Fig. 82.1
Exemplary results of examination of a painting on canvas (Portrait of Sir John Wylie by P. Franck, N., Copernicus University, Toruń); (a, b) OCT tomograms: from the top, at least three layers of varnish (1) and then the glaze layer (2) are visible. Below, another thick glaze layer (3A, B, b) on top of the opaque paint layer (4) is seen. The depth scale bar is drawn for a medium of n R = 1.5
The OCT examination of varnish and glaze layers of paintings was, from the very beginning, considered an advantageous alternative to sampling. Despite the aforementioned limitations, OCT examination, due to its noninvasiveness and thus the possibility of being performed in as many places as desired, must be regarded as exhibiting improved representativeness [11]. It was early shown [14] that images obtained with OCT are congruent with cross-sectional photomicrographs of the sample taken in the same place after the OCT examination. Latour et al. used full-field OCT microscopy to analyze the structure of pigmented paint layer with superb resolution [15]. The morphology of varnish and glaze layers has been studied to attain a better understanding of the author’s technique, as well as of the present state of preservation and of previous alterations and restoration attempts. For instance, the ability of OCT to localize a certain paint layer in relation to the other strata was used to analyze the chronology of historical inscriptions in an eighteenth-century painting, some of which inscriptions were identified as secondary, added during past renovations [16]. By OCT examination, it was possible to link these inscriptions to events from the history of the person portrayed and consequently make properly informed decisions regarding the program for restoration of the painting. In the same account, it was shown that the signature in another painting examined is located between two layers of varnish, so that its execution probably does not coincide with the final stage of the artwork, suggesting forgery.
The ability of OCT to image the top layers of a painting has also been used as a direct aid to the restoration. Preliminary studies by Fontana et al. [16] and Liang et al. [17] revealed the possibility of using OCT to monitor the solvent cleaning of varnish. It has been shown that, with OCT, it is possible to monitor the thickness of the remaining layer of varnish [17] as well as the preservation of the surface of the paint layer underneath [18]. This subject has been further investigated by Iwanicka et al. [19, 20] reporting examples of selective overpaint and varnish removal from historic paintings.
In spite of the fact that the upper layers of paintings are the most popular subject of examinations, in some cases, it has also proved possible to use OCT for examination of underdrawing [11, 18, 21–23] – a preparatory layer often present under the paint layers, used by the artist for the preliminary design of the composition, sometimes not completely followed in the final result. These are very important features for art historians in studying the evolution of creation of the image. Underdrawings are usually examined with IR cameras, but in that case, the image is blurred by scattering of the illuminating light in the paint layers covering them. When OCT is employed instead, the signal is depth separated by the coherent detection and unwanted signal easily eliminated. The same mechanism can be used to sharpen the image of gold punch marks covered by a thick layer of lacquer [22].
In addition to analysis of the structure, OCT can be used for the tracking of certain destruction processes and thus aid in resolving the state of preservation of the artwork. Delamination of paint layers may be caused by poor adhesion between the layers and/or a mechanical impact. One of the possible causes of poor adhesion, common in the nineteenth century, is the use of canvas with commercial oil primer layers. If this support was used a long time after fabrication, it would have been extremely dry, and if covered with an oil paint layer, the final structure would have become very sensitive to delamination. A second factor – mechanical impact, apart from inflicting surface abrasion – may cause delamination penetrating beyond the perimeter of the area of visible destruction, under the layers in seemingly good condition, and thus be very difficult to detect. This was the case of the oil painting on canvas analyzed by the present authors a few years ago (Fig. 4 in [24]): the delamination was clearly seen to be extending out from the damaged area (varnish loss) and localized between the thick pigmented varnish layer and the glaze.
Similar damage is quite common for a particular painting technique called Hinterglasmalerei (reverse painting on glass) [25]. This modality, popular in the folk art of Central Europe (especially in the present south Germany, Poland, Slovakia, and Romania) until the mid-twentieth century, is characterized by employing a sheet of glass as both support and protection for the painting. Since it was designed to be viewed through glass, the details (contour and lights) were painted first, directly onto the glass, followed by glazes and opaque paints. The background layers were added at the end – in this way, the painting process was reversed as compared to classic painting technique. The obvious disadvantage of this method of painting is poor adhesion of the paint to the glass. Thus, extensive delamination is a common destructive phenomenon in these objects. Moreover, the detachments are difficult to examine and evaluated physically, because they are isolated from both sides by being sandwiched between the glass and the paint layer. OCT is here an obvious method of choice because it penetrates well through glass and, due to the high refractive index gradient in such places, is very sensitive to delaminations and blisters [26, 27].
In Fig. 82.2, an exemplary tomogram from a reverse painting on glass is presented, together with a graphic model of the structure. The whole volume of the glass support (6) is visible here, with the probing beam penetrating from the right-hand side (through the glass). Paint layers of different absorbing properties (1, 2, 3) are discernible behind the glass sheet. The adhesion of these layers to the support may be established instantly: in the upper part of the tomogram, the high intensity of light scattered at the inner surface of the glass indicates the existence merely of a delamination, whereas in the lower part, there is a fully developed blister (5B), partially filled with a consolidation adhesive (4). In the past, there had been some attempts to repair the damage with use of consolidant applied from the back, which was supposed to penetrate through all of the paint layers to eventually fill the voids between glass and paint. As could be detected by OCT, this treatment was not always successful. Moreover, as discovered at other locations in the same object, the consolidant itself had deteriorated with time, losing adhesion to the glass and developing tent-shaped deformations (Fig. 4D in [27]).
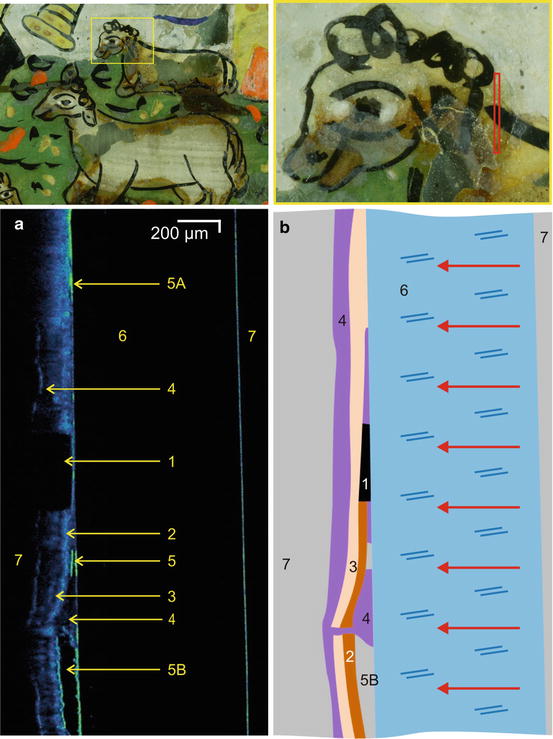
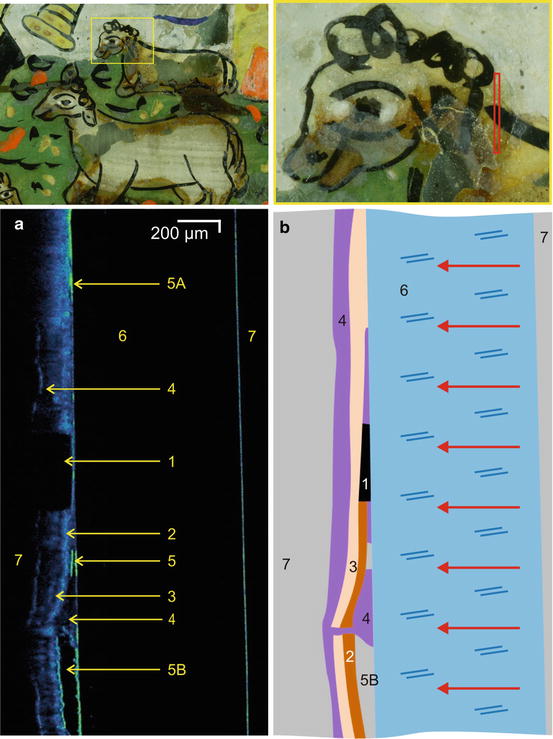
Fig. 82.2
Results of the OCT examination of the reverse painting on glass St. Wendelin, nineteenth century, Ethnographic Museum in Toruń, Poland, oil on glass (fragment); (a) tomogram obtained in the region indicated by the red-outlined bar in the macrophotograph above. The depth scale bar is drawn for a medium of n R = 1.5; (b) graphic model of the structure of the painting derived from the OCT data; (1) contour (nontransparent paint layer), (2) glaze, (3) semitransparent paint layer, (4) consolidation adhesive, (5A) early detachment of paint layer, (5B) developed detachment of paint layer, (6) glass, and (7) air (Photography: W. Grzesik)
In addition to examining the state of preservation, OCT was also used to monitor the consolidation process [19, 28]. In particular, the wetting of glass by consolidant can conveniently be monitored by OCT in real time.
Besides the practical applications of OCT in the examination of paintings, more fundamental studies – on the drying processes of varnishes – were conducted by Liang et al. [21] and continued by Lawman and Liang with combined use of NMR [29] and multi-interface OCT profilometry [30]. In this specific case of a transparent layer of varnish on a glass substrate at both interfaces, the refractive index change is significant and is described by a step function. The OCT A-scan can be approximated by a point-spread function (PSF) in this place. By locally fitting a Gaussian function, the authors were able to localize the interface to a precision (rms = 55 nm) two orders of magnitude better than the axial resolution of their instrument (6.5 μm), but only when both interfaces were far enough from each other. In these, and similar studies by Targowski et al. [31], it was confirmed that the ability of varnish to follow the roughness of the paint surface depends mostly on its molecular weight, in accordance with earlier results by de la Rie [13].
82.2.2 Application of OCT to Examination of Historic Glass
There is no doubt that OCT is well suited to the examination of glass objects, since this material is generally transparent. Thus, its internal structure can usually be examined within the whole depth of imaging of the OCT system employed. If the glass object contains structures of different scattering properties, they can be precisely traced with OCT [5, 18, 32, 33].
Apart from examination of structure for inventory purposes, some destructive processes in historic glass may be analyzed with OCT. In the case of stained glass, the advance of atmospheric corrosion [34] is the most important evaluation to carry out in the determination of its state of preservation. This process, caused by the moisture from precipitation at the outer side and condensation at the inner side of the glass sheet, essentially comprises two steps. During the first (called leaching), alkali and alkaline-earth ions are rinsed from the glass and replaced by H+ or H3O+ ions. As a result, a gel layer rich in silica is formed on the surface of the glass. During the second step, water penetrates into this layer, and the glass may be destroyed both chemically and mechanically by freezing if subjected to low enough temperatures. This silica-rich layer may have one of several different optical properties, depending on the glass composition. Usually it has a porous structure and thus a high-scattering coefficient, although sometimes it is perfectly transparent, but of different refractive index. In both cases, it can be detected with OCT [18, 33, 35]. By contrast with the standard examination technique, SEM–BSE (Scanning Electron Microscopy–Backscattered Electrons), using polished cross sections, no prior preparation of the glass is necessary. In principle, with a portable instrument, it should be possible to examine stained glass without dismounting it from the windows. It is worthwhile noting that the detection of the depth range of the gel layer is usually obstructed by multiple scattering of the OCT probing light within this structure, which is seen as fading tails in the A-scan signal. This disadvantage may be overcome if the other side of the glass is accessible and has not deteriorated: examination through the transparent glass then reveals sharp boundaries to the gel layer [27].
Another kind of historic glass destruction is called crizzling. This is caused by imbalance in the ingredients during their preparation and demonstrates itself as a network of fine cracks at one or both surfaces of the glass piece. When the object is stored under inappropriate environmental conditions, these lesions may develop with time and can eventually lead to the total destruction of the object. The OCT examination of such an artifact [27] revealed that the depth of the cracks on a given side of the glass sheet is uniform but different from that on the other side.
82.2.3 Application of OCT to Examination of Craftsmanship (Musical Instruments, Gemstones, Ceramics)
Lacquer coating on musical instruments is a layer of similar properties to those of varnish on paintings. In both cases, high axial resolution is a decisive factor for successful OCT imaging. Since these coatings are always transparent to visible light, the necessary resolution may well be achieved using OCT with probing light in the visible rather than the infrared range of wavelengths. Latour et al. [36] used red light (λcenter = 650 nm, Δλ = 150 nm) in a full-field time-domain OCT system equipped with a Mirau objective. They presented results of examination of lacquer coating an eighteenth-century Italian violin, preceded by laboratory tests on model samples of flame maple and lacquered wood. They showed, for the first time, evidence of the ability of OCT to reveal the internal structure of the wood (up to 50 μm of optical depth), but some of the conclusions concerning the structure of the coatings on the violin were ambiguous. Another application, encompassing the examination of both painting and craftsmanship, is seen in the imaging of painted enamel on a ceramic tile [18].
Jade art objects of art have been a focus of OCT examination from the very beginning right through to the present. In 2004, Yang et al. [8], using objects from the National Museum of Taiwan, proved that OCT may be used to detect forged archaeological jades, since this semitransparent stone scatters the probing light differently when it has been aged artificially. Natural ageing is a very slow process as compared with the artificial one which is induced by extensive heating. As a result of the latter, counterfeit objects have a more heterogeneous structure which is possible to differentiate from the more homogeneous, naturally aged one by OCT examination. Similar examples have been reported by Liang et al. [18]. This qualitative conclusion was developed quantitatively by Chang et al. [37], who defined a statistical measure of texture of the OCT image as being a vector in a 6D space composed of three parameters derived from the histogram of the image and three calculated directly from the pixel values of the B-scan. The length of this vector, together with the second parameter representing the translucency of the object, may be used for characterization of various jades, including the detection of fraud. In parallel with this holistic study, details of the structure of jades, in particular the morphology of cracks, have been studied by Yang [38].
Another sort of object for which the use of OCT for examination was noticed early on [9] is the glaze layer on ceramics. Those studies have been continued by other authors [18, 33], focusing on analysis of different phases (and phase boundaries) within the glaze layer [39, 40], the presence and size of air bubbles [40], and the morphology of cracks [38]. The aim of these efforts was to provide a tool useful for identification of the origin, method of production, and thus, in some cases again, recognition of fraud. In the specific case of Egyptian faience, it is possible to distinguish between three ancient production methods by analyzing their cross sections with SEM. In their recent paper, Liang et al. [41] showed that this is also possible noninvasively with OCT operating at 930 nm.
Some attention has also been given to examination of parchment with OCT in the context of its degradation caused by the presence of iron-gall ink. After application, this kind of ink undergoes chemical processes resulting in its change from almost colorless through blue to a final dark brown tint. Simultaneously, acidic components of the ink cause degradation of the support which, in some cases, may eventually lead to the total destruction of the document. Conveniently, radiation of 830 nm is practically not absorbed by this ink. OCT examination is therefore possible also for areas of support covered by the ink and thus most exposed to this degradation factor. Such a study was performed by Góra et al. [42] with utilization of standard as well as polarization-sensitive (PSOCT) techniques. It was shown that OCT of this wavelength is able to image the subsurface structure of parchment with the iron-gall ink thereon, and some evidence of degradation of the parchment was found.
82.2.4 Laser Ablation Control by OCT
Laser ablation of solids is a well-established technique and may be precisely controlled by pulse duration and energy. Therefore, it is used as a fine tool also for the examination and restoration of art objects. In this section, two applications of OCT as a monitoring device of the laser ablation process will be presented. There is no doubt that in both cases, these specific utilizations can be considered only as examples from much wider field of application.
82.2.4.1 Absolute Depth Profiling with LIBS and OCT
Laser-induced breakdown spectroscopy (LIBS, or sometimes LIPS) is a technique of elemental composition analysis by spectral examination of emission from a small plume of plasma generated by the laser pulse. It is mostly used for determination of the composition of metal alloys. The diameter of the crater is usually less than 200 μm, and thus, this technique may be considered as micro-destructive. It is therefore usually regarded as an acceptable method for examination of art objects [43]. Its modality, called LIBS stratigraphy, is especially useful: a tray of pulses is delivered to the same spot on the object, and the ablation crater is created gradually. After each pulse, the plasma spectrum is registered and the intensity of chosen spectral lines determined (Fig. 82.3a). In this way, a depth profile of concentration of a given element may be revealed and eventually associated with a particular layer of the structure examined. A significant drawback of this approach lies in the lack of information about the exact depth of the crater after each pulse. Moreover, for multilayer objects, the ablation rate usually varies from stratum to stratum, and the dependence of depth on the pulse number is not linear. Therefore, for true depth calibration of the concentration profiles, the depth of the crater should be determined noninvasively after each laser pulse. The idea of using OCT for this purpose comes from the group of Sabsabi but, since the US patent [44] and a paper by Dufour et al. [45], has not been further pursued. A similar experiment was previously described by Papazoglou et al. [46] who employed a white light interferometer, similar in principle to a full-field TdOCT system. Amaral et al. [47] have analyzed some historic Brazilian coins using a commercial TdOCT instrument to determine the final depth of the crater after applying the whole train of pulses. To estimate the depth of the crater after each pulse, they assumed a constant ablation rate throughout the whole experiment. Although for metal alloys this seems acceptable, the present authors have shown that this is definitely not the case for paint layers (Fig. 82.3b). In these experiments, performed both on model samples [48] and a historic painting [49], we used spectral-domain OCT instrument after each laser pulse as a fast profilometer. In this way, the combined LIBS/OCT experiment could be performed in a reasonable time and the true depth profile recovered (Fig. 82.3c). Moreover, from changes in ablation rates, the locations of interfaces between strata were determined and confirmed by the analysis of OCT B-scans obtained in the same place. Obviously, such confirmation was possible only within semitransparent strata, whereas the crater depth and ablation rate determinations from the surface profile were feasible throughout. In Fig. 82.3, the first boundary (I/II) can be determined from the OCT B-scan and identified as lying between two layers of varnish, whereas the second boundary (II/III) is clearly visible as a change of the ablation rate (slope of the data in Fig. 82.3b) and is identified from the OCT B-scan as the varnish/opaque paint interface. The third boundary is not visible in OCT imaging as lying within the opaque structure, but can be identified clearly by the increase in strontium concentration. It is worthwhile noting that information about elemental concentration is important for both curators and conservators, as it helps in understanding the painter’s technique, in distinguishing between original and non-original areas in the painting, and sometimes in the dating of the artwork.
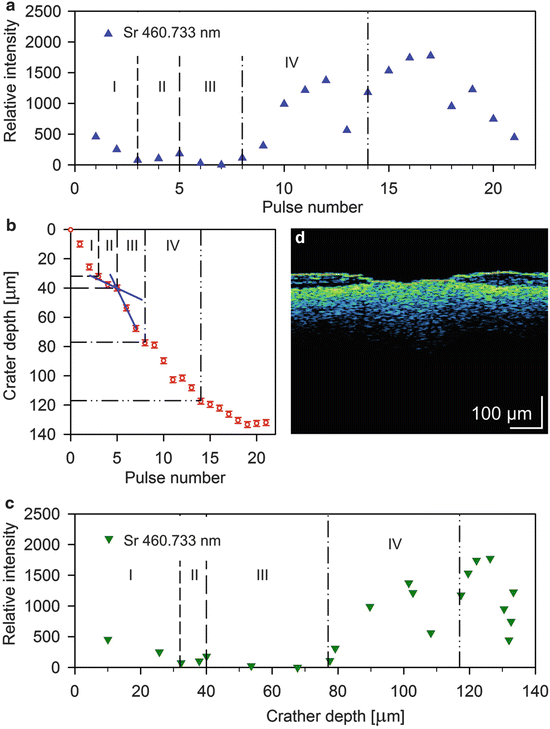
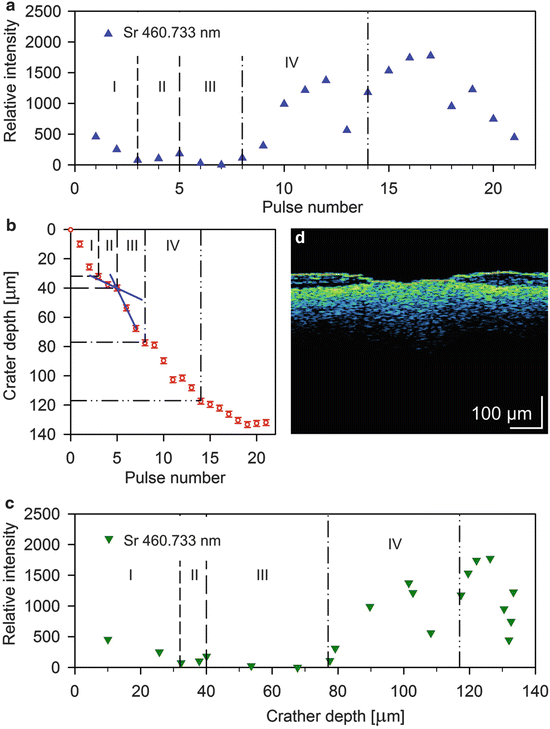
Fig. 82.3
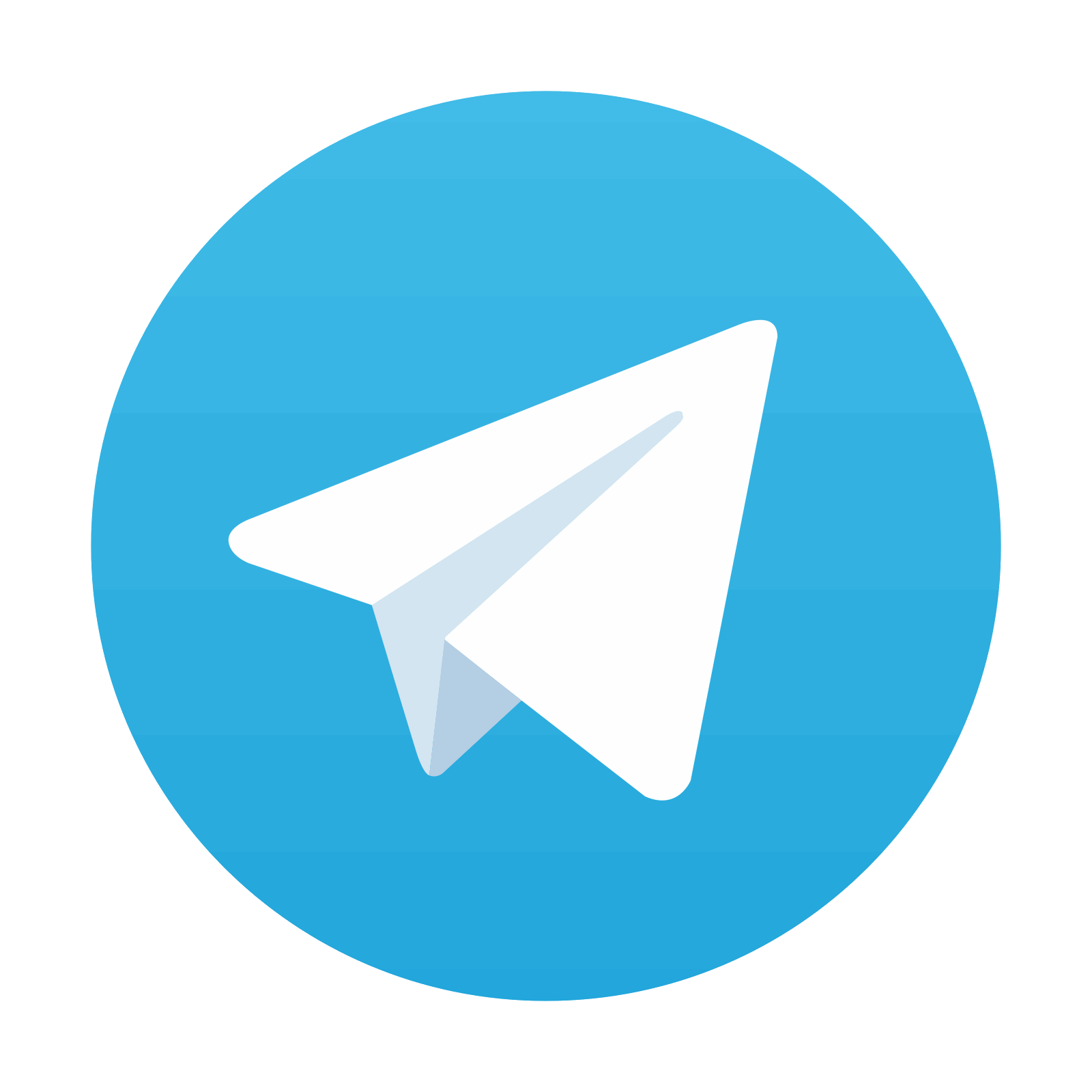
Exemplary result obtained with an LIBS/OCT instrument (Data from [49]); (a) depth profile of strontium obtained by LIBS stratigraphy at line 460.733 nm; (b) crater depth determined from OCT data – evidence for strata boundary at 40 μm from the change of ablation rate is marked (see text for description of strata I to IV); (c) true depth profile determined from LIBC/OCT data; (d) OCT cross section after the 4th pulse; the vertical scale bar is drawn for a medium of n R = 1.5
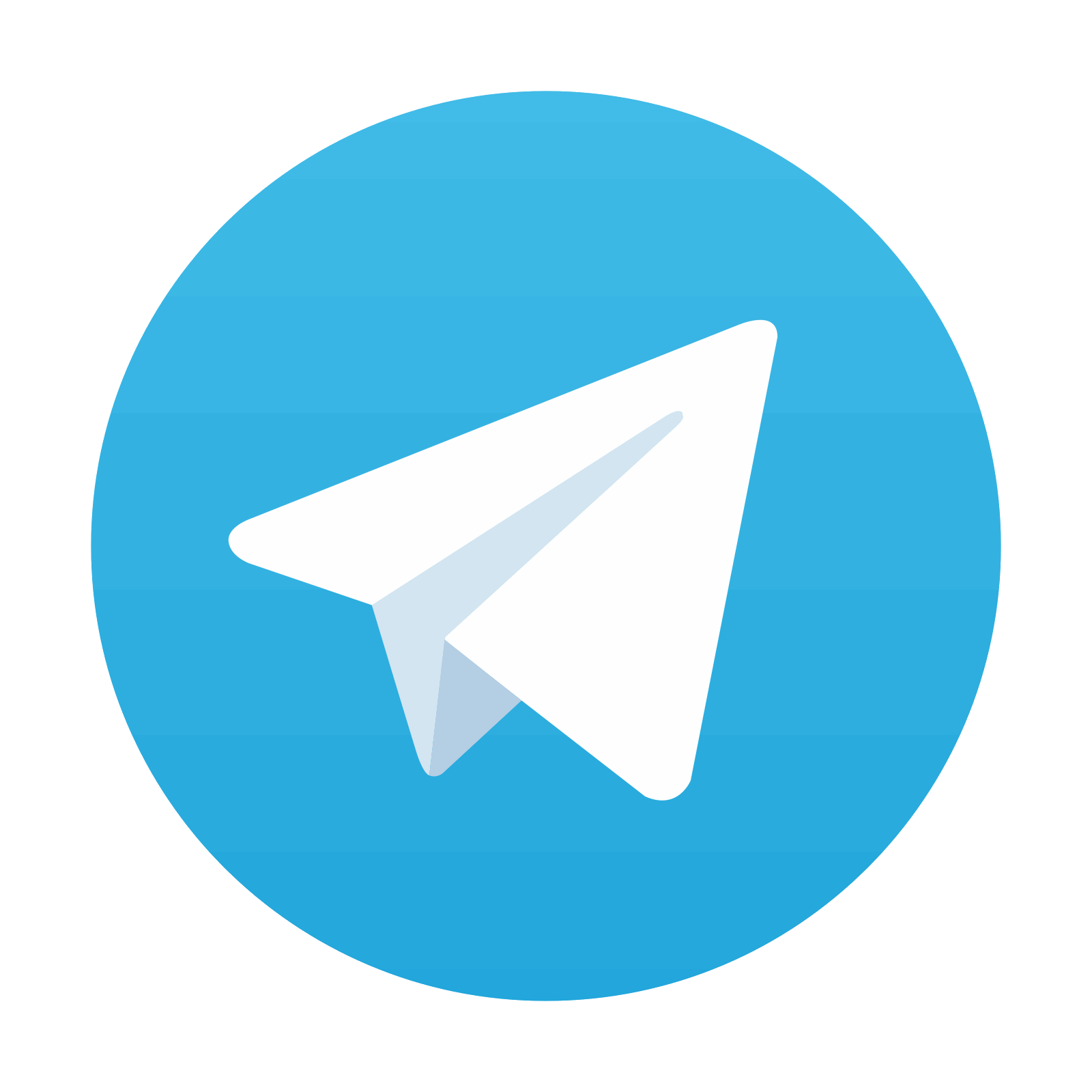
Stay updated, free articles. Join our Telegram channel

Full access? Get Clinical Tree
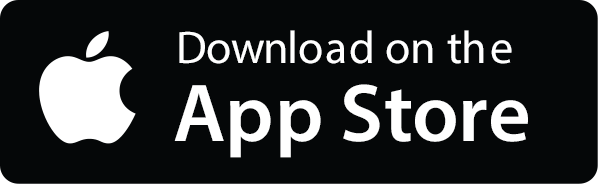
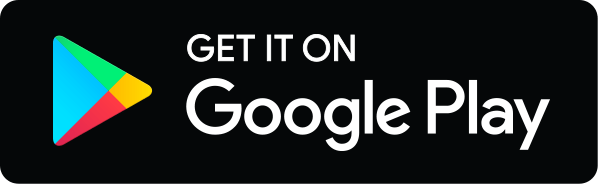
