Chapter 2 Ocular Surface Defense Mechanisms Fig. 2.1 Immune defenses: the battleground The description of the functional anatomy of the conjunctiva occupies the largest section of this book. This is because conjunctival inflammation can only be understood if one has a thorough knowledge of the different factors and their interplay. This chapter recalls the fundamentals of inflammation, with a specific description of defense mechanisms protecting the ocular surface. The conjunctiva, by its location and function, is the ideal tissue for studying all types of immune responses. The text is abundantly illustrated with Figures, tables, and insets, in order to provide both an approachable and an exhaustive practical treatise. The authors are acutely aware of the ephemeral nature of some of the information presented here: immunology is a rapidly evolving science, and history abounds with beautifully constructed theories that are now obsolete or have simply turned out to be wrong. Our aim is thus to provide the reader with the best possible update of current knowledge on chronic conj unctival inflammation at the beginning of this new millennium. Besides its function as an anatomical barrier between the external environment and internal ocular structures, the conjunctiva has a fundamental protective role. It participates in the formation of the tear film and, by secreting mucins, contributes directly to its quality. Moreover, the conjunctiva is the inflammatory and immunological tissue protecting the ocular surface. The conjunctiva is highly vascularized, connected to the lymphatic network, and rich in immunocompetent cells that interact in a continuous, dynamic manner from the most superficial to the deepest ocular structures. As such, it contrasts markedly with the cornea, even though the latter is also in contact with the external environment. Although contiguous, the conjunctiva and the cornea are two very different structures: the conjunctiva possesses all the components required to react with maximum efficacy (and even violently) to all potential insults. This powerful reactivity is required, through the limbal vessels and the tear film, to protect the cornea by eliminating the aggressor as rapidly as possible. Maintenance of corneal transparency and integrity is a major requirement of the defensive mechanisms protecting the ocular surface. The immunological network of the conjunctiva is highly developed, whereas that of the cornea is minimal; in addition, corneal defense functions are partly inhibited. The immunoinflammatory response is a redoubtable weapon and can have detrimental effects by damaging healthy “bystander” structures. The immunological defensive system of the conjunctiva, designed for maximum efficacy, is organized on several levels into a complex network of communications, which are connected both with each other and with the systemic immune system. It involves all types of immunoinflammatory defense systems, the immense subtlety and extreme complexity of which we are only beginning to understand (Fig. 2.1). Scouts spot the enemy, capture those they can, and call in reinforcements—not just more sentries, but also reserve divisions composed of phagocytic cells and other cells capable of releasing signals that attract or activate a variety of killer cells, which eliminate the target either in a direct embrace or by producing potent weapons that kill or damage the assailant, hinder its progression, or increase phagocytic activity. The remains of the intruder are broken up, digested, and carefully analyzed, then transmitted to headquarters to be permanently memorized. The response to a new contact with the same enemy, anywhere in the body, leads to even more rapid destruction and elimination with cold, methodical efficiency. The external environment poses multiple and diverse threats that call for powerful responses; however, the cornea, a precious, yet fragile structure, must be preserved from excessive host reactions. A general, nonexhaustive overview of the different types of inflammatory response and their conjunctival specificities will be given to clarify the functional organization, and certain dysfunctions, of the tissues that compose the surface of the eye. The Inflammatory Reaction (Fig. 2.2) The inflammatory reaction in multicellular organisms consists of an ensemble of vascular, tissular, and humoral modifications in response to a threat to tissue integrity.1 According to the type of aggression and the nature of the assailant, the reaction can induce variable degrees of defensive reactions. However, all inflammatory reactions, whatever their precise type, almost always obey certain general principles. Inflammation begins with an acute vascular phase and continues with a cellular phase until healing is complete; sometimes, however, the inflammation may persist when the aggressor is not eliminated or the inflammatory process is uncontrolled. The vascular phase comprises, in chronological order, active congestion, edema, and leukocyte diapedesis (Fig. 2.3). Congestion corresponds to vasodilation and local slowing of the circulation, and occurs within a few minutes of the insult. This initial phase of the inflammatory reaction is under the dependence of several mediators, such as histamine, serotonin, leukotrienes, prostaglandins, and complement fractions C3 and C5. Edema results from local vascular hyperpermeability with extravasation of a protein-rich fluid into the threatened connective tissue. Histamine, bradykinin, and leukotrienes retract endothelial cells to allow the passage of large molecules. Leukocyte diapedesis (Fig. 2.3) is the process by which leukocytes cross the vessel wall. Congestion favors leukocyte stasis against the wall of dilated vessels. Adhesion molecules expressed on the surface of leukocytes and endothelial cells permit the adhesion of these cells and the passage oft the leukocytes through the vessel wall between endothelial cells: these adhesion molecules include E and P selectins on the endothelium and L selectin on leukocytes; ICAM-1 (intercellular adhesion molecule) and VCAM-1 (vascular cell adhesion molecule) on the endothelium, and their respective leukocyte ligands LFA-1 (leukocyte-function associated antigen) and VLA-4 (very late activation); the integrins are membrane receptors connecting the cellular cytoskeleton to neighboring cells or to the extracellular matrix. Expression of these molecules (the above list is not exhaustive, see Chapter 1, Tables 1.3–1.6) on both leukocytes and endothelial cells is controlled by a variety of cytokines released by inflammatory cells as soon as the insult is detected. Fig. 2.2 General diagram of inflammation Fig. 2.3 Vascular phase of the inflammatory reaction Attracted to the target tissue by chemotactic factors, inflammatory cells exert their multiple functions, chief among which are phagocytosis, enzymatic digestion of the assailant, and its “identification,” so that subsequent contacts engender even more rapid, effective, and specific reactions, through a phenomenon known as immune memory. Once the acute phase is over and the assailant has been eliminated, the tissue, which often suffers more from the inflammatory response than from the aggressor itself, must be repaired: depending on the injured tissue, fibroblasts, vascular endothelial cells, or epithelial cells arrive to replace inflammatory cells and to repair the damage. This phase begins on day 3 or 4, before the end of the acute phase. Leukocyte apoptosis regulates the inflammatory infiltration and leads to its disappearance when it is no longer required and when elimination of the aggressor gives place to tissue repair. When this mechanism is dysfunctional, activated lymphocytes persist in the tissue and prolong the inflammatory reaction: this is one way in which autoimmunity can occur. Sometimes it is the aggression that persists or recurs. In such cases the persistence of inflammatory cells and the continued secretion of cytokines, proteolytic enzymes, and free radicals means that the local inflammatory reaction become chronic. Granulomatous inflammation occurs when phagocytes turn into epithelioid cells and multinucleated giant cells, and form nodules, as in sarcoidosis and tuberculosis, for example. In some cases the tissue is attacked not by an exogenous aggressor but by the body itself. This is notably the case in lesions linked to deposits of circulating immune complexes in the vessel walls, and autoimmune reactions in which autoantigens are erroneously attacked by the lymphocyte system, either because of structural analogies with the aggressor, or because of a malfunction of self-tolerance. This can occur when an inflammatory lesion leads to the recognition of proteins that are sequestrated or modified by a viral infection, or even by the initial inflammatory reaction. Main Actors of Inflammation The immune system comprises a number of specialized organs and cell types responsible for specific recognition of antigens that must be eliminated and destroyed. In normal circumstances, most immune cells are almost exclusively located in primary or secondary lymphoid organs such as the thymus, spleen, lymph nodes, and other lymphoid tissues (tonsils, Peyer patches, etc.). However, other immune cells, such as lymphocytes and dendritic cells, may reside or be transiently present in nonlymphoid tissues. Dendritic Cells and Langerhans Cells B cells and some T cells, especially helper T cells, are not capable of recognizing antigens unless the latter are accompanied by major histocompatibility complex (MHC) class II antigens. This role is fulfilled by specialized antigen-presenting cells (APCs), including mononuclear cells and dendritic cells. These cells are generally the first actors in the inflammatory response, which is initially nonspecific and subsequently immunological, i.e., specific to the aggressor. Dendritic cells initiate many immune responses, such as T cell sensitization (requiring compatibility with MHC class II antigens), organ transplant rejection, and T-dependent antibody production. Dendritic cells are found in many nonlymphoid tissues but can migrate via the afferent lymphatics or blood stream to T-dependent regions of lymphoid organs. In the skin, the immunostimulatory functions of dendritic cells are potentiated by cytokines, especially granulocyte macro-phage-colony stimulating factor (GM-CSF) and tumor necrosis factor alpha (TNF-α).2 At this stage the expression of adhesion molecules and the appropriate costimulatory signals are essential for the immunogenic response.3 High levels of MHC class I and class II antigens and many adhesins such as ICAM-1 and LFA-3 contribute to the efficiency of these functions. The cycle of dendritic cells can be summarized as follows (Fig. 2.6): (a) migration of bone marrow-derived immature precursors into the vessels of the surrounding tissues (epidermis, intestinal mucosa, respiratory epithelium, conjunctival epithelium, etc.); (b) maturation and formation of a network of resident dendritic cells that capture, internalize, and metabolize antigens; (c) activation and migration via the afferent lymphatics to T-dependent regions of the draining lymphoid organs, where they acquire optimal antigen-presenting functions, probably under the influence of cytokines. Presentation of antigens trapped by resident tissue dendritic cells to T cells leads to activation and proliferation of the latter in draining lymph nodes.4 Table 2.1 Immunophenotypic characteristics of dendritic cells in the conjunctival epithelium33
Antigen | Function/Distribution | Positivity on Dendritic Cells | |
---|---|---|---|
Conjunctiva | |||
Normal | Inflamed | ||
HLA-DR | APCs | ++ | ++ |
HLA-DQ | APCs | + | + |
CD1A | Participates in antigen presentation | ++ | ++ |
CD2 | Lymphocyte adhesion to target cells | – | – |
CD4 | Helper T cells: antigen recognition | – | +/- |
CD5 | T cell activation | – | – |
CD8 | Suppressor/cytotoxic T cells Antigen recognition | – | – |
CD11A | LFA-1 α chain (immunocompetent cells) | + | + |
CD11B | MAC-1 α chain (immunocompetent cells) | +/- | +/- |
CD11C | Integrin α (immunocompetent cells) | – | – |
CD14 | Lipopolysaccharide receptor (macrophages) | + | + |
CD18 | LFA-1 β chain (immunocompetent cells) | + | + |
CD21 | C3d and Epstein-Barr virus receptor (dendritic cells, B lymphocytes) | – | +/- |
CD22 | B cells, CD45RO ligand | +/- | +/- |
CD23 | IgE receptor | – | – |
CD25 | IL-2 receptor | – | – |
CD29 | Integrin β1, adhesion to cells and matrices | – | – |
CD32 | IgG receptor | +/- | +/- |
CD36 | Collagen receptor | +/- | +/- |
CD41 | Integrin αIIb | – | – |
CD45RO | CD22 ligand (immunocompetent cells) | + | + |
CD50 (ICAM-3) | LFA-1 ligand | + | + |
CD54 (ICAM-1) | LFA-1 and MAC-1 ligand | +/- | +/- |
CD61 | Integrin β3 | – | – |
CD62 | Selectin | – | – |
CD63 | Polymorphonuclear cell and monocyte adhesion | + | + |
CD71 | Transferrin receptor | – | – |
Tryptase | Mast cells | – | – |
Vimentin | Mesenchymal cells | ++ | ++ |
Fig. 2.4 Dendritic cells on a normal conjunctival impression cytology sample (in green) (HLA DR marker), surrounded by superficial epithelial cells (red-stained nuclei)
Fig. 2.5 Dendritic cells in the subepithelial stroma of the conjunctiva (in green)
Fig. 2.6 The dendritic cell cycle
Fig. 2.7 Conjunctival Langerhans cells (in green) (CD1a marker) on a conjunctival impression cytology sample
Fig. 2.8 Increased Langerhans cell density during conjunctival allergy (HLA-DR marker)
Fig. 2.9
a Langerhans cell (arrows) in the suprabasal position within the limbal conjunctival epithelium (TEM, x 3500)
b Langerhans cells contain Birbeck granules (asterisks) and are not connected to neighboring cells by desmosomes (TEM, x 60 000)
Intraepithelial dendritic cells are particularly abundant at sites strongly exposed to antigens, such as the epidermis and conjunctival epithelium; these cells are known as Langerhans cells. In these sites they express the CD1a surface antigen and form a highly organized network reflecting their sentry role in immune defenses5 (Fig. 2.7). The iris, the ciliary body, and the choroid are also rich in dendritic cells.6
Mature dendritic cells are inefficient phagocytes compared to macrophages, for example, but they are very powerful APCs. Mature dendritic cells are the only cells capable of initiating a primary immune response, i.e., of activating T cells during initial contact with an antigen. At a more immature stage they resemble macrophages, having strong antigen capture potential and being unable to stimulate naive lymphocytes.
Langerhans cells also have other characteristics that give them a recently recognized role in allergic phenomena (Fig. 2.8), especially IgE-linked delayed hypersensitivity, an allergic reaction that combines symptoms of contact eczema (classically a type IV hypersensitivity reaction) and mechanisms involving IgE (theoretically type I hypersensitivity). Langerhans cells possess at least three different types of surface receptor for IgE. Binding of certain antigens to IgE on the surface of dendritic cells may trigger their activation, cytokine synthesis, and stimulation of the immune system via the humoral Th2 system, leading to chronic allergy.
Macrophages (Fig. 2.10)
Macrophages are bone marrow-derived cells widely distributed throughout the body. They play a key role in cellular immunity, as inflammatory, tumoricidal, and microbicidal effectors. Moreover, through active secretion of a large number of molecules, such as TNF-α and nitric oxide (NO), macrophages play a role in other processes such as immune regulation, tissue regeneration (synthesis of elastase, collagenase, fibroblast growth factor, etc.), and angiogenesis.7,8
Phagocytosis, perhaps the most important macrophage function, is an important defense against microbes, supplementing the activity of polymorphonuclear neutrophils. Macrophage endocytosis is particularly efficient for proteins, viruses, bacteria, tissue debris, and other cells (particularly if they are opsonized via the immunoglobulin Fc receptor, complement receptors, and mannose receptors). Binding of an opsonized particle to the cell surface receptor triggers the formation of pseudopods that surround the particle and enclose it in a phagocytic vacuole.
Macrophages can express class II antigens and, to some extent, the CD1a molecule, conferring a role in antigen presentation.9 This role is clear in viral infections, for example, in which CD1a expression on macrophages increases, probably after stimulation by gamma interferon. Moreover, macrophages, by phagocytosing large insoluble protein antigens, can bolster the action of dendritic cells, which have limited phagocytotic capacity.10
Fig. 2.10 Macrophages
Fig. 2.11 Intraepithelial lymphocytes (arrows) between basal cells (TEM, x 8000)
Lymphocytes (Fig. 2.11)
Lymphocytes derive from multipotent stem cells in the bone marrow, and evolve into two functionally different populations: T cells and B cells.11–13 T cells differentiate in the thymus and B cells in the fetal liver and spleen and adult bone marrow. T and B cells, which are morphologically identical, can only be distinguished by their surface markers and related functions.
Fig. 2.12 B lymphocytes
Fig. 2.13 T lymphocytes
Fig. 2.14 T lymphocyte differentiation into Th1 and Th2 cells
B Lymphocytes (Fig. 2.12)
B cells, which represent 5-15% of the circulating lymphocyte pool, are the cornerstone of humoral immune reactions. Their membrane is covered with immunoglobulins that serve as antigen receptors. These surface immunoglobulins are mainly of the IgM and IgD classes. B cells also possess class II antigens and receptors for some complement fractions and many other mediators and growth factors. Contact with the antigen for which a given B cell is specific leads to its activation and transformation into a plasma cell, which synthesizes immuno-globulin and releases it into the circulation. Each plasma cell synthesizes antibodies with a single specificity and immuno-globulin class.
The initial B cell response after contact with an antigen is to produce IgM, followed by IgG. This activation requires two types of effector: an APC and helper T lymphocytes, which release growth factors and facilitate the humoral response.
T Lymphocytes (Fig. 2.13)
T lymphocytes participate indirectly in antibody synthesis, but are mainly involved in cellular immune reactions (cytotoxicity, transplant rejection, delayed hypersensitivity, etc.). T cells are continually circulating between the blood, the lymph, the lymph nodes, the spleen, and nonlymphoid tissues. The “homing” mechanism of T cells involves interaction with ligands or adhesion molecules on the vascular endothelium, both in lymphoid organs and also at sites of inflammation.14 Circulating lymphocytes cross the endothelium of post-capillary venules, through activation mechanisms. When tissue inflammation occurs, cytokines (gamma interferon, interleukin-1 [IL-1 ], TNF) induce endothelial cells to express integrins and selectins (especially P and E), permitting lymphocyte migration through the vessel wall.
T lymphocytes are in fact a fairly heterogeneous group of cells with helper, cytotoxic, or suppressor functions. They can be subdivided on the basis of their phenotypic characteristics by the use of specific antibodies binding to their membrane markers. Immunophenotypic and functional studies have shown that T lymphocytes are distributed into two main populations—helper (CD4+) and suppressor (CD8+) cells—which both comprise functional subpopulations (naive cells, memory cells, etc.).
The Th1/Th2 System (Fig. 2.14)
Characterization of helper (CD4+) T lymphocytes led to the identification of two distinct functional subpopulations that differ not by their membrane markers but by the mediators they produce and the immune reactions they induce.12,13 Th1 lymphocytes produce cytokines that activate macrophages, stimulate IgA and IgG secretion by B cells, and orient inflammatory reactions toward delayed-type hypersensitivity mechanisms. The main cytokines involved in Th1 reactions are IL-2, IL-12, gamma interferon, and TNF.
Th2 lymphocytes mainly favor the recruitment and differentiation of mast cells and eosinophils, and IgE production. The main cytokines involved in these reactions are IL-3, IL-4, and IL-5, and GM-CSF. Interleukin-4 is a key factor in Th2 activation. Interleukin-3 stimulates mast cells, while IL-5 stimulates eosinophils. In turn, these activated cells release IL-3 and IL-4, and thereby amplify the Th2 response. Th2 cells are thus directly involved in allergic and antiparasitic reactions.
However, “naive” T lymphocytes, which have not yet encountered an antigen, have an undifferentiated profile which is potentially either Th1 or Th2. Initial contact with an antigen triggers one or other type of reaction, through a poorly understood mechanism that appears to involve the type of antigen, its size (large antigens tend to induce Th1 reactions), its concentration (low concentrations tend to induce Th2 responses), and stimulatory cofactors released by the APC (the presence of gamma interferon and/or IL-2 induces a Th1 response, while IL-4 induces a Th2 response). In addition, the two systems are mutually inhibitory: gamma interferon produced by Th1 lymphocytes blocks Th2 reactions and reduces IgE secretion; in contrast, IL-10, secreted by Th2 lymphocytes, inhibits gamma interferon synthesis, reduces the density of class II molecules on APCs, and thus reduces Th1 cell stimulation.
Little is known of the way in which these systems operate specifically in the ocular environment, but the accumulation of Th2 cells in vernal conjunctivitis15 shows their role in ocular allergy. Recently, another subpopulation of T cells, designated γ/δ T cells, was identified. These cells have a predilection for epithelial sites, and appear to be able to attenuate local adverse immune responses.16,17
Interactions of T and B Lymphocytes with Antigen-Presenting Cells
The distinction between humoral and cellular immune responses is in fact highly artificial, as most antigens trigger a mixed response with a predominance of one or other mode. Interactions between the different types of immune effector cells are always essential, however. In most cases B cell activation requires the activation of helper T cells and involves class II antigens. Helper T lymphocytes possess a specific antigen receptor named T-cell receptor (TCR). T lymphocytes corresponding to a given antigen can be activated following contact with the specific determinant delivered by an APC (Fig. 2.15) or bound to the surface immunoglobulin of a B lymphocyte. It should be noted that some “thymoindependent” antigens can themselves activate B cells without the assistance of T cells, although they only account for a minority of immune reactions. T cell stimulation always involves simultaneous presentation of the antigen with an MHC class II molecule. T lymphocytes appear to possess either two independent receptors for the two types of molecule, or a receptor for the complex. In the absence of class II antigens (or if the class II antigen is blocked by a specific antibody), the helper T lymphocyte cannot be activated and the immune response is inhibited. This phenomenon is known as class II antigen restriction. Once activated, T lymphocytes secrete soluble mediators (which may or may not be specific for the antigen), in turn recruiting and activating other helper T cells and B cells.
Mucosa-Associated Lymphoid Tissue
Mucosa-associated lymphoid tissue (MALT) is found in the gastrointestinal, respiratory, urogenital, and conjunctival mucosae (conjunctiva-associated lymphoid tissue [CALT]). Lymphocytes in these regions form loose aggregates or are organized into true secondary follicles with germinative centers.18 They contain T cells, B cells, and immunoglobulin-secreting plasma cells. In the substantia propria, 70% of T lymphocytes are CD4+, while 30% are CD8+. There are also intraepithelial lymphocytes, most of which are CD8+ T cells expressing the CD45RO isoform (characteristic of memory T cells), and the human mucosal lymphocyte antigen, HML-1, an integrin which is a marker of T cell activation. Mucosal antibody responses are mainly of the IgA type. Secretory IgA coating the mucosal surface hinders invasion by infectious agents. To resist the action of proteolytic enzymes, IgA is secreted in dimeric form bound to the secretory component, which is derived from the receptor which mediates transepithelial transport of IgA. Through the cell homing mechanism (Fig. 2.13), lymphocytes activated at a mucosal site migrate to the regional nodes and thence to the blood. They are then distributed throughout all the mucosae, through the action of specific endothelial adhesion molecules (integrins) (Chapter 1, Tables 1.3–1.6). This mechanism ensures that all the mucosae are defended, even after a single contact with the antigen on a single mucous membrane.
Fig. 2.15 Antigen presentation
Mast cells are large, round or oval cells with a central nucleus and abundant granulations. They are normally present in connective tissue, skin, and mucosae. They are often located near lymphatic and blood vessels, and around nerves. Their peri-vascular location is important in inflammation, as their activation leads to vasodilation, increased vasopermeability, and leukocyte extravasation. Mast cells are often compared with blood polymorphonuclear basophils, with which they share certain characteristics, such as high-affinity surface receptors for IgE, and histamine, proteoglycan, and protease storage.19
Antigen attachment to the IgE that coats the mast cell surface membrane leads to IgE aggregation; this triggers abrupt mast cell degranulation and the release of mediators stored in granules. This is the key event in immediate hypersensitivity reactions (anaphylactic reactions), allergic reactions, and anti-parasite defenses. The different cytoplasmic organelles (especially the Golgi apparatus) synthesize granular contents, generating a new mature mast cell. Another mode of degranulation, known as piecemeal degranulation, is slower and poorly understood. It appears to be involved in many human disorders, such as contact allergy, bullous pemphigoid, primary and secondary malignant tumors, and chronic inflammation. The granules remain in the cytoplasm, but some are partially or totally emptied of their contents. Synthesis of granular contents and reconstitution of the mature mast cell occur gradually.20
Fig. 2.16 Mast cell activation and degranulation
Fig. 2.17 Diagrammatic representation of human mast cell granule synthesis and degranulation20
Fig. 2.18 Conjunctival mast cells labeled (green) with tryptase
Other molecules can activate mast cells, such as certain complement fractions (C3a and C5a), drugs (e.g., codeine), insect venom, and even cytokines (IL-1, IL-3, IL-5, IL-8, and gamma interferon).
Another mode of mast cell activation and degranulation involves the nervous system, with release of neuropeptides such as substance P, gastrin, vasoactive intestinal peptide (VIP), somatostatin, endorphin, and opioid peptides. There is thus an orthodromic current coming from the damaged tissue to the central nervous system, and an antidromic current provoking the release of these neuropeptides by nerve endings, leading to mast cell degranulation and stimulating the inflammatory reaction.21
Activated mast cells release a variety of mediators. Some are preformed in cytoplasmic granules, such as histamine, tryptase (which activates C3 to C3a, itself a mast cell degranulating agent), kallikrein (which activates proinflammatory kinins), TNF-α, heparin, vasoactive amines, chemotactic factors, and enzymes (proteases and hydrolases). Histamine is one of the main mediators involved in allergic reactions. It plays a role in capillary vessel regulation via H1 and H2 receptors. Through H3 receptors it also acts as a neuromediator with a central influence on vigilance, and a peripheral effect on nerve endings. Histamine release during mast cell degranulation is responsible for immediate allergic reactions (edema, vasodilation, pruritus, and, according to the organ, sneezing, tearing, rhinorrhea, bronchospasm, etc.). Histamine can also be released in a delayed manner, more than 24 hours after initial activation, during the late phase of the allergic reaction.
Other mast cell mediators are synthesized in response to an activation signal. The action of phospholipase A2 on membrane phospholipids permits the synthesis of arachidonic acid derivatives, prostaglandins, leukotrienes, and platelet-activating factor (PAF), which have powerful inflammatory properties. Mast cells also produce many cytokines, including IL-1, IL-3, IL-4, IL-5, IL-6, gamma interferon, transforming growth factor ß (TGFβ), and GM-CSF, which are involved in nonspecific inflammatory reactions. They can alter vascular endothelial cells, provoke leukocyte migration, activate coagulation, and have an autocrine or paracrine action on mast cells themselves and on other lymphoid cells.22 Thus, the initiation and development of many inflammatory and immune responses may be physiologically dependent on mast cell-derived cytokines, independently of any “allergic” reaction (Table 2.2).23
Fig. 2.19 Mast cells:
a Mast cells are characterized by multiple expansions of their cytoplasmic membrane and by the presence of dense granules in their cytoplasm (TEM, x 12 000)
b Some granules have a scroll-like appearance (asterisk) (TEM, x 50 000)
Polymorphonuclear Eosinophils
Polymorphonuclear eosinophils, like neutrophils, are circulating granulocytes with mediocre phagocytic capacity. Eosinophils remain in the blood for 6-12 hours, until an interaction between an integrin and an endothelial adhesion molecule (ICAM-1, endothelial leukocyte adhesion molecule-1 [ELAM-1 ], etc.) in the post-capillary venules allows them to adhere and migrate to extravascular tissues.24 After entering connective tissue, eosinophils remain in the perivascular space for a few days. Human eosinophils possess receptors for complement and the Fc portion of IgG, IgA, IgM, and IgE. Eosinophil granules contain proinflammatory proteins (major basic protein [MBP], eosinophil cationic protein [ECP], eosinophil-derived neurotoxin [EDN], eosinophil peroxidase [EPO], etc.), PAF, and hydrogen peroxide and superoxide anions, which are the main weapons used to eliminate parasites (especially helminths), as well as bacteria (including mycobacteria). Eosinophils also release histaminase and arylsulfatase, which inactivate substances produced by mast cells such as histamine and slow-reacting substance of anaphylaxis (SRS-A). This tends to attenuate the inflammatory response and to limit granulocyte migration to the inflammatory site.
Table 2.2 Mast cell activities23
Leukocytes Adhesion Chemotaxis Phagocytosis IgE production Mast cell proliferation Polymorphonuclear eosinophil activation |
Fibro blasts Proliferation Collagen synthesis |
Vessels Increased venous permeability Leukocyte adhesion Constriction Dilation |
Phagocytosis Bacteria Parasites |
The Weapons: Cytokines and Chemical Mediators
All cells involved in inflammatory responses communicate with one another via abundant and complex signals that have multiple and sometimes apparently conflicting roles in regulating what is potentially a highly destructive process. Cell-cell communication occurs either through contacts via membrane receptors, or more often at a distance via cytokine release.
Inflammatory Cytokines (Tables 2.3 and 2.4)25a,25b
The first cytokine to be discovered was gamma interferon, which was initially described in 1957. Besides the interferons, cytokines include hematopoietic growth factors (granulocyte colony-stimulating factor [G-CSF], macrophage colony-stimulating factor [M-CSF], and GM-CSF), interleukins, chemotactic factors, chemokines, TNF, and some growth factors. Their mode of action can be paracrine (acting on neighboring cells), autocrine (activating receptors on the cells that synthesized them), or endocrine (acting at a distance, via the circulation)25(Fig. 2.20). All inflammatory cells secrete cytokines and thereby contribute to the orchestration of immunoinflammatory reactions.
The principal cytokines are IL-1 and TNF-α. Secreted by macrophages, T lymphocytes, polymorphonuclear cells, endothelial cells, epithelial cells, and fibroblasts, these two cytokines have multiple properties that enable them to participate in inflammatory reactions such as vasopermeability, cell activation, and stimulation of the synthesis of inflammatory proteins such as prostaglandins, PAF, leukotrienes, free radicals, and proteases. Other cytokines have partial or more limited actions that may be additive, agonistic, or antagonistic.
Most of these molecules have specific antagonists that are designed to limit the extension (in space or time) of deleterious inflammatory reactions. That is particularly so in the eye, where immune reactions run the risk of being more harmful than the insult itself. All proinflammatory proteins induce their own antagonists. For example, IL-1-producing cells also secrete the IL-1 receptor antagonist (IL1-ra), that binds to the same receptors but does not induce a cell-activating signal. As IL-1 receptors are more sensitive to the native molecule, the reaction is initially proinflammatory. Then, as the level of IL1-ra increases, competition occurs between the two proteins for their receptor, until the signal is extinguished when IL1 -ra gets the upper hand. Interleukin-4, -10, and -13, and TGF-J3 also inhibit IL-1 production.
Fig. 2.20 Modes of cytokine action
There is also a receptor-mediated regulatory mechanism: IL-1 possesses two receptors, but only one induces the activation signal; the other appears to act as a decoy, as its activation has no biological effect.25 Interleukin-1 can also be neutralized by soluble forms of its own receptors. This is also true of TNF-α, which is mainly regulated by soluble receptors.
Cytokines compose a form of alphabet, each molecule exerting actions that vary greatly according to the cells that secrete them, their environment, their target cells, and their association with other cytokines. Numerous combinations are possible, offering the cells involved in inflammatory reactions a very refined means of communication. One example of this complexity is IL-4, which inhibits IL-6 production by monocytes but increases IL-6 production by fibroblasts and B cells.
The organization of the cytokine network is thus extremely complex; in addition, its equilibrium is fragile and our knowledge of this system is still very fragmentary and continually evolving. It is not, therefore, surprising that even a minor deviation of the complex ocular inflammatory process, which is subject to a very particular regulatory mechanism, can lead to highly noxious reactions, readily becoming chronic when the immune response becomes excessive.
Immunoglobulins
Immunoglobulins11–13 are a family of structurally related molecules, the main function of which is the specific recognition of antigens (antibody function), but which also possess other properties (effector functions), such as complement activation and binding to cellular receptors. The backbone of the immunoglobulin molecule is composed of four polypeptide chains, two of which are heavy (molecular masses of approximately 50 000-60 000 Da) and two of which are light (25 000 Da each). The nature of the heavy chain and the disposition of the different basic units define five classes of immunoglobulins: IgG, IgM, IgA, IgD, and IgE.
IgG (approximate molecular mass: 150 000 Da) is the most abundant immunoglobulin in the body. Its structure corresponds to the basic model described above. Two Fab fragments bear the antibody activity and the Fc fragment possesses several biological properties, such as binding to many cell types, complement binding, transplacental passage, and its own antigenic characteristics (immunoglobulin is itself an antigen that can be recognized by specific antibodies).
IgM is composed of five subunits linked by disulfide bridges and a junctional J chain. A very voluminous molecule, IgM possesses 10 antibody sites and binds complement. IgA molecules are dimers, and are the principal immunoglobulin species found in external secretions. IgD, like IgE, is composed of only one unit, with four chains. IgD remains at the surface of B cells and acts as an antigen receptor. IgE, secreted in tiny amounts, represents the antibodies involved in immediate hypersensitivity reactions.
The heterogeneity of immunoglobulin occurs at three levels, and is determined by genes coding for the different portions of the heavy and light chains: isotypic, common to all members of the same species; allotypic, proper to each individual; and idio-typic, proper to all the immunoglobulin molecules synthesized by a given plasma cell. Whatever its precise nature, immuno-globulin possesses an entirely specific region that reacts only with a precise antigenic structure, in a lock-and-key configuration. This idiotypic specificity reflects the fundamental notion that a given plasma cell secretes an immunoglobulin of a single species, specific for a single antigen. To defend itself the body must therefore possess all possible varieties of lymphocytes, synthesizing immunoglobulins directed against all known types of antigens. When the body encounters an antigen, the lymphocyte for which it is specific is stimulated and secretes the corresponding antibodies. These immunoglobulins, which can only recognize a single antigen (or highly similar antigenic sites), and are synthesized by only a single specific lymphocyte and its daughter cells, are called monoclonal antibodies.
Table 2.3 Maincytokines involved in inflammatory and immune responses25a,25b
Table 2.4 Cytokines secreted by ocular surface cells
Conjunctival epithelial cells | IL-1, IL-6, IL-8, IL-10, GM-CSF |
Corneal epithelial cells | IL-1, IL-6, IL-10 |
Langerhans cells | IL-1 |
Fibroblasts | IL-1, IL-3, IL-6, IL-8, Interferon γ |
Keratocytes | IL-1, IL-3, IL-6, IL-8, Interferon γ |
Vascular endothelium | IL-6, IL-8 |
Neurons | Substance P |
Mast cells | IL-1, IL-4, IL-6, IL-7, Interferon γ |
Th1 lymphocytes | Interferon γ |
Th2 lymphocytes | IL-4, IL-5, IL-6, IL-10 |
B lymphocytes | IL-1, IL-4, IL-6, IL-10, M-CSF, G-CSF TGF-α/β, interferon α/β, TNF-α |
Macrophages | IL-1, IL-3, IL-4, IL-6, IL-8, IL-10, M-CSF, G-CSF, GM-CSF, TGF-α/β, interferon α/β, TNF-α |
Complement System (Fig. 2.21)
The complement system is a group of soluble proteins that bolster the efficacy of the humoral defense system against infectious agents. More than twenty different molecules are known to be involved in the complement system, and they interact in cascade fashion in the same way as coagulation factors. They are normally present in the circulation in inactive form. There are two known activation pathways: the classical pathway, triggered by immunoglobulin (IgG or IgM) binding to an antigen, and the alternative pathway, which is activated by bacterial polysaccharides or aggregated IgA. These two pathways both lead to the formation of a lytic protein complex capable of altering cell membranes and disrupting target cells.
Some complement fractions also have vasodilatory and chemotactic properties and activate B cells and polymor-phonuclear cells. They can also lead to the aggregation of immune complexes, inactivate certain viruses, and promote phagocytosis of antibody-coated (opsonized) antigens.
Free oxygen radicals (superoxide anion, the hydroxyl radical, peroxyl radical, and NO derivatives) are hyperreactive chemical species possessing a single electron. With a life span of about a microsecond, they trigger a very strong oxidative reaction that preferentially targets double bonds of polyunsaturated fatty acids, and some amino acids, leading to membrane disorganization and cell destruction.26
Free radicals are produced in large quantities by phagocytes, polymorphonuclear cells, and macrophages, and are the main mechanism used to destroy microbes, foreign bodies, and necrotic tissue debris. They can also be released into the extracellular medium, where they may cause major tissue damage, itself a source of further inflammatory reactions. Free radicals have a proinflammatory action: at sub toxic concentrations they favor polymorphonuclear cell adhesion and accumulation, as in ischemia-reperfusion, for example.27
Fig. 2.21 Complement components
The Battle Plan: Tactics and Strategies
The different types of inflammatory cell interact in a complex manner in order to produce a response tailored to the aggressor, with the most effective mediators, while at the same time preparing the response to later contacts.
Antigen Presentation
The first type of interaction is between CD4+ helper T lymphocytes and APCs (macrophages, Langerhans cells, dendritic cells, or even B lymphocytes) that capture the antigen, internalize it, and present it to T lymphocytes, in conjunction with the class II antigens they express. Endothelial cells, fibroblasts, and astro-cytes are accessory APCs, whereas Müller cells tend to be suppressive. The activation capacity of APCs is highly variable: it only takes 60 peptide class II antigen complexes on the surface of a macrophage to activate a T lymphocyte11; whereas a fibro-blast requires more than 5 000 complexes.
The antigen is presented to the T lymphocyte in association with a class II antigen, the complex being recognized by the TCR receptor of helper T cells. However, other signals are necessary too (Fig. 2.15), such as membrane signals in the form of complementary receptors that favor contact between the two cells and also promote lymphocyte activation (ICAM-1/LFA-1; LFA-3/CD2, CD5/CD72, etc.); soluble signals secreted by the APC, such as IL-1 and IL-6, activate T cells and increase IL-2 receptor expression. These actions permit the recruitment and activation of new T cells.
Certain complex and poorly understood interactions can also lead to lymphocyte inactivation, in a phenomenon known as T and B cell anergy. This mechanism is probably designed to hinder the activation of self-reactive lymphocytes directed against autoantigens. Disruption of these protective mechanisms would favor the emergence of autoimmunity.
Most antigens follow the classical pathway (APC interaction with T lymphocytes then B lymphocytes), but thymoindependent antigens can activate B cells without the help of T cells, and superantigens can activate T lymphocytes nonspecifically, without the participation of class II antigens. Some molecules, known as haptens, only become immunogenic when coupled to a carrier molecule.
Humoral and Cellular Responses
Helper T cells play a key role by coordinating chemical and cellular responses (via cytokines) to the antigen. According to the type of stress, they can stimulate either all immunocom-petent cells or only specific cell types (phagocytes, B lymphocytes, cytotoxic T cells, natural killer [NK] cells or killer [K] cells), dependent on antibodies, mast cells or eosinophils.
Helper T cells can select the type of response according to the cytokines secreted and certain antigen-related factors, together with the mode of antigen introduction and the initial target organ. B cell activation leads to a humoral response and antibody production. The secondary response differs from the primary response (on first contact with the antigen) in several ways: it is triggered more rapidly but expands more slowly, and the plateau phase is longer; antibody titers are approximately 10 times higher than during a primary response. Furthermore, the secondary response is mainly composed of IgG (not IgM), and antibody affinity is far higher. This increased rapidity and efficacy of the response after secondary contacts is due to the expansion of antigen-specific lymphocyte clones during the primary response. This phenomenon increases the chances that the specific clone will encounter the antigen during a subsequent challenge, and is known as the immune memory.
In many cases the antibody response is less important than cellular interactions, which mainly involve T lymphocytes and phagocytes: this is known as cellular immunity. In fact, each phenomenon is necessary for the induction or amplification of the other. It is in fact the predominance of one of the two modes that determines the overall nature of the immunological response.
Cell-mediated cytotoxicity can vary according to the cells, targets, and effectors involved. Killer cells always link to their targets via MHC molecules (class I antigens), antibodies fixed to surface antigens, or directly to specific receptors. Cytotoxic cells secrete proteins that penetrate the target cells, triggering apoptosis and death. Perforin, a protein similar to complement fraction C9, creates pores in the target cell membrane in the presence of calcium. T cells use a proteoglycan to protect themselves from self-destruction by perforin.
Types of Hypersensitivity (Gell and Coombs Classification)
Hypersensitivity reactions are excessive or inappropriate immune responses that cause host tissue damage. Four types have been described: the first three are mediated by antibodies, while the fourth is mediated by T cells and macro-phages.
Type I Hypersensitivity (Fig. 2.22)
Now synonymous with allergy, type I hypersensitivity is due to activation, by a specific antigen, of sensitized mast cells coated with IgE. Allergy generally occurs on a genetically determined background that is mainly characterized by strong IgE synthesis, in a state known as atopy. The allergic reaction occurs in two phases: an early phase due to mast cell degranulation within minutes of exposure to the allergen; and a late phase occurring between 4-6 hours, involving eosinophils and macrophages. The frequently violent nature of type I hypersensitivity reactions is explained by the numerous and powerful mediators synthesized and released by mast cells and accessory cells. Allergic reactions can be investigated by means of skin tests (prick test or patch test), assays of specific and total IgE in serum or tears, and challenge tests.
Type II Hypersensitivity, or Antibody-Dependent Cytotoxicity (Fig. 2.23)
Type II hypersensitivity is a reaction directed against antigens to which antibodies (mainly IgG) have already bound. These antigens are generally borne on blood cells (red cells, leukocytes, or platelets) that are lysed after complement activation or directly by cytotoxic K lymphocytes via IgG carried by the target cells. Other cell types intervene subsequently, such as neutrophils, mast cells, and macrophages. Release of their soluble mediators can lead to severe “bystander” lesions. This mechanism is considered responsible for cytopenia induced by dietary antigens and drugs, as well as glomerulonephritis and myasthenia. The antigens can also be self-antigens. As regards chronic inflammatory disorders of the conjunctiva, type II hypersensitivity occurs in certain forms of autoimmune conjunctivitis such as ocular cicatricial pemphigoid, in which the antigen is an autoantibody-coated component of the BMZ.
Fig. 2.22 Type I hypersensitivity
Type III Hypersensitivity (Fig. 2.24)
Type III hypersensitivity involves IgG- or IgM-containing immune complexes, and complement activation. Several mechanisms can be involved. Chronic infection with persistence of the infectious agent stimulates a weak IgG response, with formation of immune complexes; an autoimmune disorder leads to continuous autoantibody formation, with immune complex deposition in tissues. Repeated contact with the exogenous antigen can also lead to chronic immune stimulation. Deposits of immune complexes in vessels or tissues sometimes cause intense inflammatory reactions with a risk of tissue necrosis. The conjunctiva can be the target in this type of hypersensitivity reaction, in patients with systemic vascular diseases associated with peripheral ulcerative keratitis or scleritis.
Type IV (Delayed-Type) Hypersensitivity (Fig. 2.25)
Type IV hypersensitivity is a cell-mediated reaction. It occurs after approximately 48-72 hours in the case of contact hypersensitivity or tuberculin hypersensitivity, and after a few weeks in the case ofgranulomatous reactions. The most typical form of contact allergy is eczema. Most of the culprit antigens are haptens. Haptens themselves are too small to be immunogenic, but they can cross the skin or mucosae and become immunogenic by combining with receptor proteins. Recognition of these complexes by T lymphocytes is specific for the hapten-carrier conjugate, and not for the hapten alone.
Initial sensitization takes about 10-14 days. The complexes are captured by APCs, including mainly cutaneous or mucosal Langerhans cells. These migrate via the efferent lymphatics to the nodes, where they present the complex to helper T lymphocytes phocytes in association with class II antigens. Memory cells are thus stimulated and trigger a more rapid reaction on subsequent contact. Contact hypersensitivity begins after 4-8 hours and becomes maximal after 48-72 hours. The tissue is edematous, infiltrated principally by CD4+ cells but also by CD8+ cells and Langerhans cells, and then by macrophages. Mast cells and basophils intervene secondarily. Many cytokines are secreted, some of which have a direct action on epithelial cells. In particular, gamma interferon stimulates the expression of human leukocyte antigen (HLA)-DR class II antigens and ICAM-1 on keratinocytes and conjunctival cells. These antigens favor the migration of immunocompetent cells, especially those expressing LFA-1 integrin (the ICAM-1 ligand).
Fig. 2.23 Type II hypersensitivity
Fig. 2.24 Type III hypersensitivity
Fig. 2.25 Type IV hypersensitivity
Tuberculin and granulomatous hypersensitivity reactions are characterized by the presence of large epithelioid cells, lymphocytes, and giant multinucleated cells (probably derived from macrophages). A central area of necrosis or fibrosis is often observed.
Local Strategies—Defensive Organization of the Conjunctiva and Cornea
The cornea and conjunctiva are permanently exposed to mechanical, toxic, and microbial insults from the outside environment. The eyelids and tears eliminate most irritant foreign bodies. When these mechanical barriers are breached, the corneo-conjunctival epithelium is the last obstacle to penetration into the deep ocular structures.
The Tear Film (see Fig. 1.10 and Chapter 9, Fig. G) (see Chapter 1 Focus 2)
The tears are the first line of defense of the ocular surface, concentrating chemical mediators released during local inflammatory reactions and thus playing a major role in conjunctival immunology.28. Normally, specific tear defenses are mainly ensured by secretory IgA (100-500 mg/L) produced by plasma cells in the lacrimal glands and conjunctiva. Associated in dimeric form with the secretory component, IgA carpets the ocular surface by binding to the sialic acid component of mucus. IgA thereby hinders bacterial adhesion and neutralizes certain toxins and viruses. Tears also contain a lower concentration of IgG (3-10 mg/L) and traces of IgM, IgE, prostaglandins, leukotrienes, interferons, and several complement fractions.
The protective properties of the tear film are mechanical (flushing of the ocular surface by reflex tearing), chemical (antibacterial lysozyme, lactoferrin, and betalysin), and trophic (growth factors, especially epidermal growth factor [EGF]). Lysozyme is a bacteriolytic enzyme that ruptures the membranes of sensitive microorganisms, in a similar manner to penicillin.29 It represents 30-40% of all tear proteins, but its concentration falls with age and during dry eye syndromes, facilitating infections. Lactoferrin, by complexing with iron, deprives bacteria of this crucial nutrient, and also activates NK lymphocyte functions. It thus plays a key, nonspecific antibacterial role. Mucus itself is a supplementary defensive component that englobes foreign particles, facilitating direct mechanical elimination via the conjunctival fornices.The mucin network is also thought to produce free radicals with bactericidal properties.30
A final nonspecific defense mechanism of the ocular surface is the normal microbial flora.28 Composed mainly of Staphylo-coccus epidermidis, Cory neb acterium, and Propionibacterium acnes, the commensal flora hinders the proliferation of more aggressive microorganisms. In some circumstances, when the microbial equilibrium is upset, saprophytic organisms can become pathogenic.
The Conjunctiva (Figs. 2.26 and 2.27)
The conjunctiva is both a mechanical obstacle and an immuno-logical barrier containing abundant immune cells ready to capture and destroy aggressors. These immune defenses exert their effects both in the epithelium and in the stroma.
Laboratory Investigations of Conjunctival Inflammation
1. Conjunctival Biopsy
Conjunctival biopsy is the method of choice for the diagnosis of certain inflammatory, autoimmune, and infectious disorders (especially chlamydial or mycobacterial infection), and tumors of the ocular surface. It also provides important material for research purposes, leading to important discoveries in the field of corneo-conjunctival biology,1,2 notably through immunohistochemical and molecular biology techniques, and conventional, confocal, or electron microscopy. However, not all patients accept conjunctival biopsy, which can be a supplementary tissue insult; furthermore specialized laboratory facilities are required.
Conjunctival biopsy is particularly useful for the diagnosis of autoimmune conjunctivitis such as ocular cicatricial pem-phigoid, epidermolysis bullosa acquisita, and bullous pem-phigoid.3,4,5 Immune deposits at the conjunctival basement membrane are detected by using polyclonal antibodies directed against human immunoglobulins and complement factors. Conjunctival biopsy also can help with the diagnosis of systemic vasculitis and granulomatous disease, when conjunctivitis is associated with peripheral ulcerative keratitis or scleritis.
2. Conjunctival Impression Cytology (Technique: see Focus 5)
Conjunctival impression cytology is a simple and noninvasive technique which allows study of the epithelial cytology.6 It is performed under topical anesthesia with opaque filters (e.g., Millipore or Gelman Supor, diameter 13 mm, pore size 0.2 µm) or transparent filters (e.g., Biopore). Contrary to conjunctival scraping, it provides a homogeneous cellular layer with an intact architecture and preserved intercellular junctions. The number of cells collected is far larger, and their morphology is better preserved for histopathological studies. Nevertheless, conjunctival impression cytology is no substitute for conjunctival biopsy, as the latter can yield information on the conjunctival architecture (epithelium and chorion) throughout its thickness. Conjunctival impression cytology is used to analyze the most superficial layers of the conjunctival epithelium, in which the three main cell populations are readily identifiable: epithelial cells, goblet cells, and Langerhans cells.7
Conjunctival impression cytology is generally used to investigate dry eye syndromes. It can show the degree of cellular morphological changes, which mainly consists of a reduction in the number of goblet cells, followed by a gradual reduction in the nuclear-cytoplasmic ratio of epithelial cells, and extensive keratinization.8 The use of this technique for purely morphological purposes, however, is limited to disorders with marked cellular alterations, i.e., essentially dry eye syndromes.
To obtain information on cell structure and function, immunocytological techniques are required to detect and/or quantify activation markers present on cell membranes or in the cytoplasm. This type of investigation is particularly useful in noninflammatory clinical settings such as dry eye syndromes resistant to substitutive therapy,7 or chronic treatment of glaucoma patients with multiple eyedrop preparations.9 In these situations, conjunctival impression cytology can reveal a subclinical inflammatory reaction that cannot otherwise be identified, but whose treatment is essential to relieve the patient’s symptoms. Dendritic cell counts which are increased during conjunctival inflammation, and especially the percentage of epithelial cells expressing HLA DR class II antigens (induced by secretory interferon gamma), are reliable and useful markers of local inflammatory status. Only dendritic cells normally express HLA DR antigens. However, during even very mild inflammation, some epithelial cells will also express this marker, probably as an accessory means of recruiting inflammatory cells to the ocular surface. Counts of epithelial cells expressing this marker provide precise information on the degree of local inflammation and are a preferred follow-up method, especially during treatment or on withdrawal of an irritant drug. Conjunctival impression cytology also can be used to assess degenerative and/or toxic modifications of the ocular surface, using markers of apoptosis, for example.
Finally, conjunctival impression cytology is useful for the detection of certain pathogens, such as chlamydiae and the herpes virus, using specific antibodies. Immunofluorescence is a simple and reliable diagnostic method, although gene amplification techniques, which are far more sensitive, especially for chlamydiae, are being developed for routine use.10
3. IgE Assay in Tears
There are two main tear collection techniques: the glass micropipette method (based on capillarity), often combined with stimulation of the nasal mucosa; and the strip of cellulose filter paper placed in the lower conjunctival fornix.11 These two methods do not seem to significantly modify levels of purely lacrimal proteins (lactoferrin, lysozyme, etc.), but levels of serum-derived proteins (including IgE) appear to be higher with the filter paper technique, possibly because of protein transudation provoked by the irritant paper strip.12 One study showed good agreement between the two techniques.13 Local production of lacrimal IgE has also been demonstrated.14 Immunological assays are based on anti-IgE monoclonal antibodies labeled with a radioactive marker (paper radioimmunosorbent test [PRIST]) or an enzyme (enzyme-linked immunosorbent assay [ELISA]).
Determining the level of tear IgE provides important diagnostic information, and elevated levels theoretically point to a type I hypersensitivity-mediated allergic disorder.15 High levels of tear IgE are typically associated with allergic manifestations such as hay fever conjunctivitis, vernal conjunctivitis, or atopic conjunctivitis, but tear IgE measurement in adults with chronic conjunctival disorders sometimes provides unexpected values and changes diagnostic orientation. In adults with clinically “allergic” chronic conjunctivitis, IgE levels are only significantly elevated in a relatively small proportion of cases (40-50%), while elevated levels are found in approximately one third of eyes with apparently nonallergic conditions. Symptoms including pruritus and seasonal reactivations are not always associated with high levels of tear IgE, and are thus poor markers of the underlying pathogenic mechanism in adults with chronic conjunctivitis. This finding is consistent with recent immunological studies which clearly show that IgE-dependent allergy can occur in nona-topic patients with an atypical chronology, and that symptoms such as pruritus are nonspecific.15a
In addition, the Th1 and Th2 lymphocyte systems possess a very interesting characteristic, namely that activation of one inhibits the other. When IgE is detected in tears, reflecting stimulation of the Th2 system, the Th1 activation pathway is normally inhibited. This latter phenomenon can be demonstrated with an indirect method that consists of analyzing class II antigen expression by conjunctival epithelial cells using conjunctival impression cytology. Class II antigen expression, which is a very sensitive marker of conjunctival inflammation, is dependent on interferon gamma secretion (Th1 system). There is a very strong negative correlation between the results of conjunctival impression cytology and tear IgE levels, with a virtual absence of class II antigen expression by the epithelium when IgE is present in tears, and an absence of IgE in the tears of patients with class II antigen expression.
This provides two relatively simple routine laboratory tests to distinguish between the two activation pathways and to identify patients with true, IgE-dependent allergy (in whom allergological investigations and specific therapy may be beneficial), and those with nonspecific, nonallergic inflammatory reactions (in whom such therapy is useless and may even be detrimental).
Nevertheless, it must be stressed that the absence of IgE in tears or blood does not rule out an allergic mechanism, as none of the available allergological tests is truly specific.
Other proteins can be assayed in tears, such as histamine, tryptase, IL-4, and specific IgE.8,16 Assay of eosinophil-derived neurotoxin (EDN), a protein present in eosinophils, is a good marker of eosinophil activation and degranulation. Although the presence of these mediators in tears is highly suggestive of allergy, it is not pathognomonic. Tear protein electro-phoresis can also be used to determine local inflammatory protein concentrations. All these assays require careful and nontraumatic tear collection, and are better done by laboratories accustomed to dealing with very small sample volumes.
4. Blood Protein and Circulating Autoantibody Profiles
The blood protein profile is based on a series of serum proteins with a variety of functions, regulation, molecular weights, half-lives, and tissue origins. Between eight and 12 serum proteins are used, generally including prealbumin, albumin, transferrin, haptoglobin, orosomucoid, complement fraction C3, and three immunoglobulins (IgA, IgM, and IgG). These proteins may play different roles according to the inflammatory process, and their resulting electrophoretic profile may help with the diagnosis and shed light on the patho-genesis of inflammatory disorders such as connective tissue diseases, sarcoidosis, and Sjögren syndrome.17 Furthermore, blood protein profile analysis can be helpful for the follow-up of patients treated for some inflammatory syndromes.
Also, specific blood test analysis can detect circulating autoantibodies consistent with the diagnosis of autoimmune diseases with conjunctival involvement such as Sjögren syndrome, systemic lupus erythematosus, and polyarteritis nodosa. In many cases, however, a low autoantibody level simply reflects nonspecific immune hyperreactivity.
References
3. Foster CS. Cicatricial pemphigoid. Trans Am Ophthalmol Soc 1986; 84: 527-663.
4. Mondino BJ. Cicatrical pemphigoid and erythema multiforme. Ophthalmology 1990; 97: 939-52.
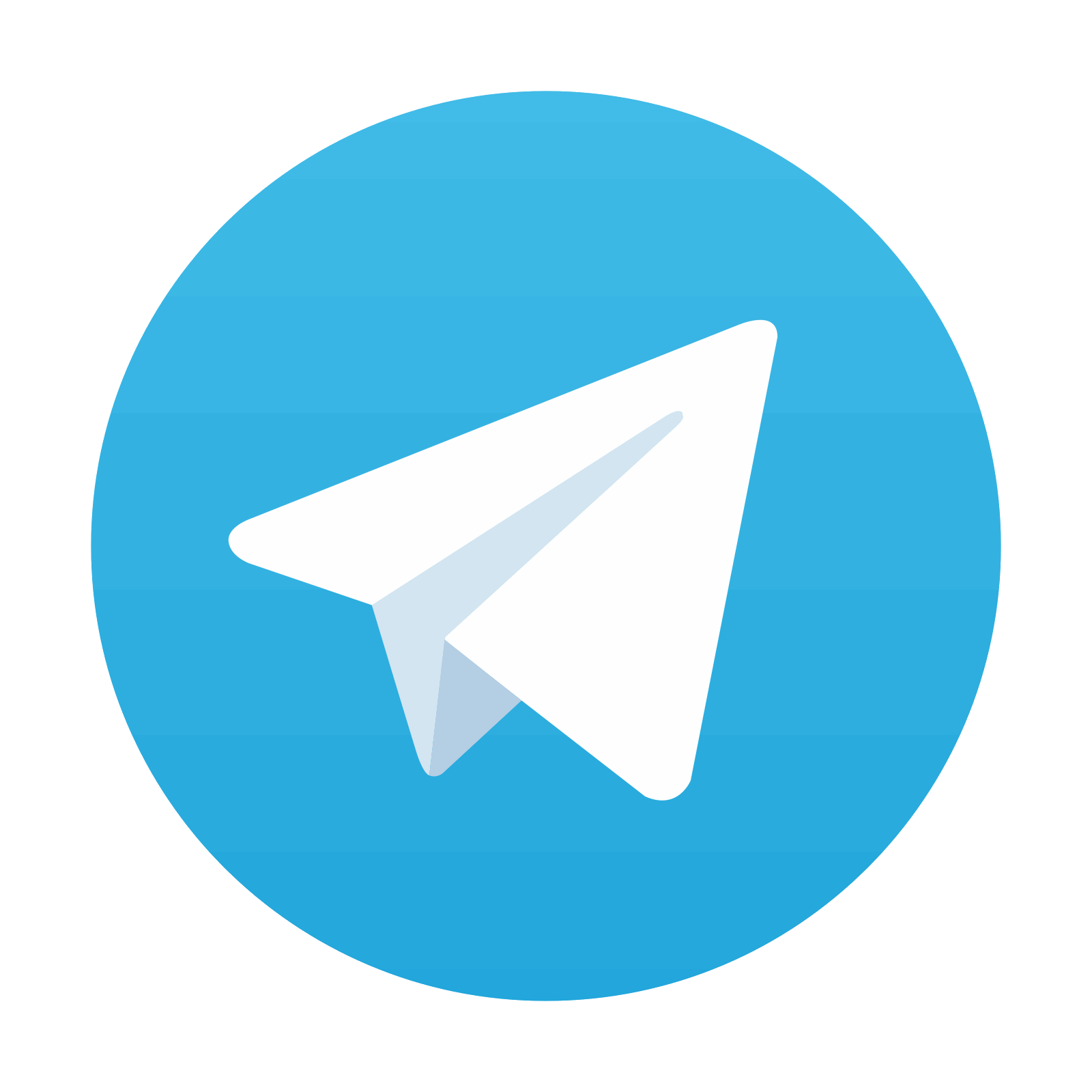
Stay updated, free articles. Join our Telegram channel

Full access? Get Clinical Tree
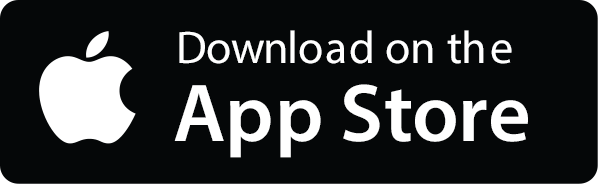
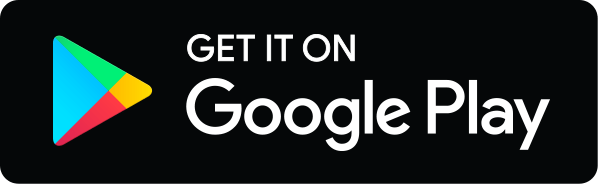