routine cases is probably to avoid the issue of laterality when instructing the patient, and to give her the option of indicating sidedness if she asks. Having the patient indicate the sidedness of the tone in an appropriate manner is often useful in special cases and with children. The patient must know exactly what to do during the test. For this reason, test instructions should be explicit and clear. However, they also must be appropriate to the patient, which means that the same set of instructions cannot be given to every patient. Always remember that patients are calmer and more cooperative when they understand what is going on, what to expect, and what they have to do. With these points in mind, the following is a typical set of instructions for pure tone, air-conduction testing: The idea of this test is to find out the softest sounds you can hear. You’re going to hear tones from these earphones. There will be many tones, one at a time. Some of the tones will be loud, but most of them will be very faint, and many of them will be too soft to hear. Your job is to raise your hand every time you hear a tone, no matter how faint it is, and to put your hand down whenever you don’t hear any tones. We will test one ear and then the other one. Remember, raise your hand every time you hear a tone, no matter how faint it is. Do you have any questions? Several techniques to obtain pure tone thresholds are accepted by the audiological community, such as the Carhart-Jerger (1959) modification of the Hughson-Westlake (1944) approach, and the ANSI (2004) and ASHA (2005) testing methods. That these respected methods are not identical highlights the point that there is no one method that is the singularly “right” way to test pure tone thresholds. However, these methods agree on the most important issues, and their similarities reveal a consensus of general approach within the audiological community. The clinical threshold for a tone is generally defined as the lowest hearing level at which it can be heard for at least 50% of the presentations on ascending runs. In addition to this, the Carhart-Jerger (1959) method requires at least three responses at this level, whereas the ANSI (2004) and ASHA (2005) approaches require at least two, therefore taking less time. The two- and three-response rules appear to yield essentially the same results (Harris 1979; Tyler & Wood 1980). The typical test signal is a continuous pure tone lasting 1 to 2 seconds. However, pulsed tones and warble tones may also be used (ASHA 2005), and provide similar results with an easier listening task for many patients, especially those with tinnitus (Hochberg & Waltzman 1972; Mineau & Schlauch 1997; Burk & Wiley 2004; Franklin, Johnson, Smith-Olinde, & Nicholson 2009). Testing begins by familiarizing the patient with a 1000 Hz test tone and making a ballpark guesstimate of approximately where the threshold might be. The traditional Carhart-Jerger (1959) method did this by first presenting a test tone lasting 1 to 2 seconds at ~ 30 dB HL if the patient seems to be normal, or at 70 dB HL if he appears to have a hearing impairment. If the patient does not respond to the first tone, then its level would be raised in 15 dB steps until the tone is heard. Contemporary guidelines (ANSI 2004; ASHA 2005) recommend using either of two methods. One technique involves first presenting a test tone lasting 1 to 2 seconds at 30 dB HL, which is followed by 50 dB HL if the 30 dB HL tone was inaudible. If the patient still does not hear the 50 dB HL tone, then its level is raised in 10 dB steps until the patient responds. The alternative approach is to begin presenting the tone at the lowest attenuator setting, and then gradually increase its level until the patient responds. After a ballpark estimate is obtained, the threshold search procedure is then begun, which uses the following strategy: 1. Each test tone is presented for 1 to 2 seconds. 2. We want the threshold to be approached from below, so testing starts at a level that is known to be below the patient’s threshold. This can usually be done by simply setting the tone to 10 dB below where the patient responded during familiarization, and then present it to him. 3. The level of the tone is then raised in 5 dB steps until the patient responds. 4. The tone is now decreased by 10 dB (or 15 dB) and presented again, in which case it should again be inaudible. This is done so that the threshold can again be approached from below. [Sometimes a patient will respond at this lower level. When that happens the tone is decreased another 10 dB (or 15 dB) and presented, and so on, until it is inaudible.] 5. The level of the tone is then raised in 5 dB steps until the patient responds. Steps 3 and 4 are repeated until the clinical threshold criterion is achieved. In other words, the clinician lowers the level of the next tone by 10 dB after every “yes” response, and raises the level of the next tone by 5 dB after every “no” response. The tone presentations should be reasonably irregular in their timing rather than following any rhythmic pattern. In summary, the pure tone testing procedure can be thought of as having two parts. First, we raise or lower the intensity of the tone in fairly large steps to quickly find the ballpark location of the threshold. Once we know the general location of the threshold, we switch to a more formal threshold determination strategy in which the threshold is approached from below in 5 dB steps. This involves two tactics, which are illustrated in Fig. 5.4: (1) Whenever the patient does not hear the tone (–), we increase the level of the next tone by 5 dB (“up 5” after a “no”). (2) Whenever the patient hears the tone (+), we decrease the level of the next tone by 10 dB (“down 10” after a “yes”). It is no wonder this is known as the up-5 down-10 technique. The threshold search procedure itself is easily understood by going through a typical example. The example shows how to do the procedure and also reveals why it works. We will illustrate only one “threshold search.” However, the student should remember that a separate threshold is needed for every test frequency for both ears, and for both air and bone-conduction. The threshold search procedure is therefore performed many times for each patient. The example is portrayed in Fig. 5.5. The numbers along the abscissa represent each of the individual presentations of the test tone, or trials. The ordinate shows the hearing levels of the tones presented to the patient. It is assumed that all of the necessary preliminary procedures have been done, and that we are ready to begin the actual testing process. We begin by presenting the tone at 30 dB HL. The patient does not respond, implying that 30 dB was not heard. This situation is indicated by the – for trial 1 at 30 dB HL. Since the 30 dB HL starting level is not audible, we increase the level of the tone to 50 dB HL for the next trial. This time the patient does respond, indicated by the + for trial 2 at 50 dB HL. We can now estimate that the threshold is between 30 and 50 dB HL. If the patient did not hear the 50 dB HL tone, we would have raised the level in 10 dB steps until he did. On the other hand, if the patient heard the tone at the initial level of 30 dB HL, we would have lowered it in 10 dB steps until he could no longer hear the tone. In either case, the idea is to rapidly find the approximate range of the threshold, so that we do not waste any effort. Fig. 5.4 Conceptual illustration of the “up-5 down-10” technique typically used in pure tone audiometry. Because the tone was heard at 50 dB HL in trial 2, the tone is lowered by 10 dB and is next presented at 40 dB HL in trial 3 (“down 10” after a “yes”). The patient hears the tone (+) at 40 dB in trial 3, so we drop its level by 10 dB and present it at 30 dB HL in trial 4. The patient responds to the tone (+) at 30 dB HL in trial 4, so we again reduce its level by 10 dB and present the tone at 20 dB HL in trial 5. The patient does not hear the 20 dB HL tone (–). The rule now tells us to raise the level by 5 dB to 25 dB HL for trial 6 (“up 5” after a “no”). The tone is not heard at 25 dB HL in trial 6 (–). Hence, it is presented 5 dB higher in trial 7. The patient does not hear the 30 dB HL tone in trial 7. Thus, the “up 5” rule calls for trial 8 to be presented at 35 dB HL, which is heard (+) by the patient. Notice how trials 5 through 8 constitute an “ascending run” that ends in a “yes” (+) response for trial 8. In other words, we have approached the response from below. Fig. 5.5 Hypothetical threshold search for a patient whose threshold is 35 dB HL. A + indicates the patient heard the presentation, and a – shows that the patient did not hear the tone. Notice that the hearing level of a trial is raised by 5 dB following a – (the “up 5” rule) and is lowered by 10 dB following a + (the “down 10” rule), which causes the tester to search for responses in a series of ascending runs. The + response at 35 dB HL in trial 8 means that the tone must be presented 10 dB lower, at 25 dB HL, in trial 9 (“down 10” after a “yes”). The patient does not hear the tone at 25 dB HL in trial 9 (–), or at 30 dB HL in trial 10 (–), but he does hear the tone at 35 dB HL in trial 11 (+). Thus, we have again approached a response from below in 5 dB steps, completing a second ascending run (trials 9–11)) ending in a + outcome at 35 dB HL. This completes the threshold search for this tone using a criterion of two responses out of four theoretical presentations, and establishes the threshold to be 35 dB HL. In other words, 35 dB HL is the patient’s threshold because it is the lowest level at which he responded to the tone at least 50% of the (theoretically four) presentations, with at least two responses at that level. We would still need one more ascending run if we wanted to use a three-response criterion. This exercise is shown in trials 12 through 14. Because 35 dB HL was heard in trial 11, the “down 10” rule now calls for us to present trial 12 at 25 dB HL, where we find no response (–). Trial 13 is thus presented at 30 dB HL according to the “up 5” rule. Because the tone is still not heard (–) at 30 dB HL, it is raised again by 5 dB, to be presented at 35 dB HL in trial 14. The patient hears the 35 dB HL tone in trial 14 (+), completing a third ascending run. In other words, 35 dB HL is the lowest level at which the patient responded to at least 50% of (theoretically six) presentations, with at least three responses at that level. Clinical pure tone thresholds are routinely tested in the frequency range from 250 to 8000 Hz. The threshold at 125 Hz is also obtained when there is an appreciable hearing loss in the low frequencies. High-frequency audiometry, which involves testing at frequencies above 8000 Hz, is sometimes done for special purposes, such as when monitoring patients who are at risk for ototoxicity. Pure tone thresholds are routinely tested separately for each ear, followed by bone-conduction testing. For air-conduction, the right ear is tested before the left ear unless there is reason to believe that one ear is better than the other. In the latter case, the apparently better ear is tested before the poorer one. Of course, special circumstances often call for flexibility in one’s testing approach. Typical examples include evaluating young children, difficult-to-test patients, or those with poor reliability. Air-conduction traditionally has been tested for the octave frequencies from 250 to 8000 Hz. In this case, pure tone thresholds are obtained in the following order for each ear: The 1000 Hz retest is done as a reliability check and is expected to be within ±5 dB of the first 1000 Hz threshold in that ear, and the lower (better) of the two is considered the threshold. The semioctaves (750, 1500, 3000, and 6000 Hz) are tested whenever there is a difference of ≥ 20 dB between two adjacent octave frequencies (e.g., 3000 Hz is tested if the thresholds at 2000 and 4000 Hz differ by 20 dB or more). In contrast, the current ASHA (2005) guidelines include 3000 and 6000 Hz among the regularly tested frequencies. Here, the test order for each ear is In this case, 750 Hz is tested if the 500 and 1000 Hz thresholds differ by ≥ 20 dB, and 1500 Hz is tested if the 1000 and 2000 Hz thresholds are ≥ 20 dB apart. So, which group of frequencies should one routinely use? In principle, the author is inclined to side with the ASHA (2005) guidelines, but it makes sense to weigh the value of the added information gained by an extra pure tone threshold against the cost of obtaining it. Patient fatigue and clinician time must be considered because other tests will follow, and pure tone thresholds often have to be repeated with masking. The ANSI (2004) method includes the commonsense recommendation to include semioctaves depending on the intended purpose of the test. Thus, it seems prudent to include 3000 and 6000 Hz when conditions make this feasible, and certainly when they are needed for the intended purpose of the test. For example, we will see in a later chapter that 3000 Hz is often needed for medico-legal purposes. However, it is essential to test semioctaves when one finds a ≥ 20 dB spread between adjacent octave frequencies. Bone-conduction is usually tested from 250 to 4000 Hz, traditionally in the following order: However, the ASHA (2005) guidelines add 3000 Hz, in which case the test order becomes: Many audiologists do not perform the 1000 Hz reliability check or test semioctaves by bone-conduction unless there is reason to do so. However, a 3000 Hz bone-conduction threshold is quite desirable if that frequency was tested by air-conduction. A false-positive response means that the patient responds when he should not have responded. In contrast, a false-negative response means that he fails to respond when he should have responded. False-negative responses can occur for several reasons. Equipment problems or tester errors can prevent a signal from reaching the patient, or cause it to be presented in a manner that was not intended. Some patients are confused by tinnitus if their ear noises are similar to the test tones. The patient may not have fully understood the instructions or learned the proper mode of response. For example, some elderly patients have extremely strict criteria for when they will consider the tone present (i.e., they want to be very sure the tone is there) before they will respond. As we shall see in Chapter 14, there are also patients who do not respond because they are trying to make their hearing appear to be worse than it really is for conscious or unconscious reasons. False-negative responses can also occur for technical reasons, such as collapsed ear canals and standing waves, which are discussed later. False-positive responses make thresholds difficult to obtain and unreliable from test to test. Some false positives are caused by tactile stimulation and/or acoustical radiations (discussed later). Others are the result of confusion between test tones and tinnitus. Many false-positive responses are behavioral and are often due to misunderstood or improperly learned instructions, or to very lax response criteria. Behaviorally based false positives can often be reduced by refamiliarizing the patient with the test tones and reinstructing him about exactly when and how to respond. The tester can inadvertently encourage false positives by presenting tones rhythmically. Remembering that most of the tones are hardly audible to begin with, learning the tester’s rhythm makes the patient expect tones at certain times, thus biasing him to respond at those times. A difficult situation can develop if a patient’s false response coincidentally occurs at just the “right” moment after a real tone presentation. Because the false response had every appearance of being a real one, the tester might even confirm its correctness for the patient. This problem can interact with rhythmic tone presentations. Not only does presenting the tones in a rhythmic pattern cause the patient to expect tones at certain intervals, but a rhythmic pattern also makes it more likely that the false responses will occur at the “right” times. Several strategies can minimize equipment problems and tester errors. Daily equipment checks are the first line of defense. Errors due to incorrect equipment settings can be minimized by establishing a set of “start-up” positions that are always the same (e.g., frequency at 1000 Hz, HL dial at 0 dB, input selector to “tone,” output selector to right earphone, etc.). By always resetting the audiometer to these initial setup positions after each patient, the clinician can avoid errors caused by a control inadvertently set at the wrong position. An added benefit of resetting the audiometer is never having a control in the wrong position. Simply returning everything to the start-up position and then setting up the test from a “clean slate” is a lot easier and more effective than looking for the error. The ultimate version of this strategy is possible with digital audiometers that automatically return to their default positions when they are turned on. One should not be too eager to use the reset button or to turn the power off and (after waiting) on again. However, this can be the only solution when a digital audiometer “locks up” because of programming bugs or design flaws. The patient’s thresholds at each frequency are recorded on an audiogram, which is usually shown as a graph. Many audiologists record the information for both ears on the same audiogram form, whereas others use a separate graph for each ear. The audiogram form and symbols recommended by ASHA (1990) are shown in Fig. 5.6. Frequency is shown on the abscissa, going from 125 Hz on the left to 8000 Hz on the right, and it is customary to label the frequency axis along the top. Notice that octaves are equally spaced. In other words, the distance covered by any doubling of frequency (e.g., 125 to 250 Hz, 1000 to 2000 Hz, 1500 to 3000 Hz, and 4000 to 8000 Hz) is always the same. This means that the frequency scale on an audiogram is logarithmic. Hearing level is shown in dB HL (ANSI/ISO or ANSI S3.6-2010) along the y-axis, with intensity increasing from –10 dB HL at the top to the maximum level (120 dB HL in the figure) at the bottom.
Hearing Threshold
Air-Conduction Testing
Bone-Conduction Testing
Occlusion Effect
Mastoid versus Forehead Bone-Conduction
Pretesting Issues and Considerations
Talk Before You Test
Look Before You Touch
Orientation of the Patient
Patient Responses
Test Instructions
Determining the Pure Tone Threshold
Familiarization and Ballpark Threshold Estimate
Threshold Search
A Step-by-Step Example
Test Frequencies and Testing Order
1000, 2000, 4000, 8000, retest at 1000, 500, 250 Hz
1000, 2000, 3000, 4000, 6000, 8000, retest at 1000, 500, 250 Hz
1000, 2000, 4000, 1000, 500, 250 Hz
1000, 2000, 3000, 4000, retest at 1000, 500, 250 Hz
False Responses
Avoiding Equipment Problems and Tester Errors
Recording Pure Tone Thresholds on the Audiogram
The Audiogram Form
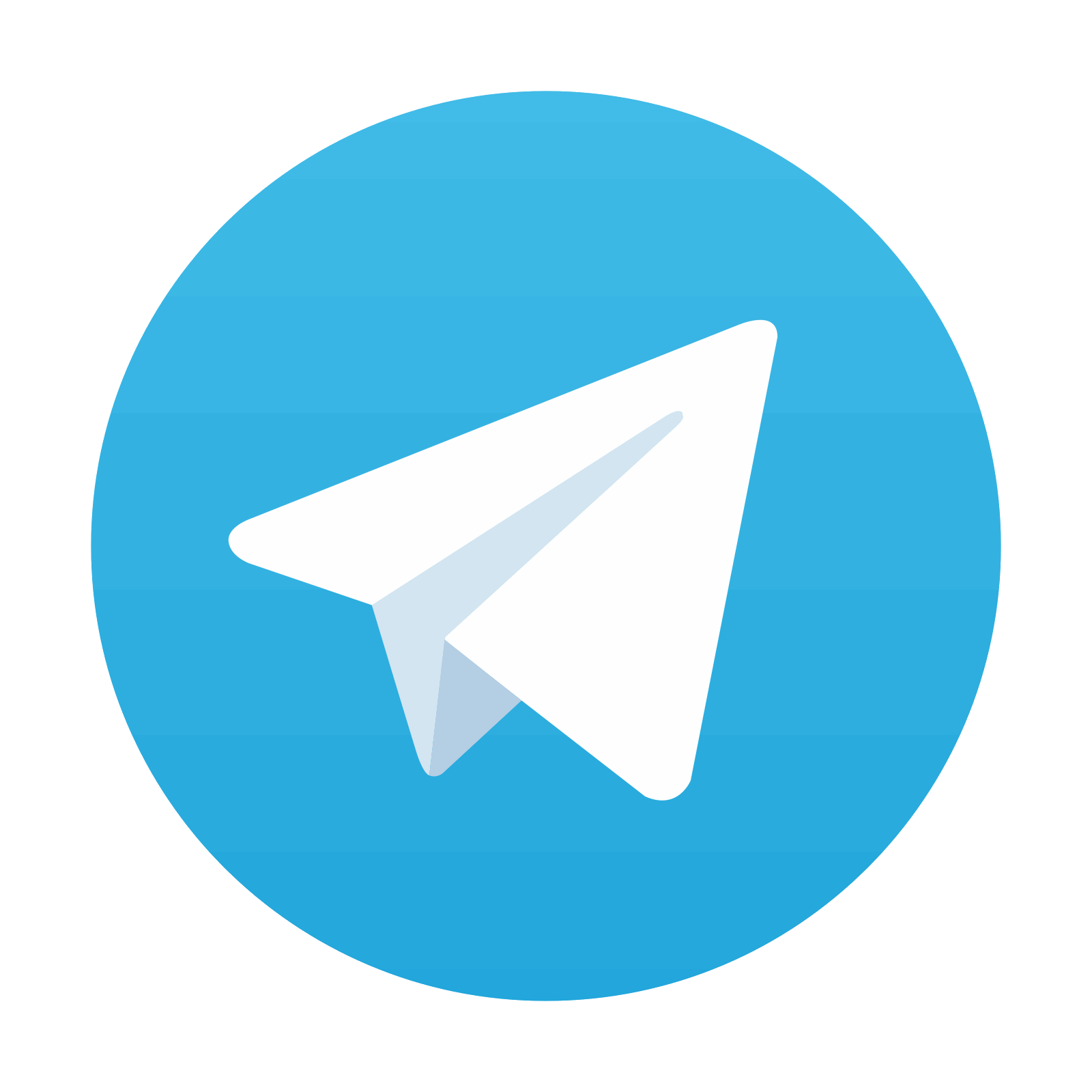
Stay updated, free articles. Join our Telegram channel

Full access? Get Clinical Tree
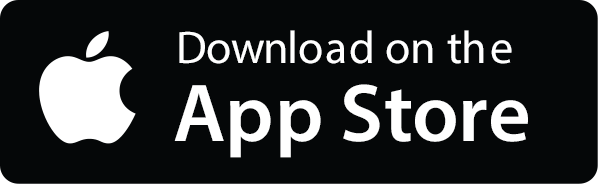
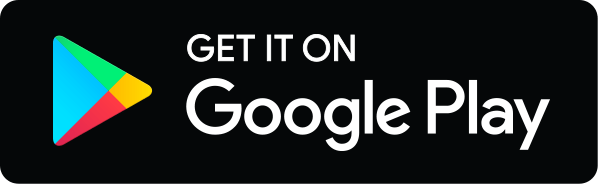