Pathogenesis of Herpes Simplex Ocular Disease
Richard D. Dix
Ocular infections caused by herpes simplex virus type 1 (HSV-1) are a common cause of vision loss and blindness worldwide.1 There are approximately 8.4 to 13.2 new HSV-1 ocular infections per 100,000 person-years in developed countries, and an overall incidence (including recurrences) of 20.7 to 31.5 episodes per 100,000 personyears.2 Although a majority of HSV-1 ocular infections manifest as corneal disease, HSV-1 can also invade the retina of an immunologically normal person to cause a sight-threatening retinitis, an event associated with some cases of acute retinal necrosis (ARN).3 A comprehensive understanding of the pathogenic mechanisms that operate during the evolution of HSV-1 ocular disease is dependent on a fundamental understanding of several concepts related to virus structure, replication scheme, and latency and reactivation.
TAXONOMY, CLASSIFICATION, AND STRUCTURE
Classification
Members of the family Herpesviridae are relatively large, enveloped, DNA-containing viruses.4 Eight human herpesviruses have been recognized to date and share the clinically important feature of producing a life-long latent infection in the host that is potentially recurrent. Of the eight human herpesviruses, HSV-1, HSV type 2 (HSV-2), and varicella-zoster virus (VZV) exhibit a remarkable affinity for nervous system tissues. Together, they are classified as alphaherpesviruses,4 a subfamily of Herpesviridae that exhibit a variable host range, relatively short replication cycle, rapid spread and efficient destruction of cells grown in culture, and the capacity to establish latent infections primarily but not exclusively in sensory ganglia.
Virion Structure
HSV-1 is a spherical particle with an average diameter of 186 nm that extends to 225 nm when including the spikes that protrude from the envelope.5 Key structural components of the mature infectious virus particle (virion) include an electron-dense core consisting of the viral genome in the form of a double-stranded DNA, a protein shell or nucleocapsid, an amorphous tegument that surrounds the nucleocapsid, and the envelope.6 The genomic DNA of HSV-1 consists of about 150,000 base pairs with a high G + C content of 68%.6 The DNA is contained in the virion core in the form of a toroid or spool that is in a liquid crystalline state. The DNA-containing toroid is suspended by fibrils embedded in the underside of the nucleocapsid, a 100-nm structure that exhibits icosahedral (20-sided) symmetry through the precise arrangement of 162 hollow capsomers (12 hexagonal and 150 pentagonal). The nucleocapsid serves to protect the genome and consists of a number of immunogenic virus-encoded structural proteins. The tegument, the space between the surface of the nucleocapsid and the undersurface of the envelope, has no distinctive features other than exhibiting an amorphous fibrous appearance that shows variable thickness. The tegument is composed of a least 20 different virus-encoded proteins that are immunogenic and function as transactivators in several early events of virus replication.6 Surrounding the tegument and nucleocapsid is the envelope that is obtained from an extensively modified inner nuclear membrane and nuclear lamina of the host cell during the maturation process. These modifications include the insertion of at least 11 different virus-encoded glycoproteins (gps), some of which serve as receptors to mediate entry of the virus into the host, cell-to-cell spread of virus infection, and stimulation of host immune responses including neutralizing antibodies.6 An intact envelope is an absolute requirement for HSV-1 infectivity.
Genome Structure
The genome of HSV-1 possesses several unique structural characteristics. It consists of two covalently linked structural components, designated L (long) and S (short). Each component consists of unique sequences bracketed by inverted repeats that allows for rapid circularization of the DNA molecule when released from the capsid into the nucleus of the infected host cell.6 Circularization appears to be necessary for DNA replication and may be necessary for the establishment of latency. The L and S components can invert relative to one another to yield four equimolar linear isomers of which all are functional in the infected cell. The genome encodes for approximately 90 unique transcriptional units6 of which at least 84 encode for structural and nonstructural proteins, the latter being regulatory proteins and enzymes. Several of these unique transcriptional units are antisense to each other. The complete genome sequences for an increasing number of strains of HSV-1 have been determined. These include the genome sequences of HSV-1 strains 17, KOS, F, McKrae, and H1297,8,9,10,11 as well as a comparison of the genome sequences of KOS and F with those of six ocular HSV-1 clinical isolates.12
Restriction endonuclease analysis of the HSV-1 genome has demonstrated intratypic variation and therefore the existence of numerous strains of HSV-1 of which no two epidemiologically unrelated clinical isolates are identical. The restriction endonuclease cleavage patterns of an individual HSV-1 strain are relatively stable as demonstrated by no changes noted in isolates collected from the same person over a period of 13 years.6 The “fingerprinting” of HSV-1 clinical isolates by restriction endonuclease cleavage patterns has also proven helpful in tracing the transmission of individual virus strains from one person to the next and in identifying multiple isolates from a single patient. Animal studies suggest that some HSV-1 strains selectively cause epithelial disease, stromal disease, or uveitis following ocular inoculation of mice.13,14,15 HSV-1 strains also differ with respect to neurovirulence and neuroinvasiveness as measured by their ability to cause experimental encephalitis in mice.16,17 Experimental neurovirulence of HSV-1 has been correlated with the expression of a subset of virus genes18,19 that include the γ34.5 gene of the virus.20,21,22 Thus, the HSV-1 genome appears to be a major determinant of viral virulence.
Epidemiology
HSV-1 is distributed worldwide1 and has been reported in developed and primitive societies including tribesmen of remote Brazilian villages.23 Because animal vectors for human HSV-1 infection have not been recognized, humans remain the only reservoir for transmission of the virus to other humans. Seroepidemiologic studies indicate an incidence of infection that generally increases with age and approaches 80% by age 60 years.6 This fact, coupled with the observations that HSV-1 infection is rarely fatal and that HSV-1 establishes a latent infection in the host, has led to the conclusion that nearly one-third of the world’s population suffers from some form of recurrent HSV-1 infection.24
MICROBIAL AND CELLULAR PATHOGENESIS, INCLUDING IMMUNOLOGY
Productive (Lytic) Infection
Like all viruses, HSV-1 is an obligate, intracellular parasite and therefore requires a healthy host mammalian cell to produce new progeny viruses through a productive (lytic) replication mechanism. Attachment of the virion to the host cell takes place initially through binding of virion envelope glycoproteins gB and gC to cell-surface glycosaminoglycans,25,26 followed immediately thereafter by the interaction of the virion envelope glycoprotein gD with additional cell-surface receptors that include nectins, herpes virus entry mediator (HVEM), and 3-O-sulfated heparan sulfate.27,28,29 Fusion of the virion envelope with the host-cell plasma membrane (adsorption) is mediated by gD in collaboration with gB, gH, and gL.30 Following attachment and adsorption, the nonenveloped nucleocapsid is transported via the microtubule network31 to the nuclear pores where the viral DNA is released into the nucleus. A tegument protein of the parental virus also rapidly inhibits host protein synthesis in preparation for efficient translation of virus-specific transcripts by host ribosomes.32
After viral DNA enters the nucleus, a well-coordinated cascade of events takes place that leads to the expression of more than 80 HSV-1 genes.6 Briefly, viral DNA is transcribed in the nucleus into mRNA molecules using host RNA polymerase II in participation with several viral factors. The virus-specific mRNAs are then transported to the cytoplasm where they bind to host ribosomes to assemble amino acids into virus-specific proteins. It is important to recognize, however, that HSV-1 gene expression is tightly regulated and actually occurs as a cascade in three distinct waves of transcription to yield three distinct classes of viral proteins designated immediate-early (alpha), early (beta), and late (gamma).33 Transcription of six immediate-early genes is stimulated by a tegument protein known as VP16 (alpha-transinducing factor) of the parental virus that requires an existing cellular transcription factor to function, but does so in the absence of de novo virus-specific protein synthesis.34 The synthesis of immediate-early proteins reaches peak rates at approximately 2 to 4 hours postinfection. Immediate-early proteins have regulatory functions and are required for the synthesis of the second group of viral proteins, the early proteins. This group of proteins reaches peak rates of synthesis at about 5 to 7 hours postinfection and includes many enzymes involved in viral DNA replication such as thymidine kinase and DNA polymerase. Importantly, the early proteins serve to downregulate the synthesis of immediate-early proteins and ultimately to induce the synthesis of the late proteins. Structural proteins such as the capsid proteins and envelope glycoproteins are included among the late proteins that also serve to downregulate the synthesis of the early proteins. Late proteins destined to become envelope glycoproteins acquire their carbohydrate sidechains by using the glycosylation machinery of the host cell. Completed glycoproteins are inserted into all host-cell membranes, including the plasma membrane, where they serve as important targets for immune surveillance.
Viral DNA replication takes place in the nucleus following early gene expression that supplies many of the enzymes needed for DNA synthesis. The HSV-1 genome contains three origins of DNA replication that leads to DNA synthesis by a rolling circle mechanism, yielding concatamers that are cleaved into monomers and ultimately packaged into capsids.35 Why three origins of replication exist on the HSV-1 genome remains unclear. Only a small portion of the total input viral DNA from parental virions is actually replicated.
Assembly of progeny virus particles occurs in several distinct stages.6 Following the packaging of newly synthesized DNA into preassembled capsids, the virus matures and acquires infectivity by budding through the inner lamella of the cellular nuclear membrane that has been modified by the insertion of several different virus-induced glycoproteins. The mechanism by which the mature virus particle exits the host cell remains a topic of debate. The favored hypothesis includes transport of the virus from the nucleus to the cell surface via the endoplasmic reticulum and Golgi apparatus. In fully permissive tissue culture cells, the entire productive (lytic) replication of HSV-1 takes approximately 18 to 20 hours.
Cells that are productively infected with HSV-1 do not survive. Productively infected cells undergo major structural and biochemical alterations almost at the outset of the reproductive cycle. These profound alterations ultimately result in the death and destruction of the host cell, a process known as virus-induced cytopathology.
Establishment and Maintenance of Latent Infection
HSV-1 is transmitted to mucosal surfaces by close personal contact. The most common site of primary HSV-1 infection is in the facial area served by the maxillary division of the trigeminal nerve. Although primary virus infection is often asymptomatic, infection of this area leads to the establishment of a latent infection in the maxillary division of the trigeminal ganglion. Following a round of productive infection in epithelial cells, the virus enters the nerve endings of the local sensory and autonomic nerves innervating the area of infection. Virus replication in epithelial cells may not be an absolute requirement, however, because replication-defective HSV-1 mutants can also infect neurons and establish a latent infection in experimental animals.36 It is thought that the virus loses its envelope on entry into the neuron, and the nucleocapsid is transported by microtubule-dependent retrograde axonal flow to the neuronal cell body at a rate of 5 to 10 mm per hour.6 Because only 10% of the cells in a typical sensory ganglion are neurons, it is thought that some of the remaining 90% of cells that consist of nonneuronal satellite cells and Schwann cells are infected during initial infection, and these nonneuronal cells may be a major source for infectious virus isolated from sensory ganglia during primary infection.37 Primary HSV-1 infection is normally restricted to the trigeminal ganglion where ganglion infection usually resolves within 7 to 14 days after primary virus infection. Virus particles are ultimately cleared, and a latent infection is established in neurons. Molecular techniques used to provide a comprehensive quantification of HSV-1 latency within the mouse trigeminal ganglion at the single-cell level have revealed that as few as 1% to as many as 50% of neurons in a ganglion may harbor latent virus.38 Assuming approximately 20,000 neurons per ganglion, latent HSV-1 genomes may therefore be carried in less than 200 neurons or more than 10,000 neurons in the mouse trigeminal ganglion.37 Many infected neurons may also contain multiple copies of latent HSV-1 genomes, ranging from fewer than 10 genome copies per neuron to more than 1,000 genome copies.38 That a single neuron might harbor latent genomes from multiple virus strains is possible in theory. More recent work has suggested that HSV-1 preferentially establishes latency in a specific subset of sensory ganglion neurons that bear the A5 marker.39,40
The molecular events that take place during the establishment and maintenance of HSV-1 latency in neurons are complex and not completely understood. Because neurons that harbor HSV-1 in a latent state are not necessarily killed by virus-induced cytopathology associated with a productive infection, a latent infection must therefore represent a virus-cell interaction in which genes associated with lytic infection are shut off. In fact, HSV-1 latency is characterized by the presence of the entire virus genome in a circular episomal form associated with the nucleosome,41 but in the absence of detectable physical virus particles or even detectable virus-specific proteins. Thus, latency does not represent a persistent, low-level form of productive infection. For many years, these fundamental observations constituted an operational definition of HSV-1 latency; that is, infectious virus cannot be recovered from latently infected ganglia following homogenization. However, explantation or cocultivation of ganglionic tissues that harbor latent virus will usually result in in vitro reactivation and ultimate recovery of infectious virus within 10 to 45 days of culture.42
Although neurons latently infected with HSV-1 are thought not to contain detectable virus-specific proteins, they do contain detectable virus-specific mRNA molecules localized in the nucleus that have been termed latency-associated transcript (LAT).43,44,45,46 The discovery of LAT more than two decades ago has expanded (and often confused) our understanding of neuronal HSV-1 latency, and the detection of LAT alone now serves as a molecular marker for HSV-1 latency in neurons. LAT maps to the long terminal repeat regions of the HSV-1 genome and is antisense to ICP0, an important regulatory immediate-early gene of the virus. The primary 8.3-kilobase (kb) primary LAT transcript is unstable and is spliced, yielding an abundant 2-kb LAT that is a stable intron.47 Interestingly, the 2-kb LAT intron is also detected during productive HSV-1 infection.
Because LAT is transcribed during latent infections, its role in the establishment, maintenance, and/or reactivation from latency continues to be an area of intense investigation. For this reason, the literature on LAT is extensive and does not lend itself to a comprehensive review. In brief, however, a common theme of LAT studies has been that LAT probably serves to protect neurons from death48 by protecting neurons against apoptosis (one of several programmed cell death pathways),49,50,51 by reducing virus gene expression via a microRNA (miRNA) effect on ICP0 protein expression or small interfering RNA (siRNA) silencing of lytic genes at the chromatin level,6 or by other mechanisms not yet identified.
Transcripts to all virus genes (immediate-early, early, late, as well as LAT) are detected in trigeminal ganglia of infected animals during the first 24 to 72 hours after primary infection.52 Control of early virus replication in ganglia is thought to involve several host immune response effectors that include interferon-gamma and CD8+ T cells. CD8+ T cells infiltrate the ganglia beginning at about 5 to 7 days after infection, coincidentally at the time when virus replication is observed to decrease prior to the establishment of latency.53,54 Maintenance of HSV-1 latency within sensory neurons has also been associated with interferon-gamma55,56 and HSV-1-specific CD8+ T cells57 that include HSV-1-specific memory CD8+ T cells.58,59 Recent work by Hendricks and coworkers has suggested that HSV-1-specific CD8+ T cells that accumulate within trigeminal ganglia during latency recognize a strongly immunodominant epitope on glycoprotein B (gB498) as well as a subdominant epitope on the ribonucleotide reductase (RR1822) of the virus.60,61 Priming of HSV-1-specific CD8+ T cells appears to depend on early CD4+ T-cell help,62 although CD4+ T cells do not appear to have a direct involvement in the maintenance of HSV-1 latency. Because interferon-gamma can block HSV-1 reactivation in some, but not all, latently infected sensory ganglia,55,56 another nonlethal mechanism has been postulated whereby HSV-1-specific CD8+ T cells directly maintain neuronal latency. This novel nonlethal mechanism63 proposes that the typically lytic granule component of CD8+ T cells, granzyme B, instead blocks HSV-1 replication and thereby maintains virus latency within sensory neurons by directly cleaving ICP4, a virus-encoded protein required for efficient transcription of early and late virus genes.
Reactivation from Latent Infection
Reactivation of HSV-1 at the ganglionic level to produce recurrent clinical disease in the human host usually occurs in response to well-defined stimuli that include immunosuppression, hormonal changes, neurectomy, nerve damage, and ultraviolet light exposure. Similarly, reactivation of HSV-1 in experimental animals has been induced by physical trauma to the animal, high temperature, ultraviolet radiation, neurectomy, or iontophoresis of adrenaline into the eye. General stress has also been shown to induce reactivation in mice, a process that seems to involve a reduction in the number and functionality of HSV-1-specific CD8+ T cells.64,65 Taken together, reactivation of HSV-1 from latent infection appears to be triggered by signals that alter the physiologic status of the neuron that harbors latent virus, leading to activation of neuronal signaling pathways, subsequent activation of host-cell transcriptional factors or protein kinases,66 and ultimately initiation of productive virus replication.
Two long-standing hypotheses have been put forth to explain the initiation of the lytic cycle during reactivation from latency. The first hypothesis proposes that the HSV-1 immediate-early gene ICP0 initiates reactivation from latency by serving as a nonspecific transactivator capable of inducing expression of all classes of HSV-1 genes.67,68 The second hypothesis postulates that HSV-1 early gene expression followed by virus DNA replication precedes and is required for efficient immediate-early gene expression during reactivation from latency.69,70 Both hypotheses, however, were formulated using explant reactivation models, and reexamination of these hypotheses using more recent in vivo reactivation approaches71 have suggested that reactivation from latency requires neither prerequisite virus DNA replication72 nor functional ICP0.73 More recent work has therefore suggested a third novel hypothesis to explain HSV-1 exit from latency, a hypothesis that focuses on VP16, a multifunctional virion protein that is expressed late in the virus replication cycle.6 Studies using the mouse corneal model of HSV-1 latency coupled with genetically engineered virus mutants have provided intriguing evidence for the de novo expression of VP16 in latently infected neurons, but in the absence of other virus proteins following stress.74 Moreover, de novo expression of VP16 in latently infected neurons appears to promote entry of virus into the lytic program, thereby releasing the virus from latency.74,75,76
The fate of neurons after virus reactivation is unclear and remains a controversial area of debate.6 One school of thought believes that neurons survive after the reactivation event because patients with frequent recurrences at the same site fail to develop local anesthesia at that site. Arguing against this belief is the observation that nerve endings from adjacent unaffected neurons can grow into the site of recurrence during the healing process and reestablish a sensory nerve network. Probably the best argument in support of neuron survival, however, can be found in clinical studies of women with recurrent genital lesions who shed virus at times between episodes of frank vesicular lesions. Because the shed virus originates from focal microscopic lesions that arise frequently and for long durations, and each microscopic lesion must arise from a reactivated neuron, there simply would not be enough neurons to support virus reactivation over many years if the individual neurons die during the process of virus reactivation.6
Reactivation of HSV-1 from the trigeminal ganglion to cause recurrent ocular disease requires infection of the ophthalmic division of the ganglion. This may occur at the time of either primary infection or reactivation when virus spreads to the ophthalmic portion of the trigeminal ganglion and then peripherally to the ophthalmic branch of the trigeminal nerve. It is therefore possible for HSV-1 infection of the eye to occur as a consequence of primary infection at an orofacial site. Moreover, HSV-1 infection of the ophthalmic division of the trigeminal nerve may occur without primary infection of its ophthalmic branch.
Overview of Host Immune Responses
A detailed description of the complex innate and adaptive immune events of the host that orchestrate the containment of HSV-1 spread within the peripheral nervous system of the host, and eventually the clearance of acute virus infection at peripheral sites, is beyond the scope of this review. In general, however, both innate and adaptive immunity act in concert to provide an effective immune defense. Paradoxically, latent and recurrent HSV-1 infection occurs in the face of active humoral and cellular immunity.77 More specific information on host immune responses during the pathogenesis of HSV-1 ocular diseases is provided below in sections on animal models for corneal and retinal disease.
HSV-1 infection stimulates the production of antibodies that participate in virus neutralization, antibody-dependent complement-mediated cytotoxicity, and antibody-dependent cell-mediated cytotoxicity. Passive transfer studies conducted in experimentally infected mice have suggested that humoral immunity plays a role in restricting the spread of virus from peripheral sites to sites within the nervous system. Administration of either hyperimmune HSV-1 sera78,79 or monoclonal antibodies to different HSV-1-specific glycoproteins80,81,82,83 provided significant protection against acute neurologic illness and death. Although passively administered antibodies may serve to reduce the extent of ganglionic infection in HSV-1-infected mice, they fail to prevent the establishment of latency within sensory ganglia.84 Virus apparently gains entry to peripheral nerve endings very soon after primary infection and appears to become protected from immune surveillance.85
Although humoral immunity plays a role in the general immune response to HSV-1 infection, cell-mediated immunity appears to be central to the control of primary and recurrent virus disease in humans. This conclusion is based on the observation that patients with human immunodeficiency virus (HIV)-1-induced immunosuppression (acquired immunodeficiency syndrome [AIDS]) or hematologic malignancies (e.g., Hodgkin disease) or patients receiving immunosuppressive therapy for bone marrow or organ allografts develop cutaneous HSV-1 infections that are usually more severe, require longer periods of time to heal, and may disseminate to involve multiple organ systems. Thus, cell-mediated immunity appears to be involved in virus clearance from cutaneous sites, whereas antibody may function cooperatively to restrict access of virus to and from nervous system tissues. Much of our knowledge regarding cellular immunity has been derived from mouse models of experimental herpesvirus infection, primarily because a variety of inbred mouse strains and available immunologic reagents have allowed a detailed analysis of major histocompatibility complex (MHC)-restricted responses. A number of cell-mediated immune responses are stimulated during experimental HSV-1 infection of mice (see below). These include macrophages, natural killer (NK) cells, and a variety of T lymphocyte subpopulations (i.e., CD4+ and CD8+ T lymphocytes) and Th1-type cytokine responses that mediate cytotoxicity, delayed-type hypersensitivity (DTH), and helper or suppressor functions.86
Like many viruses, HSV-1 has developed effective strategies to evade both innate and adaptive host immune responses. Type 1 interferon signaling87 and dendritic cell maturation88 associated with the innate immune response are blocked by HSV-1-encoded proteins ICP0 and ICP27, respectively. Similarly, humoral immune responses of adaptive immunity are prevented by glycoprotein gC and a complex of glycoprotein gE and glycoprotein gI of the virus via blockage of complement-mediated neutralization of virions by IgM antibody89 and antibody-mediated virion neutralization without complement by binding to the Fc region of IgG molecules,90 respectively. Finally, one of the first immune evasion mechanisms identified for HSV-1 was a role for ICP47 in blocking peptide transport into the endoplasmic reticulum and loading of this peptide onto MHC class I (i.e., antigen presentation), thereby evading CD8+ T-cell effector function.91 Additional strategies used by HSV-1 to evade cellular immunity have been traced to inactivation of cytotoxic T lymphocyte function by expression of the Us3 gene product92 and inhibition of B cells to present MHC class II epitopes to CD4+ T cells by the ICP22 protein of the virus.93
ANIMAL MODELS FOR HSV-1 CORNEAL DISEASE
Pathogenesis of Clinical Disease
Most cases of HSV-1 corneal disease involve macroscopic lesions in the superficial epithelial layer of the cornea that originate from direct virus-induced cytopathology. This form of HSV-1 corneal disease tends to be self-limited and usually heals without permanent loss of vision. Its pathogenesis is relatively straightforward. Localized productive virus replication of corneal epithelial cells results in cell lysis and death that leads to release and spread of virus to adjacent cells. These foci of cytopathology merge to form dendrites, which combine eventually to form map-shaped geographic ulcers if untreated. Unfortunately, about 20% of these patients develop disease in the deeper stromal layer of the cornea.
Two distinct forms of stromal disease have been recognized.2,94,95,96 Disciform keratitis is a form of stromal disease that is characterized by corneal edema. Two theories have been advanced to explain the pathogenesis of disciform keratitis. In the first, HSV-1 is thought to infect the corneal epithelium that results in cell lysis caused by virus-induced cytopathology alone or in combination with immune mechanisms. The subsequent cell dysfunction leads to stromal edema. The second theory postulates that the stromal edema originates from a DTH reaction to HSV-1 antigens contained within the stroma or endothelium. This is consistent with the rapid clinical response of patients with disciform keratitis to topical corticosteroids. These two theories are not mutually exclusive.
The second form of stromal disease is herpes stromal keratitis (HSK), a necrotizing keratitis that leads to scarring of the cornea and progressive loss of vision with each recurrence. Importantly, HSK occurs at a time when infectious virus cannot be detected in the cornea. This observation has led to the conclusion that HSK represents an immunopathologic disease that develops because of an immune response to virus antigens that remain in corneal tissue following clearance of replicating virus97 (see below). The clinical finding that corticosteroid treatment hastens the resolution of this stromal disease in patients supports the finding that HSK is an immunopathologic disease.
A comparison of clinical isolates of HSV-1 from patients with dendritic keratitis to those recovered from patients with stromal keratitis has suggested that virus strain might influence the pattern and severity of HSV-1 corneal disease. Virus strains associated with stromal keratitis were found to release larger amounts of envelope glycoproteins into the culture media when grown in tissue culture cells.14,15 Although there is some disagreement among studies as to whether glycoprotein gD or glycoprotein gC of the virus is released preferentially, these studies support the hypothesis that HSV-1 strains responsible for stromal keratitis release more antigens into the stromal milieu, thereby causing stromal rather than epithelial disease.
Animal Model for Investigations of the Pathogenesis of Herpes Simplex Keratitis
The scarring and subsequent vision loss associated with HSK often leads to corneal transplantation that is usually performed between recurrences of active infection. The diseased corneal tissue, however, is of little use in determining the virologic and immunopathologic events that take place during the progression of HSK. For this reason, experimental animal models have been helpful in providing insights into the pathophysiology of HSV-1 corneal disease.
Much of the early literature on experimental HSK used rabbit because the animal exhibits spontaneous recurrences of virus shedding and disease following HSV-1 infection of the cornea. The rabbit eye is also large, a practical consideration that has allowed for basic histopathologic studies of primary and recurrent disease, testing of various drugs for treatment of HSK at various stages of recurrence, and basic pathogenic studies of primary and recurrent HSV-1 epithelial disease. A major disadvantage in the use of rabbits to study the pathogenesis of HSK, however, has been a relative lack of available immunologic reagents when compared with the mouse to explore the immunopathologic components of the disease. Consequently, many investigators have turned to mouse models of experimental HSK because there exists an exhaustive literature on the genetics and immunology of the mouse, as well as a wealth of available immunologic reagents for this animal. Unlike rabbits, however, mice generally fail to exhibit spontaneous shedding of virus in the tear film and recurrent corneal disease. These animals nevertheless develop a delayed onset of corneal inflammation that resembles many of the characteristics of recurrent HSK in the clinical setting. Certain mouse strains, however, are more susceptible for developing HSK (BALB/c, A/J, NIH), whereas other mouse strains tend to be more resistant to disease development (C57BL/6, C3H).98
Corneal infection of a mouse with most strains of HSV-1 is accomplished by topical application of a suspension of virus onto a cornea that has been abraded with a sterile needle prior to virus inoculation. Lesions with a characteristic dendritic morphology develop within 2 days of infection resulting from virus replication and destruction of corneal epithelial cells, but these lesions are transient and resolve by 4 days after infection. Although the duration of virus replication in the cornea varies with dose and strain of virus, replicating virus is generally cleared from the cornea within 7 to 8 days of infection. Virus clearance is due to innate immune responses predominated primarily by neutrophils that originate from blood vessels in the limbus and migrate toward the center of the cornea,99,100,101 although NK cells are also involved.2 These cells are attracted to and activated within the cornea by a number of Th1 cytokines that include interferon-gamma, IL-2, and IL-12.102 The Th2 cytokine IL-6 also plays a central role in virus clearance in part though induction of the neutrophil chemokine MIP-2 (CXCL2). More recent work has implicated a transient role for Th-17 in neutrophil infiltration.103 These (and other) innate immune responses are initiated by HSV-1 activation of toll-like receptors, especially TLR2, TLR3, and TLR9.104 The neutrophil response peaks at about 4 days following primary HSV-1 infection and declines thereafter between 6 and 8 days after infection.2 During virus replication in corneal epithelium, the virus gains access to the termini of sensory neurons, and it is transported to neuronal nuclei in the trigeminal ganglion where it establishes a latent infection after brief rounds of productive replication.
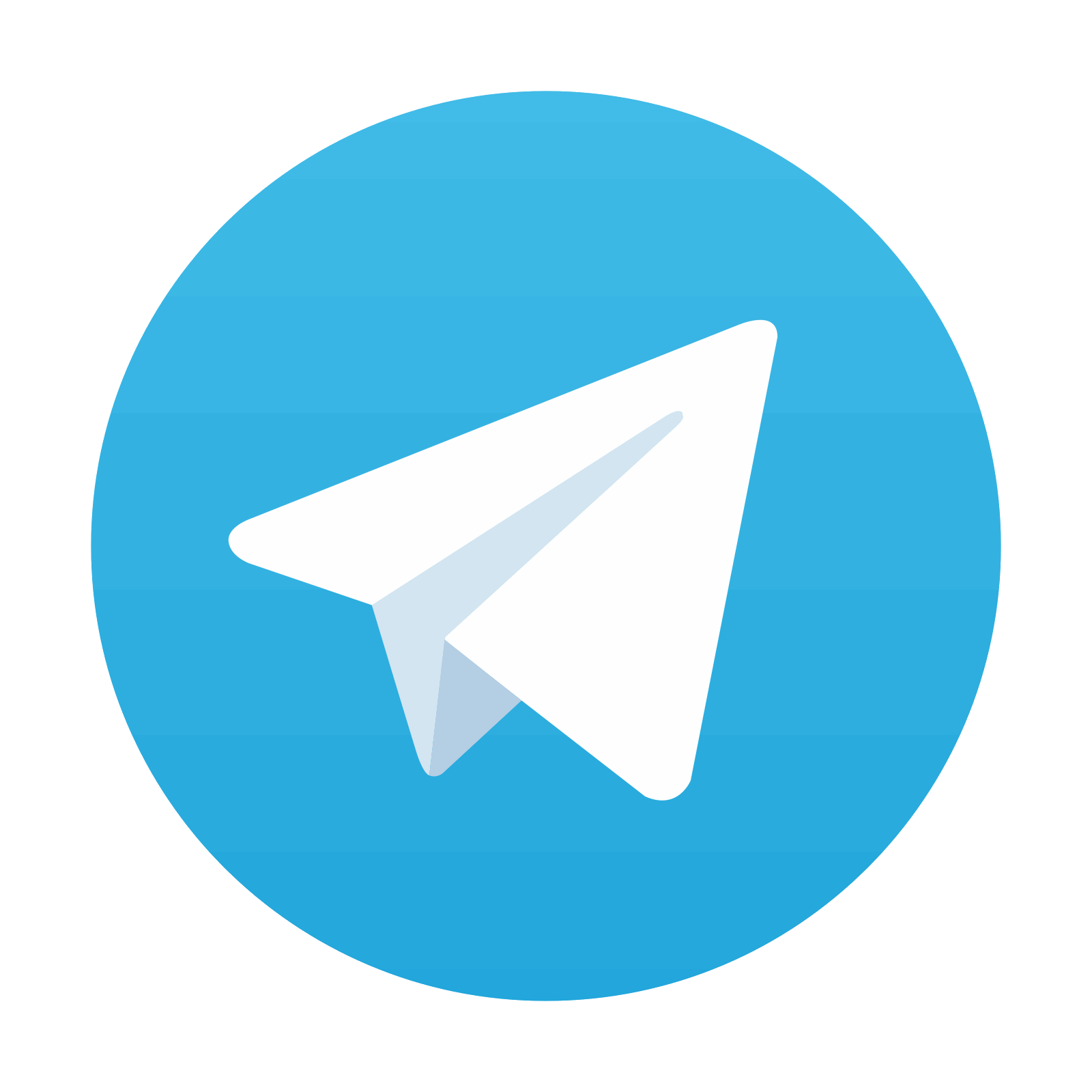
Stay updated, free articles. Join our Telegram channel

Full access? Get Clinical Tree
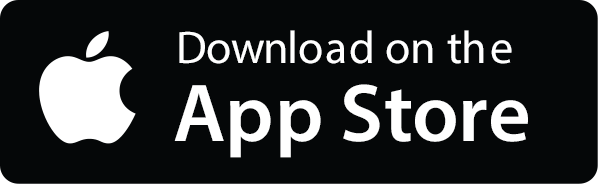
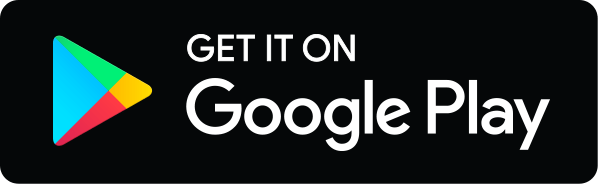