CHAPTER 41 Physiology of Olfaction
Supported in part by NIH Grant NIDCD9-P01 DC00220.
Anatomy of Olfactory Stimulation
Nasal Passageways
Experiencing an odor is a result of input from the olfactory, trigeminal, glossopharyngeal, and vagus nerves. Apparently, the properties of any given odorant determine the particular “mix” of these various inputs. Olfactory nerve (cranial nerve I) stimulation, which is necessary for identification of most odorants, depends on the odorant molecules’ reaching the olfactory mucosa at the top of the nasal cavity. Although molecules can reach the olfactory cleft by diffusion, essentially olfaction requires some type of nasal airflow, usually as part of inhalation (orthonasal flow). During eating, there is a retronasal flow of odorant molecules that stimulate the olfactory receptors at the top of the nose and contribute greatly to the flavor of the food.1,2 This airflow can be very small, such as that generated by the mouth and pharyngeal motion.3–5 Additional data from a large-scale model indicate that at physiologic airflow rates, approximately 50% of the total airflow passes through the middle meatus, with approximately 35% flowing through the inferior meatus (Fig. 41-1). About 15% flows through the olfactory region.6
Mathematical models of the nose, created from digitized computed tomography (CT) anatomy slices and predicted mass transport functions, can predict odor intensity for varying amounts of olfactory epithelium, the character of the carrier gas, and the solubility of the odorant molecules.7–9 These theoretical results show agreement with human and animal experimental data.
The effect of a rapid change in flow velocity, such as with a sniff, on the in vivo airflow pattern remains unknown. Scherer and colleagues6 have found in their large-scale model studies that the percentage and velocity of airflow to the olfactory region are similar for various steady-state airflow rates in the physiologic range. The fact remains, however, that sniffing is an almost universally performed maneuver when a person is presented with an olfactory stimulus. It is possible that its purpose is to momentarily increase the number of olfactory molecules in the olfactory cleft by means of a transient change in the airflow pattern of the nose. A sniff also may allow the trigeminal nerve to alert the central olfactory neurons that an odorant is coming. Sniffing duration, flow velocity, and volume are quite different among subjects but remain remarkably constant for any one subject.10 Furthermore, Laing11 has shown that different sniffing paradigms do not improve a subject’s olfactory perception. The naturally chosen sniff seems to be optimal for that subject’s nasal anatomy.
For molecules to reach the olfactory area, they must pass through the tall but narrow nasal passageways. The epithelium lining the walls of these passageways is wet, has variable thickness, and is aerodynamically “rough.” Schneider and Wolf12 have observed that olfactory ability is best when this epithelium is moderately congested, wet, and red, such as during an upper respiratory infection. Furthermore, olfactory ability seems to improve with narrowed nasal chambers,13,14 but the changes in nasal patency that occur during the natural rhythmic engorgement and thinning of the nasal epithelium (nasal cycle)15 do not have any effect on olfactory ability.16–18
The sorption of molecules to these mucus-lined walls extracts some of them from the air stream and increases their travel time. This process could influence the spectrum of chemicals reaching the olfactory cleft or spread their arrival over time. Moncrieff19 described this phenomenon in the variable times required for odorants to traverse the nasal passageways of sheep. The sorption of molecules may separate or sort the odorants before they reach the olfactory mucosa. Highly sorbable chemicals may have minimal or no odor simply because they sorb to the nasal walls before they reach the olfactory cleft, thereby accentuating the trigeminal component. Could it be that our world would smell different if we had no external nose to lengthen the path of the olfactory region? Does an animal with a long snout, such as the dog, smell the world differently as a result of these sorptive effects?
Olfactory Mucus
After the odorant molecules reach the olfactory region, they must interact with the mucus overlying the receptor cells. The mucus apparently comes from both Bowman’s glands deep in the lamina propria (only of serous type in humans)20,21 and the adjacent respiratory mucosa (goblet cells). Researchers have observed that the supporting (sustentacular) cells of the human olfactory epithelium are not histologically equipped to secrete mucus.21–23 The supporting cells of most other species, however, do secrete mucus, often in response to odorants.20,24–26
The partitioning of an odorant’s molecules between the air phase and the mucus phase is most certainly another determinant of its being perceived. To reach the olfactory receptors, the odorant molecules must be soluble in the mucus but, as Laffort and associates27 argue, not so “strongly captured” that they are unable to interact with the receptors. In addition, changes in the thickness or composition of the mucus can influence the diffusion time required for odorant molecules to reach the receptor sites.28 Adrenergic, cholinergic, and peptidergic agents have caused these changes in the mucus overlying the olfactory receptors through their effects on the secretory activities of the mucosal glands. Moreover, these same agents have influenced the sensitivity of the olfactory receptor cells themselves.29–31
Once in the olfactory mucus-epithelial system, the rate at which the odorant is cleared also is important. Hornung and Mozell32 showed that 79% of a radioactively labeled odorant (butanol) remained trapped in the mucus 30 minutes after inspiratory exposure, whereas radioactively labeled octane cleared rapidly. The mucus may exert a differential role in deactivating, removing, or desorbing odorants from the olfactory area.
Olfactory Epithelium
Located 7 cm inside the nasal cavity, the olfactory sensory neurons are protected in a 1-mm-wide crevice of the posterosuperior nose. At the epithelial surface, these bipolar neurons are exposed to the outside world through their dendrites and cilia. Proximally, axons of these same neurons synapse at the base of the brain (olfactory bulb). Although the olfactory receptor region is a solid sheet of olfactory mucosa housed in a protected environment in human fetuses and laboratory animals, a number of research groups have shown that there is mixing of olfactory and respiratory epithelial tissues in adult humans (Fig. 41-2).22,33–36 The number of these clumps of respiratory epithelium, which are found in the olfactory area, increases with age, suggesting that a loss of primary olfactory neurons at least partially explains the decreased olfactory ability associated with aging.37
The human olfactory epithelium covers an area of roughly 1 cm2 on each side. The epithelium is pseudostratified columnar, and it rests on a vascular lamina propria with no submucosa (Fig. 41-3). In most mammals studied, four main cell types have been identified (Figs. 41-4 and 41-5): ciliated olfactory receptors, microvillar cells, supporting (sustentacular) cells, and basal cells.22,23,35,38 Research that uses immunohistochemical staining of olfactory elements continues to provide information on growth, maturation, function of specific cellular elements, and similarities with other neural tissue.39–45 One possible use for this technique may be to clarify the etiology of the patient’s olfactory dysfunction and possibly offer a prognosis regarding improvement of olfactory ability.18,46 Equally important to analysis of the epithelium is the evaluation of the olfactory axon bundles that travel within the lamina propria of the mucosa. Given that these bundles arise from receptor neurons distributed along a region of the epithelium, the identification of mature olfactory axons in comparison with the abundance of immature axons or collagen replacement within these bundles suggests a healthy functional olfactory mucosa. This may be a truer representation of the status of the epithelium as a whole than what can be deduced from analysis of the epithelial surface alone in a small biopsy specimen.47
The olfactory receptor neuron is bipolar and has a club-shaped peripheral “knob” that bears the cilia (Fig. 41-6). The surface of human olfactory epithelium is covered with cilia extending from the dendritic knobs of olfactory receptor neurons, but two electron microscopic studies show that there are no dynein arms on these cilia.21,23 The researchers in these studies conclude from this observation that neither dynein arms nor motility is essential for human olfaction. After widening for the nucleus, the olfactory receptor neuron tapers to a long, thin, nonmyelinated axon that can travel several centimeters to the olfactory bulb. Bundles of these fibers form in the lamina propria and become surrounded as a group by the plasma membranes of the Schwann-type ensheathing cells, forming the olfactory nerve (cranial nerve I), which traverses the 15 to 20 foramina in the cribriform plate to synapse in the bulb. Allison and Warwick48 estimate the rabbit to have approximately 50 million olfactory axons, whereas Jafek21 estimates humans to have only 6 million bilaterally.
The olfactory ensheathing cells are unique in that they share characteristics that are common with Schwann cells and central glial cells. Because olfactory neurons have the ability to regenerate and make functional synapses with the olfactory bulb, there has been interest in the role of the ensheathing cells in this process and its possible therapeutic potential for repair of peripheral neuronal injury. The glial-type cells that ensheathe olfactory neurons support axonal growth of both olfactory and nonolfactory neurons.49 Because of their ability to promote long-distance growth of regenerating axons in vivo and their ability to remyelinate axons of the adult mammalian spinal cord, these cells have received great interest as potential agents for reversing spinal cord injuries and demyelinating diseases.50 Guntinas-Lichius and others51 compared transplants of olfactory mucosa with buccal mucosa laid over a primary reanastomosis of the facial nerve in rats. Use of the olfactory mucosa resulted in better vibrissal movements, less branching of motor neurons, and greater accuracy of reinnervation.
The microvillar cell occurs about one tenth as often as the ciliated olfactory neurons.21,22 The cell body is flask-shaped, is located near the epithelial surface, and has an apical membrane containing microvilli that project into the mucus overlying the epithelium (see Fig. 41-5).23,52 The deep end of the cell tapers to a thin, axon-like cytoplasmic projection that proceeds into the lamina propria. Although there is no electrophysiologic evidence that these cells respond to chemical stimuli, Rowley and others38 have hypothesized that the cells are a morphologically distinct class of sensory receptors. The evidence for this hypothesis is the backfilling of a cytochemical tracer macromolecule (horseradish peroxidase) into the ciliated olfactory receptors and the microvillar cells when it is injected into the olfactory bulb.
In among these two receptor-type cells are the supporting or sustentacular cells. These tall cells have an apical membrane that joins tightly with the surface of the receptor cells and the microvillar cells. They seem positioned to separate the receptor cells from each other; however, intimate apposition between receptor cells does occur.21–23 Whether this proximity allows some receptor cells to influence each other’s firing patterns is unknown. Sustentacular cells do not generate action potentials, nor are they electrically coupled to each other53,54; thus, they do not seem to contribute directly to the olfactory transduction process and the elicitation of generator potentials by odorant stimuli. However, they do appear to play a role in ion and water regulation55 and, along with Bowman’s gland duct cells, contain xenobiotic enzymes such as cytochrome P-450 that likely contribute to odorant metabolism.56
Deep to all of these cells, the basal cells sit along the basal lamina. The basal cells are divided into two groups of replicating cells. The horizontal basal cells are just above the basal lamina, and the globose basal cells are positioned between the horizontal basal cells and immature neurons. The globose basal cells seem to be responsible for the continuous replacement of olfactory receptor neurons; however, during severe insult, they may repopulate the nonneuronal components of the epithelium as well.57–60 This function identifies the globose basal cell as the bona fide stem cell located within the olfactory mucosa, but new evidence for the horizontal basal cell as the neural stem cell has also been presented.61 The ongoing renewal of these special sensory neurons is not known to occur in other sensory systems. The replication cycle is between 3 and 7 weeks.62,63 When the new receptor cell forms, it also projects its axon to the olfactory bulb, where it synapses with second-order neurons, thereby ensuring continual olfactory function and continual olfactory neuron replacement.
Vomeronasal Organ
Many mammals have an identifiable pit or groove in the anteroinferior part of the nasal septum that contains chemosensitive cells.64 In most of these animals, a nerve can be identified connecting these cells to the central nervous system, often to an accessory olfactory bulb. In some situations, this system has been receptive to pheromones.65 There have been investigations into the possibility that humans have some type of vomeronasal system.66 Biopsy studies of the nasal mucosa in the small pit often seen along the anteroinferior nasal septum (Jacobson’s organ) show olfactory-like histology but no central connection (Fig. 41-7). Electrophysiologic studies have shown negative action potentials from this vomeronasal area in response to specific chemical stimuli but without a subjective response from individuals tested.67 These same stimuli did not cause negative potentials in the olfactory system. Similar electrical activity elicited by certain compounds directly delivered to the vomeronasal area has been shown to cause changes in blood pressure, heart rate, and hormonal levels.68 Because no identified central brain connection seems to exist, and an accessory olfactory bulb seen in other mammals has never been identified in humans, it is possible that this system functions as a neuroendocrine system, emitting a substance in response to a specific chemical stimulus. Positron emission tomography (PET) scanning after presentation of a putative pheromone show detection of the compound executed in higher cortical regions different from those usually activated in the perception of odors, and in contrast to PET scanning results in the olfactory system, responses to the putative pheromone show gender differences.69 V2R genes encoding for vomeronasal receptors have been found in mammals; however, in humans putative vomeronasal receptors are nonfunctioning pseudogenes.70 It may be that human vomeronasal receptors are unrelated to olfaction and that other mammalian receptors have yet to be identified. Genetic, biochemical, and electrophysiologic data now suggest that some pheromone-based behaviors in vertebrates are mediated through the main olfactory epithelium.71,72 At present, no good evidence for symptom-related importance of the human vomeronasal organ exists, but prudence suggests that this anatomic area should not be disturbed during surgery unless it is necessary.73
Olfactory Bulb
The olfactory bulb lies at the base of the frontal cortex in the anterior fossa (Fig. 41-8). It serves as the first relay station in the olfactory pathway, where the primary olfactory neurons synapse with secondary neurons. These synapses and their postsynaptic partners form dense aggregates of neuropil called glomeruli. In humans, projections of mature olfactory axons into the bulb end in glomeruli as well, but misrouted projections commonly occur, usually into the external plexiform layer.74 In the adult rabbit, approximately 26,000 olfactory axons enter each glomerulus, where they contact roughly 100 second-order neurons.75 This relationship indicates a considerable convergence of information, because these neurons pass from the periphery onto this first central station. (The interconnections among the various glomeruli in one bulb, the interconnections between bulbs, and the afferent and efferent connections with the brain indicate that considerable processing also occurs at the bulb level.)
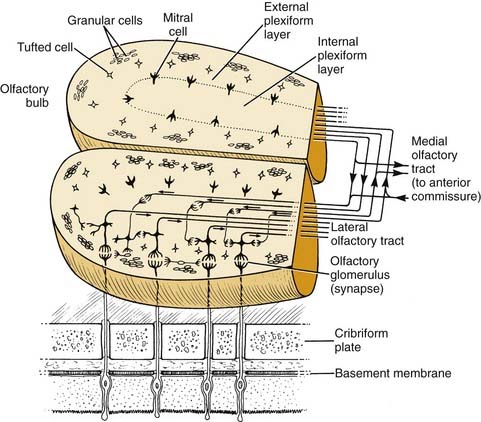
Figure 41-8. Structure of olfactory bulbs and their neural connections to one another, the olfactory mucosa, and the brain.
The neuronal projection of the olfactory mucosa onto the bulb displays some anatomic restrictions but is not strictly point-to-point. In other words, a given region of the bulb receives its most dense input from a particular region of the mucosa,76 but inputs to a particular region of the bulb converge from many receptor cells distributed throughout a certain zone of the mucosa.77 Conversely, a small focus in the epithelium projects widely, but within a restrictive region of the bulb.78 These dense and diffuse inputs possibly represent excitatory and inhibitory influences, which, as in other sensory systems, narrow the neural stimulus representation as the processing moves centrally. Alternatively, the convergence and divergence of projections from neurons in the epithelium may serve to coalesce inputs from receptor cells of sensitivity to odorants. Evidence to support this notion emerges from the coherent activation of single glomeruli or sets of glomeruli by specified odorants.79,80 Therefore, it is clear that the microcircuitry of the bulb is specialized to narrow the spatial pattern of the glomerular activation elicited by an odorant or mixture of odorants.
In line with the regenerative capacity of the olfactory epithelium, there is a mechanism for neuronal replacement in the olfactory bulb. In mammals, the olfactory bulb is closely associated with dividing cells in the lateral ventricles that migrate via the rostral migratory stream (RMS) into the bulb and become mature neurons. In humans, the rostral migratory stream appears to be located around an extension of the lateral ventricle into the olfactory bulb, and at least some of the cells derived from the stream relocate to the periglomerular region.81
Olfactory Connections in the Brain
The more central olfactory connections include the olfactory tubercle, the prepiriform cortex, part of the amygdaloid nuclei, and the nucleus of the terminal stria, with further projections to a number of structures, including the hypothalamus. Although these structures receive olfactory input, they also serve other functions, such as food intake, temperature regulation, sleeping cycles, vision, memory, hearing, and taste. These connections may explain the strong memories and emotional context of various odors. It also is possible that these structures influence the olfactory process by efferent connections.82,83
The organization of like olfactory receptors projecting into the olfactory bulb with the formation of an olfactory map (described later) appears to also occur to some degree in higher cortical areas. In mice, single odorants activate distinct patterns in the anterior piriform cortex. Exposure to different odorants results in different but overlapping maps of activity in the piriform cortex that are similar in individual mice. Odors that are related in structure also stimulate similar patterns of activity within the piriform cortex. These findings suggest an organization of axonal projection and stimulation in response to odorant stimulation within the cortex.84
Common Chemical Sense
Free nerve endings of three cranial nerves—trigeminal (the most important), glossopharyngeal, and vagus—provide added chemoreceptivity in the mucosa of the respiratory tract.85,86 The trigeminal nerves are sensitive to the burn of ammonia and the bite of hot pepper. In the nose, virtually all odorants stimulate olfactory and trigeminal nerves, even when no apparent pungency can be perceived. The peripheral anatomic pathways for these cranial nerves have long been known; however, the central connections that allow their interaction and how they interrelate with other senses are just beginning to be determined.85,87,88 Cometto-Muniz and Cain89 have shown that when tested with ammonia, the common chemical sense behaves more like a total-mass detector than a concentration detector (i.e., at a given concentration, the perceived magnitude increases with the time of presentation). It is even possible that the trigeminal nerve interprets pungent or chemically irritative stimuli as being painful or nociceptive in nature.
Olfactory Transduction and Coding
At each level of the olfactory system, different factors control or shape how the system works. The nose has narrow passageways lined by wet mucus and swept by alternating air currents. The hydrophilic olfactory mucus presents the incoming odorant molecules with constraints of sorption, solubility, and chemical reactivity. Once the odorant molecule is dissolved in the olfactory mucus, another group of events influences whether it can interact with the olfactory receptor cells. Soluble binding proteins, like odorant-binding protein, have been described in air-breathing vertebrates, and it has been suggested that these proteins enhance the access of odorants to the olfactory receptors.90–94 This enhancement is said to be accomplished by binding and solubilizing of hydrophobic odorant molecules, thus increasing their concentration in the environment of the receptor cell by as much as 1000 to 10,000 times more than their concentration in the ambient air.95,96 In addition, these same odorant-binding protein molecules may act to remove odorant molecules from the region of the receptor cell after transduction. Other workers, however, have suggested that mammalian odorant-binding protein is too slow to be able to bind and release the odorant in the mucus.97,98 They conclude that the role of odorant-binding proteins is purely hypothetical and most likely not related to odor protein transport. It also is possible that the olfactory systems of air-breathing vertebrates may have a chemical-sensing system like that of unicellular and multicellular organisms. This system can produce degradative enzymes that transform stimulants into inactive products, and vice versa.99 Therefore, many perireceptor events occur in the olfactory mucus before the odorant actually comes into contact with the cilia of the olfactory receptor cell.
In mammals, it is generally accepted that the actual transformation of odorant chemical information into an electrical action potential occurs as a result of specific interactions between odorant molecules and receptor proteins on the surface of olfactory cilia.100,101 At least the first stages of odorant discrimination occur at the primary neuron level.102–104 This process is mediated by a family of approximately 1000 genes encoding for variations of a seven–transmembrane domain receptor protein.19 The human genome is composed of approximately 30,000 genes, thus making the olfactory receptors the largest gene family in the entire genome.
As this transduction process moves through the receptor cell membrane, several second-messenger systems assist in depolarizing the cell and initiating the action potential. Patch clamp recordings have demonstrated that cyclic adenosine monophosphate (cAMP) and inositol phosphate (IP3) are the primary signaling pathways that can mediate olfactory transduction, depending on the species and the odorants.102,105 In mammals, the olfactory receptors are members of G protein–coupled receptors (Golf), and this G protein seems to be exclusively localized to the olfactory epithelium.106 With the binding of the receptor to an odorant, adenylate cyclase is activated by Golf and converts adenosine triphosphate (ATP) into cAMP. The cAMP then binds to a Na,Ca ion channel to allow influx of these ions. As more channels open, the cell depolarizes, and an action potential is produced (Fig. 41-9). Clinical evidence supporting the cAMP pathway comes from patients with type 1a pseudohypoparathyroidism, who have a deficiency in stimulatory Golf, which is needed for the second messenger transduction, and also have olfactory losses.107–109 With high doses of odorant, and in other parts of the olfactory pathway (like the olfactory bulb), another second messenger system involving nitric oxide (NO)/cyclic guanosine monophosphate (cGMP) has been identified.110,111
Once the peripheral olfactory receptor cells are depolarized, there begins a convergence of electrical information toward the olfactory bulb. In the nasal cavity, a broad, genetically determined pattern of organization is emerging that becomes more specific as the information is passed through the glomeruli and mitral/tufted cells of the olfactory bulb.104,112,113 How that electrical information is coded for the thousands of odorants that can be recognized and discriminated is just beginning to be understood. In the mid–20th century, Adrian114–119 suggested several mechanisms on the basis of his electrophysiologic recordings. Taken together, all of these mechanisms could produce different activity patterns across the mucosa, which would explain the different activity patterns he observed from anterior and posterior locations in the bulb. One mechanism is the odorant-selective sensitivity of the individual receptors, such that incoming odorants would excite different patterns of receptors along the mucosal surface. A second mechanism extends this concept, suggesting that receptors of like sensitivity are aggregated into particular regions of the mucosa, thus giving a different spatial representation for each odorant. The final mechanism Adrian suggests proposes that each odorant, having different physiochemical properties such as solubility, would spread differentially both in time and space across the mucosal sheet. Adrian’s proposals have been supported by later investigators.
Over the years, evidence for an olfactory odor map has been growing. Besides the electrophysiologic evidence described previously, anatomic evidence comes from special patterns identified through horseradish peroxidase tracing of receptor neurons to the bulb120 and the discovery of differential cell adhesion molecules specific to olfactory axon pathways leading to the olfactory bulb.121,122 However, genetic advances have helped solidify the argument for odor maps. It is now known that the olfactory epithelium of the mouse is roughly divided into four zones. Each zone contains a group of different olfactory receptor subtypes that seem to be confined within the designated zone. These zones are then reproduced by receptor projections to the olfactory bulb. What is more amazing is that the axons from each identical olfactory receptor subtype converge and synapse with mitral cells within only a few glomeruli of each olfactory bulb. Therefore, a specific odorant may activate certain olfactory receptor types that then send signals to specific glomeruli and create a pattern of activity much like patterns of other sensory systems in the brain.123–125 Inability to perceive particular odorants (specific anosmia) has been associated with loss of specific odor receptor genes, yielding clinical evidence for receptor specificity of odorants.113,126,127
The contribution made by the central nervous system to olfactory coding and discrimination is unclear. It may be that the olfactory code is complete by the time it leaves the olfactory bulb. Alternatively, the code may need to be completed by additional central neural processing. Whatever the status of the olfactory code, it is clear that the central nervous system uses olfactory information for many purposes. One such purpose, for instance, is in the area of feeding. Glucose-sensitive cells in the lateral hypothalamus of the monkey integrate many chemosensory inputs from both endogenous and exogenous sources, whereas glucose-insensitive cells from the same area distinguish among fewer, more specific chemosensory cues to control food acquisition behavior.128 In another area, the alpha 2 power on electroencephalogram (EEG) recording is increased after an unpleasant smell exposure (valeric acid) but not after a pleasant exposure (phenylethyl alcohol).129
Where the central nervous system processes and stores the olfactory information is also unclear. Studies on laterality have suggested that humans have a better side for olfactory ability.14,130 Zatorre and Jones-Gotman131 have suggested that the right side is the better one on the basis of their observation of no deficits in olfactory identification ability in patients after left central, parietal, and posterior brain excisions. This suggestion is supported by the finding that patients with right parietal and frontotemporal lesions have difficulty with the lateralization of odorants.132 Brand and Jacquot,133 who looked at electrodermal activity responses, showed a predominance of the right hemisphere in treatment of olfactory information that did not depend on quality of the odor and with no difference in the side of the nostril involved. Alternatively, Hong and coworkers18 have correlated olfactory function with nasal anatomy by use of CT scanning. They learned that most people are using only their left side when perceiving olfactory information. Handedness may be a determinant of this trend,134 or the preferred side may be related to learning. Both PET scanning and functional magnetic resonance imaging (MRI) have been used to look at brain responses to unilaterally and bilaterally presented odors. Many control measures must be instituted with these studies, because most odorants are not purely olfactory, and the introduction of trigeminal stimulation adds activation through contralateral inputs. In addition, the act of sniffing alone without an odorant present causes activation in certain higher brain centers, and the memory of an odorant activates others. It is not surprising that results have been inhomogeneous, but there tends to be more evidence for a right hemisphere dominance in olfactory functioning.135,136
Olfactory Cognition
Odors are understood largely on the basis of experience, and each person develops his or her own hedonic code within cultural restraints.137–139 Odor associations, once established, are notoriously difficult to erase from memory,83,140,141 even though the incident that formed the association is forgotten or seems irrational. In one study, odor memory was shown to last at least 1 year, whereas visual memory lasted only a few months.83 Interestingly, odor memory is facilitated by bilateral nasal stimulation, suggesting that people who have one-sided nasal obstruction may form poorer odor memories.142 An odor aversion can be formed to a perfectly good food by overindulgence. Similarly, becoming ill for another reason while eating an otherwise innocuous food can have the same effect.83 This same phenomenon is used effectively in animal training, in which lithium chloride, an emetic, is given with food to induce an aversion. For many of these reasons, the memory system that exists for odors may be distinct from other memory systems.142,143 The exciting molecular advances made in understanding olfactory transduction and coding suggest that learning and memory might also be a “hard-wired” system. The adaptive ability to meet specific biologic needs, like those described previously, require that no matter how the system is wired, it needs flexibility.144
Whether newborn human babies discriminate between pleasant and unpleasant odors or whether the sense of smell enters into their enjoyment of food is debatable.83 They can, however, identify odorants that have biologic meaning. In one study, 30 women who had just given birth underwent washing of one breast, and their newborns were placed prone between their breasts; 22 of the 30 newborns selected the unwashed (odorous) breast.145 Macfarlane146 has shown that, by the age of 6 to 10 days, infants prefer a pad from their own mother’s breast to one from a strange mother. Other studies have shown that between the ages of 3 and 5 years, odor perception plays a role in a child’s attachment to his or her mother.93 Between the ages of 2 and 7 years, children start to show odor preferences, which are similar to those of the adults living in the same area.147–149 Nevertheless, the olfactory sensitivity of prepubescent children to specific odorants can be quite different from that of teenagers and adults.150 All these observations of early human olfactory development have parallels in other mammals. Several researchers have suggested that this development is related to the marked growth that occurs in the neural circuitry of the olfactory epithelium and olfactory bulb from birth until adult size is reached.151,152
Pheromones, which are pervasive throughout the subhuman animal kingdom, are chemicals released by one member of a species and received by another member, resulting in a specific action or developmental process.153 Thus, the male hamster knows whether the female is receptive to mating,154,155 and the ant knows the trail laid by a conspecific to a particular food.156 (A more complete listing of messages that are probably conveyed among mammals by means of olfaction is given in Box 41-1.157,158). The search for a human pheromone has been going on for many years, and various biologic odorants refined from human urine, axillary secretions, and vaginal secretions have been touted, often in the popular press, as being pheromones. Both anatomic and behavioral studies support the possibility of human communication through odorants (see earlier discussion of the vomeronasal organ); however, additional studies with double-blind techniques are needed before adequate conclusions can be made.13,159–161 One example of the type of human biologic activity on which chemosensory control can be exerted is menstrual cycle synchrony. Russell and associates162 placed a mixture of alcohol and underarm secretion from one woman on the upper-lip skin of five women subjects and alcohol only on the skin of six control women subjects. Over a period of 5 months, there was a statistically significant (0.01) greater tendency for menstrual synchrony with the woman who donated the axillary secretions among the experimental group than in the control group. Similarly, Preti and colleagues163 applied male axillary extract on the upper lips of a group of women and observed changes in luteinizing hormone pulses. In addition, responses in these women to a mood scale indicated an overall reduction in tension and an increase in relaxation. These studies do not discriminate between activation of the olfactory and vomeronasal systems but argue for a chemosensory communication system that seems to occur in humans.
Box 41-1 Types of Messages Conveyed by Mammals by Means of Olfaction
From Doty RI: Odor guided behavior in mammals. Experientia. 1986;42:257; and Mykytowycz R. The role of skin glands in mammalian communication. In: Johnston JW Jr. Moulton DG, Turk A, eds. Advances in Chemoreception, Vol 1: Communication by Chemical Signals. New York: Appleton-Century-Crofts, 1970.
Liberles and Buck164 have discovered a second family of odorant receptors that occur in the olfactory epithelium of mice and humans. These trace amine–associated receptors (TAARs) also communicate through G proteins, but are located in olfactory neurons that lack odorant receptors. The receptors detect various amines that are found in mouse urine and are important in pheromone related activity. This would provide an explanation for pheromone-like activity in humans even with the lack of a centrally connected vomeronasal organ.
Stimulation and Measurement of Olfaction
The measurement of the detection threshold attempts to quantify the most dilute concentration of a particular odorant that an individual can detect. The general format of this test is to use a series of bottles containing a range of concentrations in predetermined steps. Although pyridine and n-butyl alcohol (1-butanol) are two of the most widely used test chemicals because of their water solubility, easy identifiability, and history of successful use, phenylethyl alcohol, which has a roselike smell, may be a better choice, because it has less trigeminal reactivity.165 The odorants are presented from lowest to highest concentration until the subject correctly identifies four odorants at a given concentration. This order of presentation avoids the adaptation (i.e., the loss of sensitivity from mere stimulation) that might occur if strong concentrations were to be used first.166 In the test situation, the subject is presented with two bottles, one containing the odorant and the other a blank. The subject is asked to choose the bottle that contains the odorant (two-alternative, forced-choice procedure).167,168 Discretion should be used, however, in the interpretation of olfactory detection threshold scores, because the test-retest reliability of this test has been reported to be low.169,170
Identification tests allow the subject to smell a number of odorants and name them correctly. The test is a suprathreshold test; that is, the stimuli are presented at a concentration above threshold that the subject considers normal for that odorant. In addition, identification tests presume normal cognitive ability. Without this ability, a low olfactory test score may be due to poor testing in someone with normal olfactory ability. Several versions of this test are available and commonly used. Cain and associates171 have developed an identification test that is administered along with a threshold test. In this test, eight common household items (e.g., baby powder, coffee, Ivory soap) are presented to the subject in screw-top jars. The subject is graded on how many of the odorants he or she can identify correctly.
Doty and colleagues172,173 have developed a method of testing identification ability using scratch-and-sniff booklets containing 40 microencapsulated odorants. This commercially available test is self-administered and can be either mailed to test subjects or used during the examination. Material accompanying the booklets allows percentile score ranking of test results by age and gender, which are known determinants of olfactory ability (see “Factors Affecting Olfactory Testing”). Because the subject is asked to choose the correct answer from a list of four possible answers, a chance performance would be 25% correct. Obviously, anyone scoring much less than this should be considered for malingering. The portability of the booklets, the freshness of the stimulus, and the fun of taking the test contribute to its popularity.
A simple olfactory screening test has been described that is available to all clinicians and is based on the ability to detect the odor of an opened alcohol pad. The patient is told to close the eyes, and the open pad is slowly brought closer to the nose. The patient notifies the test giver when an odor is detected, and the measured distance between the alcohol pad and the nose correlates with the extent of olfactory impairment.174 The test has been able to differentiate hyposmic and anosmic patients and can be modified for unilateral testing.
In other parts of the world olfactory testing also is being performed, sometimes with one of the tests noted previously and sometimes with locally designed tests. In Japan, the standard test is the T & T olfactometer, which is a rack containing eight concentrations of five different odorants. From this test, both detection and recognition thresholds can be determined, and they are charted on a graph similar to an audiogram.175 In Germany, an odorant identification forced-choice test that uses odorant-impregnated felt-tip pens has been developed (Sniffin’ Sticks, Burghart Modizintechnik, Erlangen, Germany). It has the advantage of a long shelf-life, the ability to be reused, and short test administration times.176
It is well known that odorants reach the nasal olfactory receptors from the front through the nostrils (orthonasal stimulation) and from the back through the posterior choanae (retronasal stimulation). Pierce and Halpern,177 using small film canister lids containing odorants that were placed on subjects’ tongues, were able to test these two stimulation pathways separately and show that they are independent. Heilmann and coworkers178 have developed a clinical testing kit to assess retronasal olfactory function with oral stimulus presentation. Testing of these two different routes has shown differential neural recruitment in the brain,179 which may explain differences in flavor perception with route of odor presentation. With this new knowledge, an improved clinical test of human olfaction will need to ensure whether it is testing orthonasal olfaction, retronasal olfaction, or both.
Doty and colleagues180 reviewed nine different olfactory tests, including tests of odor identification, discrimination, detection, memory, and suprathreshold intensity and pleasantness perception.181 Using a principal components analysis, they were able to determine that most of these tests measure a common source of variance. This result is borne out in clinical practice, in which it is rare to find a major discrepancy between olfactory tests.
For all these olfactory tests, especially those measuring threshold, control of stimulus concentration is obviously important. In general, the two main techniques to control or vary odorant concentration are (1) dilution of the liquid-phase odorant in varying amounts of solvent and (2) dilution of the vapor-phase odorant with air. Racks of sniff bottles can be designed with a gradient of concentrations. Although they are conveniently portable, the liquids can become contaminated by oxidation, or the odorant concentration can be changed by oxidation or evaporation.181 Therefore, the solutions must be changed frequently when open-bottle tests are used. More precise control over the stimulus intensity has been achieved with a variety of olfactometers. With mixing of pure air and odorized air streams, the odorant concentrations reaching the nose can be precisely controlled. Although these olfactometers are quite accurate in the control of the stimulus, their cost and size have restricted their use to research laboratories.
In an attempt to circumvent the problems of the air stream–delivered stimulus, some Japanese researchers have included intravenous smell testing in their olfactory evaluation.182 In such a test, the subject is asked to perceive the mercaptan (garlic) smell of injected Alinamin, a thiol-type derivative of vitamin B1. The time between the injection and the recognition of the smell is designated as the latent time, and the time between the recognition and the disappearance of the smell as the duration time. The researchers report that the latent time is influenced by olfactory acuity and that the duration time depends on adaptation to the odorant. Central olfactory disorders are suspected in patients with decreased duration times, and nonresponders to this test are said to have a poor prognosis for olfactory recovery. Although it is tantalizing to speculate that this intravenous test directly stimulates the olfactory receptor cells through the blood stream, studies by Okabe183 indicate that the mechanism of the test by the discharge of Alinamin’s metabolic by-products from the blood stream into the pulmonary alveoli, from which it reaches the olfactory receptors via the nasopharynx through the exhaled air stream.
A problem often encountered in testing of olfactory sensitivity is that many patients confuse the loss of the sense of smell with the loss of the sense of taste. Westerman184 has developed a simple test for such an evaluation, whereby the odorant is placed on the tongue and the subject is asked to describe the flavor of the material. This test also may be used to identify malingerers, because few individuals know that flavor is largely mediated through the sense of olfaction. When asked, therefore, to identify the taste of the coffee placed on the tongue, the blindfolded olfactory malingerer, who should report a bitter taste, identifies its taste as coffee but disclaims any ability to identify the coffee odor when it is held in front of the nose. Further development of these taste-odor tests by Laing and colleagues185 in Australia show that humans have a limited capacity to identify the components of taste-odor mixtures.
Electrophysiologic testing of olfactory ability in humans has been developed and is available in laboratories. Accurate delivery of the odorant molecules to the nose in warmed and humidified air is mandatory in all of these objective studies.186,187 The most peripheral of these tests, the electro-olfactogram (EOG), is obtained by placement of an electrode directly on the olfactory epithelium.188 When an odorant stimulates the receptor cells, a slow negative shift in voltage is seen. This has been shown in multiple animals, including humans, and is thought to represent a summation of the many generator potentials from single-receptor cells.26,53,189,190 Although the olfactory epithelium is relatively inaccessible in the human, some researchers, including Furukawa and associates,191 have succeeded in recording these electro-olfactogram potentials and have shown decreased potentials in hyposmic subjects that are commensurate with their olfactory loss. As these researchers have said, the electro-olfactogram provides the only objective method currently available for the differential diagnosis of anosmia caused by disorders of the olfactory epithelium versus the central olfactory tract.
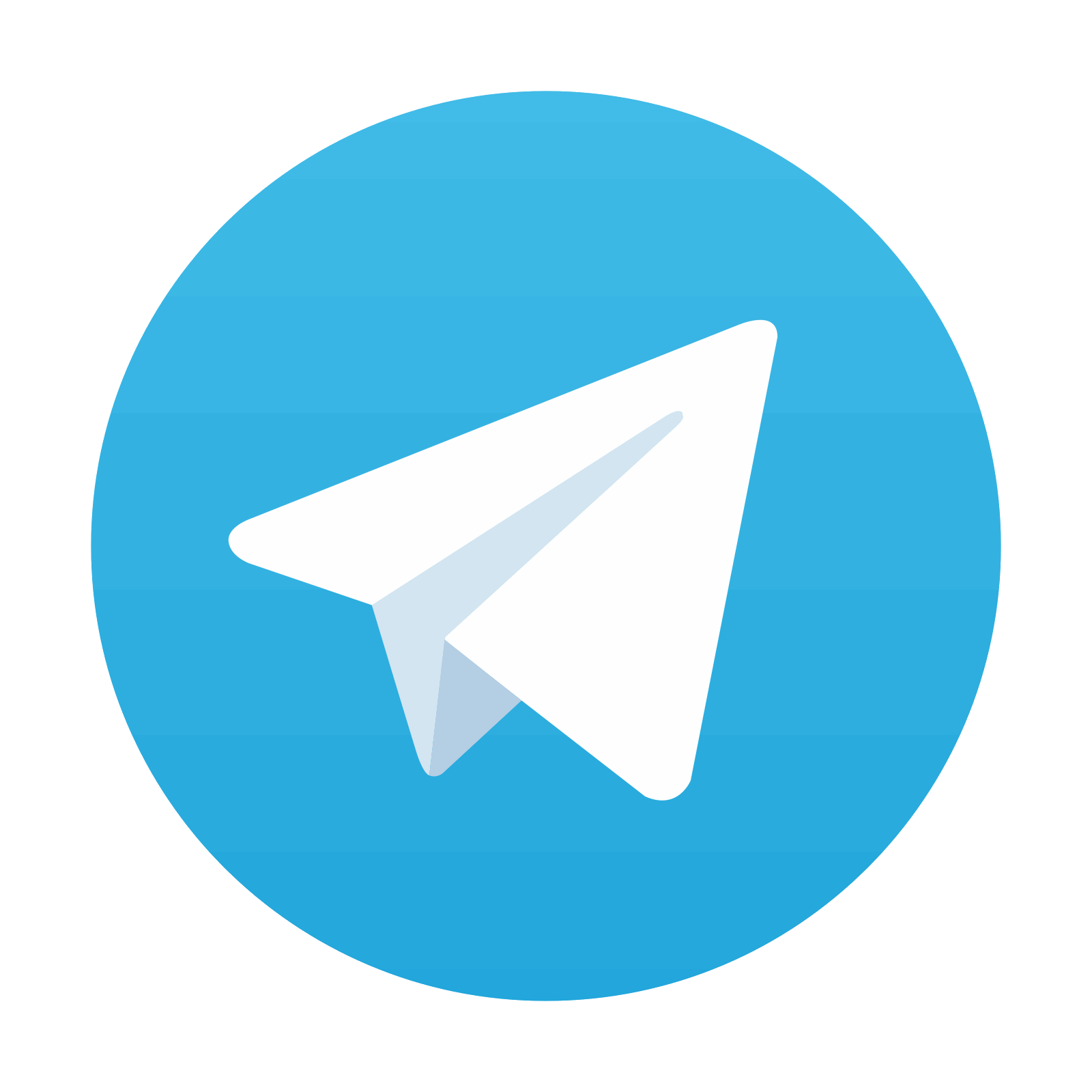
Stay updated, free articles. Join our Telegram channel

Full access? Get Clinical Tree
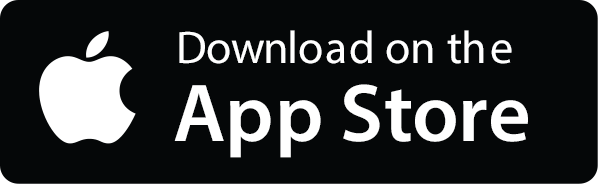
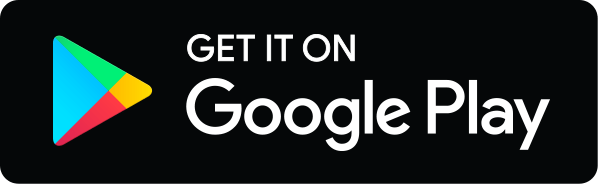