!DOCTYPE html PUBLIC “-//W3C//DTD XHTML 1.1//EN” “http://www.w3.org/TR/xhtml11/DTD/xhtml11.dtd”>
3
PATHOGENESIS AND PATHOPHYSIOLOGY OF AGE-RELATED MACULAR DEGENERATION ANA
INTRODUCTION
Age-related macular degeneration (AMD) is the leading cause of severe visual acuity loss in the United States among people older than 60 years, representing 54% of legal blindness (1). In the general population, the number of people in this group is increasing. As a result, vision loss from macular degeneration is a growing problem. Ninety percent of AMD patients have dry AMD, and 10% of AMD patients have wet AMD. Currently, an estimated 8 million Americans are affected with early AMD, and over 1 million will develop advanced AMD within the next 5 years (2).
Many changes in the macula could be due to normal aging. The focus of study has been which aging processes are implicated in the pathogenesis of senile macular degeneration or at what stage they become pathologic (3). The critical question to be answered is “Is AMD a normal process of aging or is it a disease by itself?” (4–7). Most of the authors defend the theory in which AMD is an advanced stage or perturbation of the normal process of eye senescence (3,4,8,9).
The pathophysiology of AMD is incompletely understood, but genetic tools offer new insights into the development and progress of AMD (10).
RISK FACTORS
AMD is a multifactorial disease in which multiple genetic and environmental factors are involved.
Age: Age is the largest risk factor for AMD (11–17).
Smoking: The mechanism by which smoking might affect the retina is unknown; however, there exists a direct association between the risk of developing advanced AMD with the number of cigarettes smoked (18,19).
Sunlight exposure: Individuals who wore sunglasses regularly were less likely to develop soft drusen (20). Results from the Beaver Dam Study suggest that people who spent leisure time outdoors were at an increased risk of developing early AMD (21). On the other hand, some studies have shown little or no association between sunlight exposure and the risk of AMD (22–24).
White individuals: It is postulated that increased levels of melanin could increase the free radical scavenging potential of the retinal pigment epithelium (RPE) and Bruch’s membrane (BrM), thereby protecting against the risk of advanced AMD (25–28).
Female sex: Female gender might be a risk factor in individuals older than 75 years (29), with double the risk of developing wet AMD in comparison with age-matched men. However, nonstatistically significant differences have been demonstrated (30).
Cholesterol levels, obesity, hypertension, cardiovascular disease, and increased dietary fat: These factors seem to be related to the risk of developing AMD, but different studies do not agree (31–37).
Genetics: Several studies show a genetic factor in the pathogenesis of the disease (38–41). The gene for apolipoprotein E (APOE) is the first identified susceptibility gene for AMD and has been also associated with other diseases of aging including Alzheimer’s disease. APOE epsilon 2 allele is associated with a 50% increased risk of AMD (42); however, APOE epsilon 4 allele is associated with 57% reduction in risk of wet AMD (43). Several loci have been also associated with AMD, including two major loci in the complement factor H (CFH) gene on 1q32 and the ARMS2/HTRA1 locus on the 10q26 gene cluster (44,45).
In short, genes influence many biologic pathways, but genetic susceptibility can be modified by environmental factors. Together, they are greatly predictive of onset and progression of disease (46,47).
PATHOPHYSIOLOGY AND PATHOLOGY
The pathology of AMD is characterized by degenerative changes affecting outer retina (photoreceptors), RPE, BrM, and choriocapillaris. These structures, collectively called Ruysch’s complex (4,48), provide an optimal environment for retinal function—high-resolution and color vision (cones), and peripheral vision and vision at dusk (rods).
AMD can be classified according to its onset, distinguishing early and late AMD. Early pathologic changes in AMD involve basal deposits (laminar and linear) (49) in BrM, which cannot be distinguished by clinical evaluation. Late-stage AMD shows loss of RPE, decreased choriocapillaris density, and decreased lumen diameter of the choriocapillaris. There is no neovascularization in atrophic or dry AMD. In wet AMD, neovascularization, exudative change, and disciform scar formation are observed (50).
THE RETINAL PIGMENT EPITHELIUM
The RPE is a central element in the pathogenesis of AMD. It is a postmitotic, cuboidal monolayer of pigmented cells, which improves visual resolution and neutralizes photo-oxidative stress. The RPE has a very high metabolic rate and is rich in mitochondria. It is located between the neural retina and choroid. Because of its neuroectodermal origin, the RPE is considered part of the retina. The inner boundary (apical membrane) interdigitates with the outer segments of photoreceptors. The outer boundary (basolateral membrane) faces BrM forming the outer blood–retinal barrier (BRB). Functions of the RPE include regeneration of bleached visual pigments; formation and maintenance of two extracellular matrixes, the interphotoreceptor matrix and BrM; transport of nutrients, ions, and water between photoreceptors and the choriocapillaris; and phagocytosis of membranous discs of the outer segments of photoreceptors (51). Another pivotal function of the RPE is light absorption and protection against photo-oxidation. The RPE is also involved in the immune privilege of the eye through the secretion of immunosuppressant factors (52).
TRANSPORT
Transport through the RPE is bidirectional. From the subretinal space to choroid, the RPE transports electrolytes and water. From the blood to the photoreceptors, the RPE transports glucose and other nutrients.
Transport from blood to the photoreceptors encompasses glucose and other vital nutrients. The RPE absorbs glucose, retinol, ascorbic acid, and fatty acids in the blood and delivers them to the photoreceptor. For the transport of glucose, the RPE has high amounts of glucose transporter (GLUT) in both their apical and basolateral membranes. Another notable function of the RPE is transport of retinyl to ensure its supply to the retinal photoreceptors. In this process, many complex intermediate steps take place. A critical step involves an RPE enzyme that isomerizes all-trans-retinyl esters into 11-cis-retinal, which is essential for rod and cone function (33,53).
The delivery of the fatty acids such as docosahexaenoic acid and eicosapentaenoic acid to the photoreceptor is another important RPE function (54). These omega-3 fatty acids cannot be synthesized by the nervous tissue but are an essential component of the membranes of neurons and photoreceptors. They are synthesized in the liver from the precursor, linolenic acid, and are carried in the blood by plasma lipoproteins (33). In addition, docosahexaenoic acid is the precursor of the D1 neuroprotectin that protects RPE from oxidative stress (25,55). The main sources of omega-3 fatty acid are fish products. The Blue Mountain Eye Study and some case–control studies have found evidence of reduced risk of advanced AMD and in those who eat fish regularly (34,56,57).
Transport from photoreceptors to blood is important for ions and water. The RPE transports ions and water from the subretinal space (apical side) into the blood of the choroid (basolateral side). This process requires energy, and the Na (+)/K (+)-ATPase, on the apical membrane of the RPE, provides the energy (58). Tight junctions between RPE cells result in a barrier between the subretinal space and the choriocapillaris. Notably, paracellular resistance of this barrier is 10 times the transcellular resistance. For this reason, water cannot pass through the paracellular RPE pathway and passes mainly through the transcellular pathway via aquaporin-1 (59,60).
LIGHT ABSORPTION AND PROTECTION AGAINST OXIDATIVE STRESS
The retina is the only neural tissue in the body that is exposed to direct sunlight, which favors the oxidation of lipids and is toxic for the photoreceptors. Retina consumes proportionally more oxygen than other tissues, generating free radicals that are toxic to the cells of the retina. The RPE is essential to countering oxidative stress in the retina. The RPE cells contain pigments, such as melanin and lipofuscin, which absorb and filter the light. The RPE also produces antioxidant molecules including lutein, zeaxanthin, or ascorbate as well as enzymes, such as superoxide dismutase and catalase, that reduce reactive species (61).
PHAGOCYTOSIS
The RPE is a phagocytic system that is essential for the renewal of photoreceptors. Each photoreceptor has an inner and outer segment, the outer segment of each cone has a membrane folded 700 times, and the outer segment of every rod has about 1,000 discs (62).
The photoreceptors are often exposed to constant high light levels, which leads to accumulation of oxidized proteins and lipids. The concentration of photo-oxidized substances increases within the photoreceptors daily. The transduction of the light depends on the photoreceptor by appropriate functioning and structure of the proteins, retinyl, and cell membranes. Therefore, to maintain the excitability of the photoreceptor outer segments, they are undergoing constant renewal, reconstructed from its base. The tips of the outer segments of the photoreceptors, containing the highest concentration of free radicals, proteins, and photo-oxidized lipids, detach from the photoreceptors. Outer segments maintain a constant length through the coordinated release of its extremities and the formation of new discs. The discs detach from the outer segments and are phagocytosed by the RPE (63), which digest and deliver the essential molecules, such as docosahexaenoic acid and retinyl, back to the photoreceptors to rebuild their light-sensitive outer segments (32,64). Sometimes the content of the phagolysosomes are incompletely degraded, forming the residual bodies that are the substrates for lipofuscin formation. The residual bodies in the RPE accumulate with age, beginning as early as 16 months (65,66). The limited autophagocytic capacity of the RPE eventually results in an accumulation of metabolic waste in RPE cells throughout life. Dry AMD often begins in the parafoveal ring, where lipofuscin concentration is highest. However, lipofuscin disappears with cell death and a reduction in autofluorescent lipofuscin is a sign of progression toward late AMD.
SECRETION
RPE cells produce and secrete growth factors as well as essential factors for maintaining structural integrity of the retina and choroid. These molecules favor the photoreceptors’ survival and ensure a structural integrity for optimal circulation and nutrient supply. RPE secretes pigment epithelium–derived factor (PEDF), vascular endothelial growth factor (VEGF), fibroblast growth factors (FGF-1, FGF-2, and FGF-5), transforming growth factor–β, insulin-like growth factor I, neuronal growth factor (NGF), growth factor derived from the brain, neurotrophin-3, ciliary neurotrophic factor, several interleukins, chemokines, tumor necrosis factor–α, colony-stimulating factors, and various types of metalloprotease tissue inhibitors (67,68).
The most important factors with regard to the pathophysiology of AMD are PEDF and VEGF. PEDF is a neuroprotection and antiangiogenic factor. PEDF inhibits endothelial cell proliferation and stabilizes choriocapillaris endothelium. It is important in embryonic eye development. Mice lacking PEDF develop more aggressive retinal vascularization in ischemia (69). VEGF is secreted in low concentrations during physiologic conditions, thereby preventing endothelial cell apoptosis. In addition, VEGF regulates vascular permeability, thus stabilizing endothelium fenestrations (70).
IMMUNE PRIVILEGE
The eye is an immune-privileged site, and the RPE cells have an important role in the maintenance of this immune privilege (71,72). In 1993, Liversidge et al. indicated how RPE cells could inhibit lymphocyte proliferation (73). Many pathways have been suggested to explain RPE immunosuppression (74). On the one hand, RPE inhibits T-cell proliferation through secretion of prostaglandins and immunosuppressive cytokines. On the other hand, apoptosis and phagocytosis properties have demonstrated both directly (decreasing T-cell number and clearing apoptotic lymphocytes) and indirectly (down-regulating proinflammatory cytokine secretion, such as IL-1b) (75,76).
Ambati et al. (77) in 2003 postulated in a study of mice that there is an accumulation of complement fragments that might damage RPE and induce VEGF production by RPE cells, resulting in the development of choroidal neovascularization (CNV). This study provides insight into the role of macrophages and complement in the pathophysiology of AMD (78).
BRUCH’S MEMBRANE
BrM is a complex pentalaminar extracellular matrix (ECM), located beneath the RPE, and includes three layers: a central elastic layer sandwiched between two collagenous layers. BrM is lined by the basement membranes of the RPE and the choriocapillaris (basal laminae). Apart from its structural support function, BrM is also highly permeable to fluid and small molecules like oxygen, glucose, and other molecules necessary to maintain retinal health and function (79). Proteoglycans play an important role in BrM (80). Their negative charge impairs the passage of positively charged macromolecules that are essential for maintenance of RPE.
Changes in BrM begin in the third or fourth decade of life (5,81,82). These changes include the accumulation of membranous debris on both sides of the elastic lamina, drusen between RPE basal lamina and inner collagenous layer of BrM, and basal laminar deposits that may occupy the same space as drusen or develop between the RPE cell and its basal lamina (79) and appears as an amorphous material. How drusen develop and why they can vary in location and features are unknown.
Drusen usually contain fragments of RPE cells (9), a core of glycoproteins, an outer dome of crystallins (83,84), chaperone proteins, APOE, vitronectin, and proteins related to inflammation (amyloid P, C5, and C5b–9 complement complex) (85). Drusen are classified as either hard or soft. Hard drusen look like small, yellow nodules and are not associated with AMD (86). Soft drusen appear as large, white or light yellow, dome-shaped elevations that can resemble localized serous RPE detachments.
BrM doubles in thickness and declines in elasticity between the ages of 10 and 90 years. It contains no lipids during the first 30 years of life, but lipid concentrations rise in later years (4). In addition to the structural alterations that take place in BrM during aging, its fluid permeability becomes substantially reduced. BrM acts as a scaffold for the choriocapillaris and RPE cells, regulating their survival. When this function is diminished, anoikis occurs. Anoikis is an apoptosis phenomenon resulting from incorrect cell adhesion, and this process takes place in photoreceptors, RPE cells, and possibly endothelial cells (4) of the choriocapillaris. The development of either excessive material in BrM or erosion of BrM has important consequences. It may lead to choroidal endothelial cell loss, instigate chronic local inflammation, and produce ischemic stress on the oxygen hungry photoreceptor cells.
CHORIOCAPILLARIS
Choriocapillaris is a plexus of capillaries with fenestrations on the side facing BrM and is part of the innermost layer in the choroid. The capillary network is designed in a lobular pattern for rapid blood flow to supply the high metabolic demand of the photoreceptors and RPE (82). In aging eyes, the lumina of the choriocapillaris and the choroidal thickness are reduced by half. The resulting hypoxia stabilizes hypoxia-inducible factor 1α, which activates genes encoding proteins such as erythropoietin that protect the photoreceptors (87). Hypoxia also increases the secretion of growth factors such as VEGF A within Ruysch’s complex on the basal side of the RPE cells, causing development of choroidal neovascular membranes (4,88).
PATHOPHYSIOLOGY OF LATE AMD
Late-stage AMD shows the presence of RPE loss (cell death) or choriocapillaris attenuation without neovascularization in dry or atrophic AMD and exudative changes, neovascularization, or disciform scar in wet or neovascular AMD.
GEOGRAPHIC ATROPHY
Deposits of cellular debris in BrM alter the pathway of nutrient exchange between the RPE and choriocapillaris and lead to the downturn of RPE cells. At the same time, RPE cells overlying drusen are usually inflated and depigmented, and the adjacent photoreceptors are often damaged or dead (8). When the RPE degeneration is more advanced, thick basal laminar deposits take place in the BrM and obstruct nutrient exchange. These deposits may also decrease the adhesion of the RPE to the inner collagenous layer of BrM facilitating serous detachment of the RPE and retina (89,90).
Throughout their history, drusen reflect the state of the adjacent RPE cells. The greater the number of drusen and the larger they are, the greater the probability that the RPE cells will be damaged (8). Soft drusen easily became confluent and can reach a size that produces serous detachments of the RPE (91). Drusen sometimes disappear. Macrophages, pericytes, and choriocapillaris contribute to the removal, leading to their replacement by fibrous tissue.
By this time, RPE deterioration is critically advanced, and some cells may proliferate into small clumps, detach from BrM, and migrate into the retina. The final consequence of this process is geographic atrophy. Depigmentation exists in atrophic areas; however, at the edge of the lesions, pigmentation develops due to proliferation, hypertrophy, or phagocytosis of liberated melanin and lipofuscin (8,92). During the process of geographic atrophy, neural retina and damaged RPE cells disappear and the surviving rods and cones become shorter.
DISCIFORM SCAR
The main site of disturbance in neovascular AMD is BrM. However, the changes in BrM that predispose to neovascularization are unclear. With aging and as a consequence of membrane calcification, small fractures or gaps develop in BrM. Abnormal secretion from the deteriorating RPE cells might serve as attractant for endothelial cells, phagocytic cells, and lymphocytes, resulting in neovascularization. Lipofuscin accumulation, RPE membrane thickening, and intracellular residual body increase help stimulate new vessel formation. Therefore, new vessels occur as a consequence of an imbalance in the stimulating and inhibiting influences of growth factors, and any disruption in the diffusion through BrM to choroid could alter this balance. Once established, the new vessels have tendency to leak and bleed. When this occurs, the fluid extends laterally beneath the RPE in the same plane where basal laminar deposit and soften drusen are located.
There are three types of pigment epithelial detachment (PED) associated with AMD: drusenoid, serous, and fibrovascular. Drusenoid PED is a confluence of several soft drusen. Fibrovascular PED is a form of occult CNV. Serous PED is not exactly a breakdown in the outer BRB, it seems to develop from a dysfunction in the complex RPE cells–BrM. Fluid moves from the retina into the BrM as a result of active movement of ions by the RPE cells. If BrM became hydrophobic, resistance to water flow could cause fluid to collect between RPE and BrM (74). Serous exudates and blood may enter the retinal space. The retinal detachment may extend beyond the zone of RPE detachment and result in a rapid destruction of the RPE and photoreceptors over an area much larger than the region of vascular invasion, so the loss of vision is more severe in neovascular AMD than it is in geographic atrophy. Hemorrhage under the RPE or retina stimulates proliferation of fibrous tissue that produces a disciform scar, increasing the size of the area of blindness.
CONCLUSION
The main features of the AMD are the formation of drusen; thickening and other signs of damage of BrM; depigmentation and RPE hyperplasia; and accumulation of basal laminar deposits beneath the RPE. However, these are not unique or pathognomonic of this disease as we often observe them in most eyes of elderly individuals (93–96). In summary, the main signs of AMD increase with age, but only in some persons, they progress to the stage of cell death and functional loss. According to this point of view, AMD is an advanced stage of a process that occurs in all eyes. It is part of a common and continuing process in which the transition between age and disease is marked by loss of vision (9).
In 1972, Hogan (97) described the role RPE plays in the macula and postulated the existence of a gradual decrease in the activity of RPE, which resulted in accumulation of metabolic waste in BrM and loss of permeability. Zarbin (10) summarized five key concepts:
1.AMD includes disorders of aging and pathologic events.
2.Oxidative stress plays an important role in the damage of the RPE and BrM.
3.The RPE damage results in chronic inflammation of BrM and the choroid.
4.Choriocapillaris and RPE injury lead to the formation of an abnormal ECM that produces an alteration in the diffusion of nutrients between the retina and the RPE, which in turn causes more damage.
5.RPE and choriocapillaris atrophy occurs as well as CNV growth.
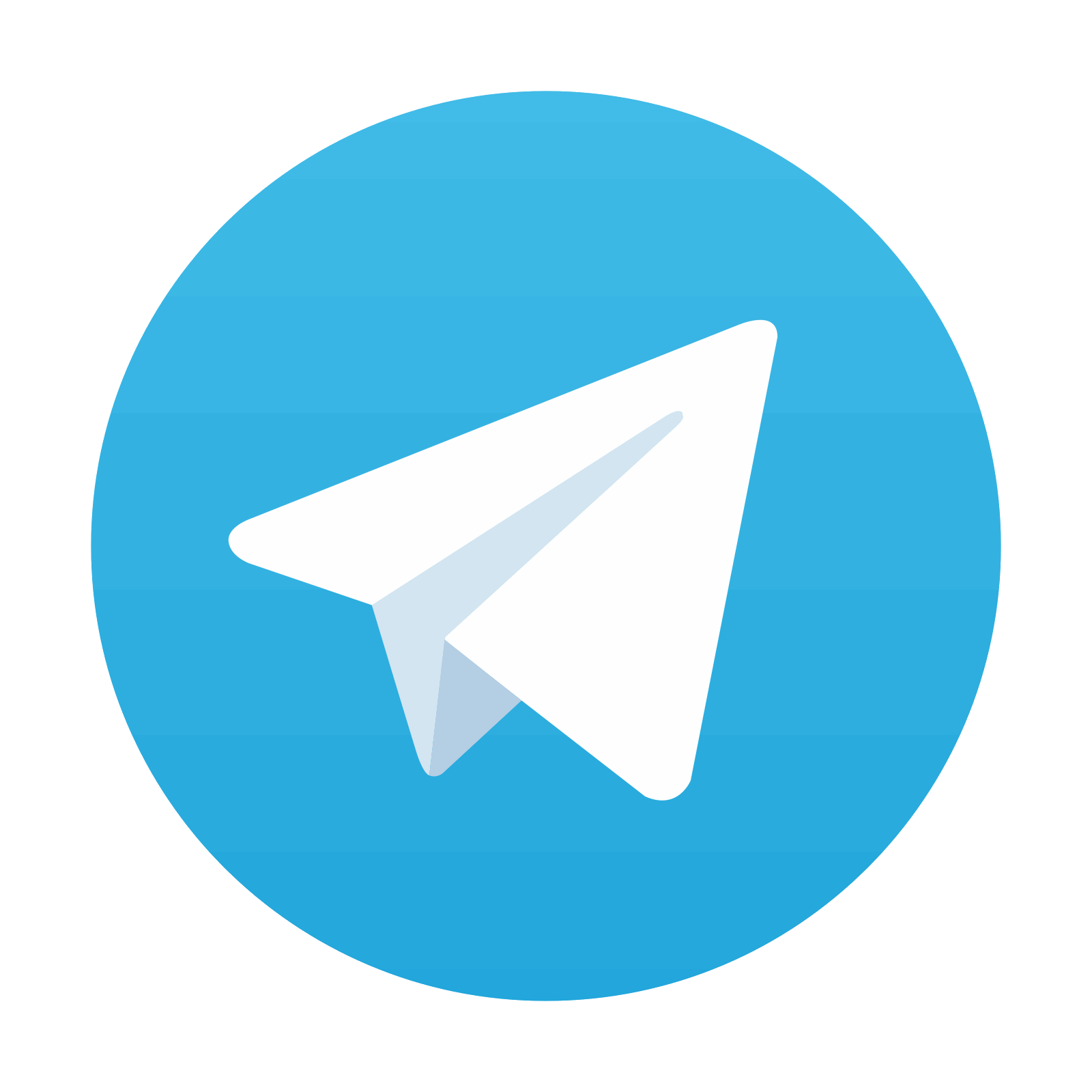
Stay updated, free articles. Join our Telegram channel

Full access? Get Clinical Tree
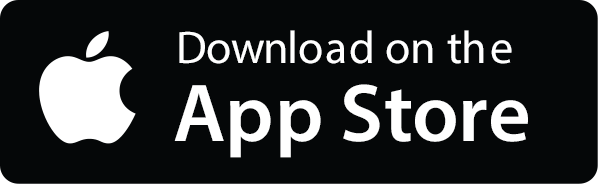
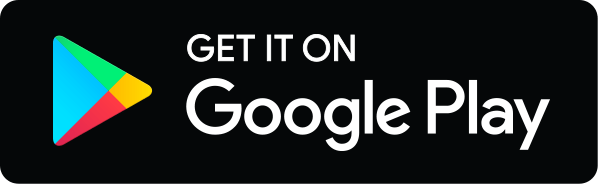