Figure 25.1 A simplified schematic of the complement cascade. The sites of action of therapies being investigated are included.
All pathways form a C3 convertase, an enzyme that cleaves and activates C3 into C3a and C3b. C3b activates the C5 convertase, which cleaves C5 into C5a and C5b. C5b combines with C6-9, forming the terminal MAC, which causes lysis of cellular membranes and is deadly to cells and pathogens. C3a and C5a are anaphylatoxins that increase vascular permeability, cause degranulation of mast cells and neutrophils, induce cytokine release, and mediate leukocyte chemotaxis (54).
Complement and AMD
Several studies have shown that complement components may be deposited in drusen, including C3, C3a, C5a (55), and C5, the MAC (56), CFH, and complement receptor 1 (57,58). C1q and C3 have been shown to occur in CNV (9), and C3a and C5a increase VEGF production (55).
Genetic polymorphisms in complement encoding genes are associated with a predisposition for AMD. Complement factor B (CFB) and complement factor 2 genes have been associated with AMD, and genetic variations may be protective (59), as the production of the classical and alternative C3 convertases are diminished (60). Variations in the C3 gene are associated with AMD (61), as is the CFH gene; the most frequently reported factor implicated (62).
Complement Component 3
C3 is important for all three pathways, and animal and in vitro studies have demonstrated its importance in AMD. Subretinal injections of C3 in mice develop signs of AMD (63), while C3 knockout mice are protected from laser-induced CNV (64). C3a leads to increased VEGF production by RPE cells in vitro (55), and C3 has been demonstrated in surgically removed CNV (9). Plasma C3 levels appear to be elevated in patients with AMD (65).
POT-4/AL-78898A is a derivative of compstatin, a peptide inhibitor of C3 activation. Compstatin has been shown to decrease drusen formation in monkeys with early-onset macular degeneration (66). In phase I studies, POT-4 was demonstrated to be safe when injected intravitreally (67). AL-78898A is currently in a phase II randomized, double-blinded, crossover study in patients with exudative AMD designed to demonstrate a biologic effect after one intravitreal injection (68). Outcomes include time to escape to standard of care therapy, change in retinal thickness and VA.
Complement Component 5
Complement factor 5 is required for formation of the MAC. In humans, C5 is found in drusen (55), and serum levels of C5a are elevated in patients with AMD (69). C5a may increase RPE-mediated VEGF secretion (55). Mouse knockouts for the C5a receptor display reduced VEGF production and CNV formation in laser-induced models of CNV. There have not been clear associations between the C5 gene polymorphisms and AMD.
Eculizumab is a humanized monoclonal antibody against C5. It is given intravenously for paroxysmal nocturnal hemoglobinuria, another complement-mediated disorder (70). Chronic and systemic use increases the risk of Neisseria meningitides infection. Eculizumab is being tested in a phase II, randomized, placebo-controlled, double-masked study for dry AMD (71). After receiving a meningococcal vaccine, patients are randomized to eculizumab or placebo for 24 weeks. Patients are followed for 12 months after the initial infusion, and outcome measures include changes in the size of GA, drusen volume, and VA.
ARC1905 is an anti-C5 aptamer that blocks the cleavage of C5 into C5a and C5b, thus blocking further complement activation (52). Aptamers are nonimmunogenic, single-stranded oligonucleotides that display three-dimensional folding characteristics that allow inhibition of protein–protein interactions (72). A phase I study evaluating intravitreal ARC1905 in combination with intravitreal ranibizumab for exudative AMD has been completed (73). Results have not been released. Another phase I trial is ongoing to investigate safety and tolerability of intravitreal ARC1905 in the treatment of dry AMD with drusen and/or GA (74).
JPE1375 is a small molecule peptidomimetic antagonist against the C5 receptor. This compound exhibits high stability and receptor specificity. In mice, it has been demonstrated to have activity in an immune complex–mediated peritonitis model (75).
Complement Factor D
CFD is required for the activation of the alternative pathway C3 convertase. CFD knockout mice showed a significant reduction in photoreceptor damage after being exposed to constant light for several days (76). Genetic alterations in the CFD gene may (77) or may not be associated with AMD (78). There are conflicting data on whether or not systemic CFD is elevated in AMD patients (5,69,77). Nonetheless, there still may be a therapeutic role for lowering CFD and inhibiting the alternative pathway C3 convertase.
FCFD4514S is a monoclonal antibody against CFD. A phase I study assessing the safety and tolerability, dosing, and pharmacokinetics of intravitreally delivered FCFD4514S is ongoing (79). A phase II, randomized, sham injection controlled study of safety, tolerability, and efficacy of intravitreal FCFD4514S in patients with GA is also ongoing (80). The main outcome measure is growth of GA at 12 months.
TNX-234 is a complement inhibitor that may be a therapeutic option for AMD. It is a humanized antibody against CFD (81).
Complement Factor B
CFB is a component of the alternative pathway C3 convertase (5). CFB has been localized in the neurosensory retina, in the RPE, in the choroid, and in drusen (59). In mice, its expression increases with age, and this was associated with increased C3 in the RPE and Bruch’s membrane (82). Variations in the CFB gene are associated with AMD (59) and confer a protective effect through the reduction of alternative pathway C3 convertase. In vitro studies have shown a fourfold reduction in C3b binding affinity in certain variants, and this was associated with a reduction in C3 convertase formation (60).
TA106 is a Fab fragment of an anti-CFB antibody (52). Intraocular injections of anti–factor B antibodies appear to decrease light-induced photoreceptor loss (83). It is currently involved in preclinical testing.
Complement Factor H
CFH regulates the alternative pathway by promoting the breakdown of its C3 convertase. In vivo studies have shown that CFH is produced in the RPE and accumulates in drusen, RPE cells, choroid, and extracellular space (58). CFH knockout mice demonstrate increased autofluorescent material and C3 deposition in the retina (84). Subretinal injection of RNA directed against CFH showed increased MAC deposition and laser-induced CNV in mice (85). Genetic studies have revealed that polymorphisms in the CFH gene increase the development and progression of AMD. A substitution of tyrosine by histidine at the 402 position (Y402H) is the most reported polymorphism (62), but other variants also exist (86). High-risk CFH polymorphisms are additive with smoking (87) and may be associated with systemic complement activation (88).
Molecules resembling CFH or with a similar function represent a possible therapy for AMD. Animal models and in vitro studies have shown that RPE deterioration and CNV development are inhibited by CR2-fH, a recombinant protein consisting of CFH linked to a factor 2 targeting fragment that binds to activated complement products (89). Other recombinant CFH proteins have been developed (83,90) and may represent future therapies.
Other Therapies
CD59 is a natural membrane-bound inhibitor of MAC formation. In a murine model, subretinal delivery of an adenovirus vector expressing human CD59 can attenuate the formation of laser-induced CNV (91). Properdin stabilizes the C3 convertase and may also actively induce its formation on foreign surfaces (92). Antiproperdin antibodies have been developed that would destabilize the C3 convertase and may inhibit the complement system. C1-INH (SERPING1) is a protein that inhibits the classical pathway and has been shown to be associated with AMD (93). C1-INH has been used systemically in a complement disorder, hereditary angioneurotic edema, but has not been tested in AMD. Complement receptor type 1 has specificity for C3b and C4b (94). It inhibits the classical and alternative pathways. A form of the receptor has been developed, the soluble complement receptor type 1 (sCR1).
AMYLOID
The extracellular accumulation of proteinaceous deposits has been implicated in several age-related conditions, including atherosclerosis, skin elastosis, AD (12), and AMD. Proteins include immunomodulators, inflammatory mediators, acute phase reactants, complement components, and immunoglobulins, highlighting a common chronic and localized inflammatory component in these disorders (95). One well-established protein in the brain is Aβ, a peptide that is produced by the cleavage of amyloid precursor protein (APP) by the β-site APP-cleaving enzyme and a presenilin complex (96). Aβ is a physiologic peptide that is synthesized but quickly degraded by peptidases such as neprilysin (97).
AD is the most common form of dementia and is the sixth leading cause of death in the United States (98). It is characterized by the extracellular deposition of amyloid plaques and neurofibrillary tangles. The accumulation and aggregation of Aβ form β-pleated sheets that form the insoluble amyloid plaques and cerebrovascular deposits in the brain of AD patients (99). Aβ is neurotoxic, as its accumulation leads to microglial and astrocyte activation and migration to the plaques (100). The microglia engulf Aβ and release cytokines, ultimately leading to neuronal apoptosis.
Amyloid Deposition in AMD
Studies have shown that Aβ is deposited in drusen (7,101). Dentchev et al. (101) found four of nine postmortem AMD human eyes to have Aβ-containing vesicles, as opposed to none in normal eyes. In postmortem eyes, the RPE was the source of APP, the precursor to Aβ (7). In mouse models for AD, RPE degeneration occurred with an accumulation of sub-RPE deposits (102). Injections of Aβ into the vitreous of rats have been shown to induce apoptosis of the outer and inner nuclear layers (103). With age, a diseased and thickened Bruch’s membrane may impair clearance of Aβ into the choroid (99).
Aβ may also have a role in the progression of AMD. Dentchev et al. (101) showed that the highest quantity of Aβ-containing vesicles occurred at the borders of GA. Aβ was not present in the center of GA or within a disciform scar. Aβ is most prevalent in eyes with moderate-to-high amounts of drusen and may be associated with advanced AMD (12). Aβ may also have a role in exudative AMD. Cultured human RPE cells and mice disrupted for the neprilysin gene had an up-regulation of VEGF and a down-regulation of pigment epithelium–derived factor, an antiangiogenic protein (102). Furthermore, studies have shown that the high temperature requirement factor A1 (HTRA1) gene encodes a protein that is directly involved in the Aβ pathway, and it colocalizes with these deposits in the brain (104). Genetic polymorphisms in the HTRA1 gene have been linked with a higher risk of AMD (105).
Though AMD and AD share common features, the ultrastructural characteristics and localization of in the two are not the same (12). In AD, Aβ is deposited mostly around major retinal vessels in the inner retina, similar to that seen in amyloid angiopathy of the brain (99). While one prospective study identified an increased risk of developing AD in patients with advanced AMD (106), other studies have shown no significant association between AD and early AMD (107). Thus, there are clearly differences between the two, and medications targeting one may not necessarily be useful for the other.
Complement and Amyloid
Amyloid deposits have been shown to activate the complement system in both AD and AMD (108,109). In AD, Aβ directly and independently activate both the classical and alternative complement pathways through C3, C5, and MAC (108,110). CFH polymorphisms (Y402H) have also been shown to be overrepresented in AD in some studies (111), but not in others (112). Drusen may form, in part, due to activation of the complement system by Aβ. Aβ colocalizes with activation-specific fragments of C3 (12). A main activator of the alternative pathway, CFB, was shown to be indirectly up-regulated by Aβ in the presence of microglia and macrophages (111). C3b is inactivated by factors H and I, but factor I has been shown to be inhibited by the presence of Aβ, resulting in unregulated complement activation (109).
Amyloid-Based Therapies
Copaxone (glatiramer acetate) is approved for relapse prevention in MS. It is thought to modify the immune process that is responsible for MS, but its full mechanism is unknown. It is neuroprotective in mice models of AD, reducing amyloid plaques and prolonging neuronal survival (113,114). Landa et al. (113) investigated the use of Copaxone to reduce drusen number. Patients with intermediate dry AMD were enrolled in a prospective, randomized, double-blinded, placebo-controlled trial. They received weekly subcutaneous Copaxone or placebo for 12 weeks. Four treated patients (eight eyes) showed a decrease in total drusen area, as compared to two control patients (four eyes) who displayed a slight increase. Another prospective study of patients with dry AMD received weekly subcutaneous Copaxone or sham injections for 12 weeks (114). Seven patients (14 eyes) were treated with Copaxone, and seven patients (12 eyes) received sham treatment. Copaxone-treated patients exhibited a disappearance/shrinkage of 19.2% of drusen, as opposed to 6.5% in the sham group (P = 0.13). No current clinical trials are registered investigating the use of Copaxone for AMD.
RN6G is an antibody to Aβ that targets the C-terminus of the Aβ peptides Aβ40 and Aβ42. In a mouse model of AMD, it improved the ERG response, reduced levels of Aβ and complement components in sub-RPE deposits, and preserved the RPE (115). A phase I, double-masked, placebo-controlled study was performed to evaluate its safety, tolerability, and dosing for dry AMD (116). Patients were randomized to an intravenous single dose of RN6G or placebo. Results have not been published. A second phase I double-masked study is under way to evaluate multiple doses of intravenous RN6G versus placebo (117).
GSK-933776 is a monoclonal antibody against Aβ that is involved in a phase II study designed to evaluate its effect on GA after intravenous administration (118). Patients are being recruited for this randomized, double-masked, placebo-controlled study, and estimated enrollment is 162. The primary outcome is the rate of change of GA over 12 and 18 months.
ANGIOGENESIS
Angiogenesis inhibitors have drastically changed the treatment and prognosis of exudative AMD. VEGF is a protein that regulates vasculogenesis and angiogenesis, is proinflammatory, and induces vascular permeability (1). Its expression is up-regulated by several growth factors, including fibroblast growth factor (FGF), platelet-derived growth factor (PDGF), insulin-like growth factor, and others. The VEGF gene family includes VEGF-A, -B, -C, -D, and placental growth factor (PlGF). VEGF-A appears to be the most associated with angiogenesis (1), while PlGF is the mostly expressed VEGF protein in endothelium (119). PDGF-β is another factor implicated in angiogenesis, as it stimulates pericytes, which support the endothelium (120).
Receptor tyrosine kinases (TKs) have been increasingly recognized as being important in the angiogenesis pathway. Three VEGF receptors exist with intrinsic TK activity (VEGFR-1, 2 and 3), and VEGFR-2 appears to be the primary mediator of angiogenesis and vasculogenesis (121). Other TK-based receptors may contribute to angiogenesis, including the PDGF receptor (CD140) and FGF receptors α and β (122). Other receptor types have also been implicated in angiogenesis and targeted in studies; these include the nicotinic acetylcholine receptor (nAChR) (123), integrin α5β1 receptors (124), and sphingosine-1-phosphate (S1P) (125).
Bevasiranib
Bevasiranib is a small interfering RNA (siRNA) that acts by inducing breakdown of the VEGF messenger RNA (mRNA) (1). It was developed to match the protein-encoding nucleotide sequence of the mRNA to prevent translation into the protein. It does not target the VEGF protein itself. SiRNA directed against VEGF inhibited and regressed ocular neovascularization in mouse and primate models (126,127). After undergoing phase I and II studies, intravitreal bevasiranib was evaluated in a phase III study to assess its efficacy in exudative AMD after three loading doses of ranibizumab (128). Ranibizumab given every 4 weeks served as the control. Outcomes at 60 weeks showed that visual results were inferior to the ranibizumab arm. The study was terminated early, as the study was unlikely to meet its primary end point.
E10030
One mechanism of resistance to anti-VEGF therapy involves the ability of pericytes to protect endothelial cells from VEGF inhibition. PDGF-β stimulates pericytes and allows their integration into the vessel wall (120). Several lines of evidence support targeting PDGF and VEGF to enhance efficacy. Coinhibition of PDGF-β and VEGF-A is more effective in inhibiting mouse models of ocular neovascularization than anti-VEGF therapy alone (129). E10030 is an aptamer directed against PDGF-β that decreases pericyte density in CNV. Phase I studies confirmed safety, dosing, and tolerability (130). Twelve-week results of 22 patients treated with combined intravitreal E10030 and ranibizumab yielded an improvement of three lines in 59% and an 86% mean decrease in CNV size (131). E10030 is involved in a phase II clinical trial to assess safety and efficacy in exudative AMD (132). Patients are randomized to one of two doses of intravitreal E10030 or sham. All groups receive monthly ranibizumab. Outcomes include proportion of patients gaining 15 letters, change in VA, CNV area, and central subfield thickness and adverse events. Visual outcomes are measured at 12 weeks, and adverse events are monitored for 24 weeks.
Pazopanib
As part of angiogenesis, growth factors and pathways exist that signal through TK-based receptors (122). Two of three VEGF receptors (VEGFR-1 and VEGFR-2) are structurally related and possess TK activity. Other receptors possess TK activity and may contribute to angiogenesis, including PDGF receptors, FGF receptors α and β, and VEGFR-3. Therapies targeting multiple TK receptors may allow for more potent suppression of angiogenesis than with VEGF inhibition alone (133).
Pazopanib is a small molecule inhibitor of multiple receptor TKs, including VEGF receptor-1, -2, and -3; PDGF receptors α and β; c-kit/CD117; FGF receptor-1, -2, and -3; and c-fms/CD115 (134). In vitro studies revealed that pazopanib inhibited VEGF expression by the RPE and choroidal endothelium (122). In laser-induced CNV in rats, topical pazopanib was found to statistically significantly decrease leakage and CNV size as compared to placebo. Pazopanib has undergone multiple phase I studies to date. It is currently involved in a phase IIb, dose-ranging study evaluating pazopanib eye drops versus intravitreal ranibizumab for exudative AMD (135). Five hundred and ten patients are enrolled, and outcomes include change in VA and number of intravitreal ranibizumab injections. Five pazopanib dosing regimens are being evaluated, and patients are able to receive ranibizumab injections as needed. Outcomes will be measured at 52 weeks.
TG100801
TG100572 is a molecule that inhibits multiple enzymes, including Src kinases and selected receptor TKs (136). Systemic TG100572 in a murine model of laser-induced CNV caused regression of the CNV, but systemic toxicity occurred. Topical administration of TG100801, a prodrug of TG100572, achieved a high retinal concentration, significantly suppressed laser-induced CNV, and reduced fluorescein leakage in mice. A phase II study of topically administered TG100801 was terminated early due to irreversible corneal toxicity (137).
Vatalanib
Vatalanib is a TK inhibitor that binds VEGFR-1, 2, 3, PDGF receptor-β, and c-kit (138). Vatalanib is an attractive option, as it has good oral bioavailability and can be given without intravitreal or intravenous injections. Mouse models of ischemic retinopathy have shown that it inhibits experimental retinal neovascularization (139). Orally administered vatalanib has been evaluated with PDT versus PDT alone in patients with subfoveal CNV due to AMD (140). The study was completed in 2007, but results were not published.
a5b1 Integrin Antagonists
Integrins are transmembrane receptors that have a large role in angiogenesis, and the α5β1 subtype is significantly up-regulated on tumor vessels (141), and expression is further increased by other growth factors. It is highly expressed in experimentally induced CNV (124) and promotes adhesion and proliferation of endothelial cells (142) and RPE (124).
JSM6427 is a small molecule inhibitor of α5β1 and has been shown to induce regression of murine models of CNV when given systemically (124). Further, it has been shown with intravitreal injection to provide dose-dependent inhibition of CNV in monkey and rabbit laser-induced and growth factor–induced models (141). JSM6427 has been investigated in a phase I study evaluating intravitreal administration in patients with exudative AMD (143). The study was completed in 2009, but results were not published.
Volociximab is a human IgG antibody to integrin α5β1 that has been shown to be a potent inhibitor in a cynomolgus model (144). A phase I clinical trial is under way to evaluate intravitreal volociximab in patients with subfoveal CNV (145).
Sonepcizumab
Sonepcizumab is an antibody to S1P (125). S1P is a lipid molecule on vascular endothelial cells that promotes their migration and survival. Receptors for S1P are up-regulated in ischemic retina, and deficiencies for these receptors demonstrate less neovascularization. Intraocular injections of sonepcizumab suppressed retinal neovascularization in murine models of oxygen-induced ischemic retinopathy. Furthermore, murine models of laser-induced CNV revealed that sonepcizumab reduced CNV area and fluorescein leakage. A phase I study has been completed (146) and a second is under way to evaluate its use in patients with persistent pigment epithelial detachments due to AMD or polypoidal choroidal vasculopathy (147). Patients are being recruited for a larger phase II study in exudative AMD (148).
ATG003
ATG003 is a topical eye drop formulation of mecamylamine, a nAChR antagonist toward one pathway that mediates angiogenesis (149). Nicotine has been shown to be proangiogenic, mediated through the nAChR (123). Antagonists toward this pathway suppress nicotine-induced retinal angiogenesis. Topical ATG003 (0.3% or 1.0%) was evaluated in a phase II randomized, placebo-controlled, double-masked study in exudative AMD (150). The study was terminated early. The primary outcome measure was proportion of patients losing fewer than 15 Early Treatment Diabetic Retinopathy Study (ETDRS) letters at 48 weeks compared to baseline, which was achieved in 88% in the 1% ATG003 group, 86% in the 0.3% ATG003 group, and 92% in placebo group (P > 0.05). ATG003 was completed in a second phase II study for exudative AMD, but results were not published (151).
Anti-VEGF Receptor Vaccine
Anti-VEGF receptor vaccine therapies represent an immune-related therapy for CNV. Inducing cytotoxic T lymphocyte responses to specific molecules expressed in vascular endothelium may decrease angiogenesis (152). Immunization with recombinant VEGFR2 protein has been shown to inhibit tumor growth and angiogenesis in mice (153). In another study, mice immunized with the VEGFR2 peptide had a significantly lower size of laser-induced CNV as compared to controls (152). A phase I study to evaluate vaccination with the VEGFR peptide was started in 2008, but the status is currently unknown (154).
VISUAL CYCLE INHIBITORS
The initial steps of visual input include the absorption of light by a chromophore (2). Rhodopsin and the color opsins are photoreceptor proteins located in the outer segments that associate with the visual chromophore, 11-cis-retinal. When a photon of light is absorbed, 11-cis-retinal isomerizes to all-trans-retinal and a conformational change occurs in the rhodopsin and color opsin proteins, leading to a change in an outer segment cation channel. This hyperpolarizes the cell and transmits an electrical impulse that eventually reaches the brain. The visual cycle involves the regeneration of the chromophore.
The regeneration of the visual chromophore, 11-cis-retinal, is a complex sequence of steps that occurs in the photoreceptor, extracellular space, and the RPE (2). It can also be formed from dietary vitamin A. After the chromophore and rhodopsin or color opsins undergo conformational changes, all-trans-retinal is converted to all-trans-retinol. All-trans-retinol is then released into the extracellular space and is taken up by the RPE along with shed outer segments. Serum all-trans-retinol is also taken up by the RPE and is delivered by retinol-binding protein (RBP). The RPE-specific 65-kDa protein (RPE65), also known as isomerase, is the enzyme that generates 11-cis-retinol. 11-cis-retinol is then oxidized to 11-cis-retinal and transported back to the photoreceptor as a visual chromophore, or it is esterified and stored in the RPE.
There are several fundamental differences between rods and cones that have clinical implications (2). Rods detect single photons, are slower acting, and are very sensitive, whereas cones are much less sensitive, but respond several times faster. Rods require 11-cis-retinal from the RPE to regenerate rhodopsin. Cones use 11-cis-retinal but can also use 11-cis-retinol to regenerate opsins. For the above reasons, inhibition of the generation of 11-cis-retinal is expected to have a greater effect on rod function.
Lipofuscin
Lipofuscin is a heterogeneous aggregation of material that forms in the RPE in a number of retinal disorders, including AMD, Stargardt disease, and types of cone–rod dystrophy and retinitis pigmentosa (155). Lipofuscin builds up in the lysosomes of the RPE, which phagocytizes shed outer segments, and incomplete digestion of these retinaldehyde-rich segments result. The material is composed primarily of retinoid precursors and oxidatively damaged lipids (156). The main component is A2E, a complex of vitamin A aldehyde and ethanolamine in a 2:1 ratio (157) but also includes other bisretinoids (158). Lipofuscin is not present in mice when the visual chromophores are absent (159). A2E is cytotoxic. It has been shown to impair phospholipid degradation and lysosomal degradation (160), induce the release of proapoptotic proteins (161), destabilize cellular membranes (162), and cause DNA fragmentation (163). Blue light is within the excitation spectra of A2E and causes the generation of singlet oxygen, resulting in apoptosis and, ultimately, RPE cell death (164). The overlying photoreceptors are then lost.
Lipofuscin accumulates with age and in AMD (165). In AMD, phagocytosis and the metabolic load of the RPE is increased. As the RPE cells die, the metabolic demand of the remaining RPE cells increase, leading to a self-accelerating accumulation of lipofuscin and RPE cell death. This is especially evident in the macula, where the number of photoreceptors per RPE cell and the amount of lipofuscin is increased. Evidence suggests that areas rich in lipofuscin eventually lead to atrophy (166). This is evident in GA, where the atrophic area is hypoautofluorescent, but there is prominent hyperautofluorescence surrounding these areas in the junctional area adjacent to normal retina. New areas of atrophy may emerge from these areas of hyperautofluorescence.
The efficacy behind visual cycle inhibitors is in their ability to slow the synthesis of 11-cis-retinal, causing depletion of all-trans-retinal, the precursor to A2E (2). The visual cycle and the medications that inhibit it are shown in Figure 25.2. Lipofuscin accumulation is dependent, in part, on dietary vitamin A. Dietary retinol deficiency can reduce lipofuscin accumulation and autofluorescence (167). Visual cycle inhibitors have been found to have several effects in the human and animal eye. Inhibitors slow the rod recovery time after bleaching light (168) and reduce the levels of A2E in the RPE (169).
Figure 25.2 A simplified diagram depicting the visual cycle and the regeneration of 11-cis-retinal. The sites of action of various therapies are included. RBP, retinol-binding protein; RPE, retinal pigment epithelium; RDH, retinol dehydrogenase; IPM, interphotoreceptor matrix.
Isotretinoin
Isotretinoin (Accutane) is a systemic vitamin A derivative used in the treatment of severe acne. Also known as 13-cis-retinoic acid, it inhibits 11-cis-retinol dehydrogenase and decreases the regeneration of 11-cis-retinal in the RPE (169) and may also have an effect on the RPE65 enzyme (2). Animals treated with isotretinoin showed reduced lipofuscin and delayed rod recovery following exposure to bright light (169). Systemic toxicity occurred before photoreceptor death (168). In humans, isotretinoin therapy was linked with nyctalopia, daytime glare, abnormal dark adaptation, and abnormal ERG (170). Doses required to inhibit the visual cycle in humans are too high and carry significant systemic side effects.
Fenretinide
Fenretinide, also known as N-(4-hydroxyphenyl)retinamide, is a retinoid analog that has been used for systemic malignancies (2). It binds to RBP and competes with retinol (171). The binding of fenretinide–RBP prevents its association with transthyretin and leads to renal excretion of the complex (172). Treatment in mouse models of Stargardt disease slowed the accumulation of A2E and its precursors and led to delays in dark adaptation (155). In patients treated for systemic malignancy, there was a reversible and transient decrease in serum retinol and RBP, delayed dark adaptation, and reduced scotopic ERG (173,174).
Fenretinide was tested in a 24-month, double-masked, placebo-controlled, prospective phase II study involving 246 patients with GA (175). Patients received daily fenretinide (100 or 300 mg) or placebo. A reduction of RBP of 60% (achieved in 43% of those treated with 300 mg) experienced a lesion growth of 30%, as compared to 50% in the placebo group. In those who experienced less lesion growth, vision loss was six letters as compared to 11 in the placebo group. Rates of CNV were lower in the fenretinide groups (13%–14%) versus placebo (22%).
ACU-4429
ACU-4429 is a nonretinoid molecule that inhibits RPE65, slows the visual cycle in the rods, and decreases the accumulation of A2E. Phase I studies have been completed (158). Adverse effects were not uncommon, as 50% experienced a dose dependent but transient and reversible visual change (dyschromatopsia, nyctalopia, blurred vision, or photophobia), but none of the adverse effects were serious. Mildly abnormal dark adaptation did occur, but cone responses were unchanged. A placebo-controlled phase II study is ongoing (176). Estimated enrollment is 84 patients, and patients are given one of several doses daily for 90 days. The primary outcome measure is safety, and serum drug levels are being checked.
OXIDATIVE STRESS
ROS include free radicals, hydrogen peroxide, and singlet oxygen (1,3). Free radicals contain one or more unpaired electrons, and hydrogen peroxide and singlet oxygen are unstable molecules. All of these compounds damage carbohydrates, membrane lipids, proteins, and nucleic acids. ROS are increased in the setting of irradiation, aging, inflammation, high oxygen tension, air pollutants, cigarette smoke, and reperfusion injury (177). The body has several ways of protecting from ROS-induced damage, including compartmentalization of the ROS, antioxidant enzymes, antioxidant vitamins, and others.
The retina is prone to oxidative damage (178). There are high levels of irradiation exposure, large amounts of oxygen consumption, and photosensitive chemical buildup (3). The photoreceptor outer segments are rich in polyunsaturated fatty acids, which are easily oxidized (179), and phagocytosis by the RPE generates ROS (180). Lipofuscin is derived from photoreceptor outer segments and is more concentrated in the posterior pole (181). It is a photosensitizer, leading to oxidative damage, and impairs the RPE lysosomal functions, further leading to buildup of cellular debris. Blue light generates ROS within lipofuscin (182). The above mechanisms are more prominent with age and in the macula (183).
Animal models have confirmed the role of oxidative stress in retinal disease. Blue light exposure damages photoreceptors, causes cellular proliferation, and RPE loss in monkey retinas, resembling atrophic AMD (184). Mutated superoxide dismutase predisposes to light-induced retinal damage in mice (185). Oxidative stress has been linked with complement activation. CFH, an alternative pathway inhibitor, is down-regulated by peroxide treatment of cells (186). In vitro studies showed that photoactivation of bisretinoid-laden RPE activated the alternative pathway and led to complement deposition (13). Blue light exposure in rats has led to the deposition of complement components, microglia, and inflammatory cells in the retina (187).
In humans, oxidative damage and excessive light have been linked to the progression and severity of AMD (188). Excessive light has been linked to photoreceptor damage through the generation of ROS and lipid peroxidation of retinal tissues (189). Oxidized lipids have been demonstrated in drusen (6). The ability of the RPE to protect from light-induced damage declines with age (190).
Iron
Iron has a role in oxidative stress. In addition to AMD, it has been implicated in other age-related conditions including AD and Parkinson’s disease (191), and iron chelation may help the treatment of these disorders (192). Iron, in its ferrous state (Fe2+), reacts with hydrogen peroxide to generate ROS that damage cellular proteins, lipids, and nucleic acids (193). Light irradiation causes iron release from ferritin, generating Fe2+ (188). Rats treated with deferoxamine, an iron chelator, may be protected against photic injury (194). Iron has several other roles in the eye. It is a component of the RPE65 enzyme, a cofactor for generation of photoreceptor outer segments and a cofactor for an enzyme necessary for steps in phototransduction (193). Iron may contribute to the loss of photoreceptors seen in subretinal hemorrhages (195). In animal models, iron chelation may help prevent this degeneration (Youssef TA et al. IOVS 2002;43:ARVO E-Abstract 3000). Hereditary disorders of iron overload exhibit retinal degeneration (196). Iron levels have been found to be increased in the RPE and photoreceptors of AMD patients, as compared to age-matched controls (197). Iron may also activate the complement cascade (198).
NtBHA
NtBHA is an antioxidant that has been shown to have protective effects against iron-induced mitochondrial damage (199). In cultured RPE cells, it can delay mitochondrial loss, reduce oxidizing substances and oxidative stress, increase the antioxidant glutathione, and decrease iron accumulation and its storage protein ferritin (200). It may restore some mitochondrial function in iron-overloaded RPE cells.
TEMPOL and OT-551
TEMPOL (4-hydroxy-2,2,6,6-tetramethylpiperidine-N-oxyl) is an antioxidant that may be protective in disorders involving ROS, such as ischemic stroke, cardiac reperfusion injury, and Parkinson’s disease (201). TEMPOL and its derivative, TEMPOL-H, have superoxide dismutase and ferroxidase-like activity (188). TEMPOL also has anti-inflammatory activity (202). TEMPOL-H is able to penetrate into posterior segment of the eye and may quench singlet oxygen. TEMPOL and TEMPOL-H protect against retinal ganglion cell damage in iron-loaded optic nerve crush models in vivo (203). In vitro studies have shown that TEMPOL-H suppresses short wavelength light-induced oxidative cell damage in lipofuscin-laden RPE cells, and it reduces A2E photooxidation (204). OT-551 is a hydroxylamine antioxidant that is converted by esterases to TEMPOL-H (188,202). Systemically administered OT-551 in rats protects photoreceptors from light-induced damage (188). It improved ERG amplitudes and outer nuclear layer thickness, and lowered oxidative end products in rats after intensive light exposure, as compared to controls. Similarly, systemic OT-551 preserved more RPE cell nuclei as compared to controls after oxidative stress (202).
OT-551 was evaluated in ten patients with bilateral GA, with one eye receiving topical OT-551 0.45% and the other as a control (178). At the 2-year end point, results were promising, as treated eyes had a significantly lower decrease in VA, and the proportion of patients losing ≥5, 10, and 15 letters was lower in the treated eyes. Scotoma size, retinal sensitivity, and change in drusen area did not differ significantly. OT-551 was evaluated in a larger phase II study for patients with GA (Sternberg et al. IOVS 2010;51:ARVO E-Abstract 6416). In a randomized, double-masked, placebo-controlled study, it was given topically for 24 months at one of two doses (0.3% and 0.45%). Forty-four of the 137 patients finished the 24 month end point at the time of study termination. The increase in size of GA from baseline to month 18 was not statistically different between the treated and placebo groups. The change in VA and percentage of subjects losing ≥15 letters at month 18 was also not different. Thus, although topically administered OT-551 was safe and well tolerated, it did not slow GA progression or maintain VA.
5-HT1A agonists
AL-8309A is a potent serotonin 5-HT1A agonist, a class of compounds that have been found to be protective in animal models of CNS ischemia and traumatic brain injury (205). This compound also provides structural and functional protection against blue light–induced retinal damage in rats, as topical pretreatment decreased microglial and T-lymphocyte activation and migration into the retina (187). The deposition of alternative complement components was nearly completely inhibited. Up-regulation of antioxidant enzymes and other antiapoptotic proteins occurred. The HCl salt of this compound, AL-8309B, is being evaluated in the treatment of GA in a phase III study (206). Five hundred and fifty patients were randomized to topically administered AL-8309B at doses of 1.75% or 1.0% or the vehicle given twice daily. Patients are treated for 24 months, and the outcome measures include VA and growth of the GA.
OTHER PATHWAYS
RNA-144101
Toll-like receptors (TLRs) are a family of membrane receptors that are expressed in ocular tissues and, when activated, induce the secretion of proinflammatory cytokines and angiogenic factors (207). The presence of TLR3 in CNV membranes was evaluated by Maloney et al. (208). Immunostaining on eight AMD patients and four controls without CNV revealed that TLR3 was found in all CNV membranes and was expressed in the RPE but was not seen in the posterior pole of three of the four controls. TLR3 may also contribute to GA, as this pathway triggers caspase-3–mediated apoptosis of the RPE (209). SiRNAs have been developed that suppress angiogenesis through the TLR3 pathway (210). RNA-144101 is a siRNA targeted against TLR3. A phase I study is under way to evaluate its safety, tolerability, and dosing when administered intravitreally in patients with GA (211).
Vasodilators
Studies have suggested that impaired choroidal perfusion may play a role in AMD. Some have hypothesized that the accumulation of hydrophobic lipids in Bruch’s membrane decreases perfusion, resulting in deposition of protein and lipids and decreasing the permeability of nutrients and waste products (212). The choriocapillaris may be maintained by factors secreted by the RPE, and the diffusion of these factors may be limited by a thickened Bruch’s membrane. Regulation of choroidal blood flow may be abnormal in patients with CNV, as pericytes within the CNV may exhibit altered contraction capabilities (213).
In a recent study of dry AMD, there was an association between increased drusen extent and decreased choroidal blood flow (214). Color Doppler (215) and indocyanine green angiography (216) studies revealed perfusion defects in aged and AMD eyes. Piguet et al. (217) found that prolonged choroidal filling was associated with vision loss. In eyes with prolonged choroidal filling, 38% lost two lines at 2 years, as compared to 14% of eyes with normal choroidal filling. Chen et al. (218) found that patients with and without abnormal choroidal perfusion were identical in VA and fundus appearance. It is unknown if choroidal filling defects are the primary cause or secondary to the disease process in AMD and that hypofluorescence may be due to abnormal staining or permeability of Bruch’s membrane, not choroidal ischemia (212).
Prostaglandin E1 (PGE1) is used for limb ischemia by enhancing blood flow (219). It has been noted that patients receiving PGE1 infusions for peripheral arterial disease reported spontaneous improvements in vision. One study revealed improvements in visual function and foveal sensitivity in patients with AMD receiving intravenous PGE1 for 3 to 5 weeks (220). Another study found that 6-month therapy with PGE1 showed a mild improvement of vision relative to controls in 11 patients. A phase III study of PGE1 (Alprostadil) for dry AMD revealed no difference between PGE1 and control groups at 3 months, but this study was terminated early (221).
Trimetazidine (TMZ) is an anti-ischemic agent used in Europe for angina pectoris, VA and visual field loss due to presumed vascular causes, vertigo and tinnitus (222). Ischemic retinal injury is mediated in part by the excitatory neurotransmitter glutamate, a potent neurotoxin (223). In animal models, TMZ can decrease damage from retinal ischemic injury (224) and inhibit the buildup of glutamate (223). TMZ was evaluated for patients at high risk of advanced AMD in a phase III, prospective, double-blinded, randomized, placebo-controlled trial (222). Seven hundred and twelve patients completed the 3 years of follow-up. Thirty-three percent of patients in both treatment groups (TMZ and placebo) developed CNV. The incidence of GA nonsignificantly favored the TMZ group.
Summary
In summary, several different pathways, including chronic inflammation, complement deposition, oxidative stress, angiogenesis, and visual cycle toxic by-products, have been associated with the development and progression of AMD. Several therapies are being investigated in ongoing clinical trials, and additional therapies are in preclinical testing, and new, unrecognized pathways may also become apparent. We are likely going to see new medications become available for both dry and exudative AMD in the near future.
REFERENCES
1.Zampros I, Praidou A, Brazitikos P, et al. Antivascular endothelial growth factor agents for neovascular age-related macular degeneration. J Ophthalmol. 2012; 319728:2012.
2.Travis GH, Golczak M, Moise AR, et al. Diseases caused by defects in the visual cycle: retinoids as potential therapeutic agents. Annu Rev Pharmacol Toxicol. 2007;47:469–512.
3.Beatty S
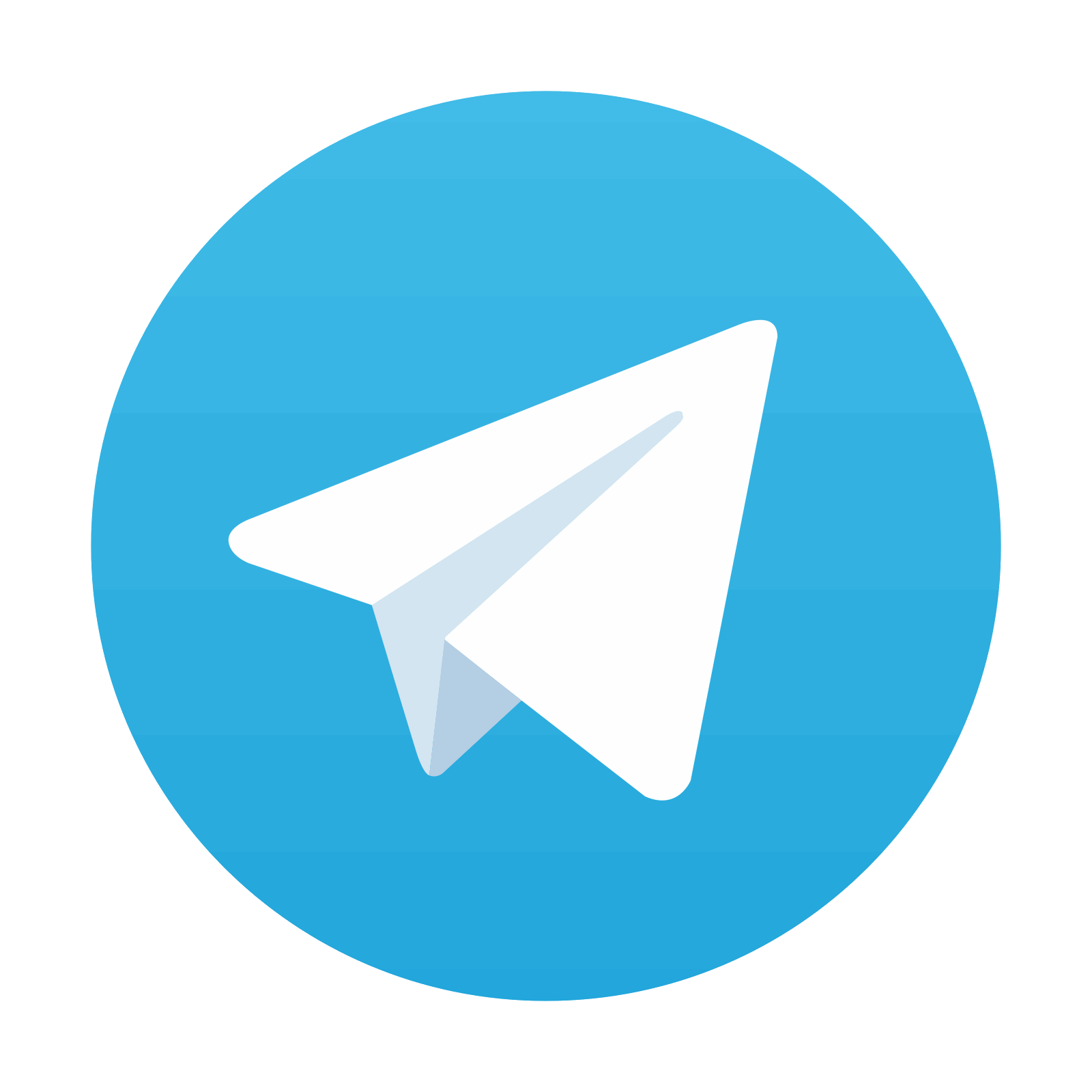
Stay updated, free articles. Join our Telegram channel

Full access? Get Clinical Tree
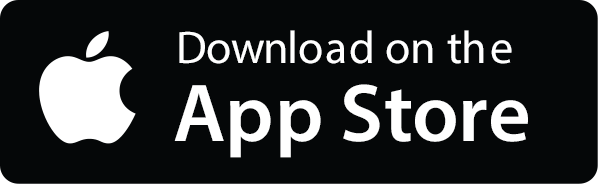
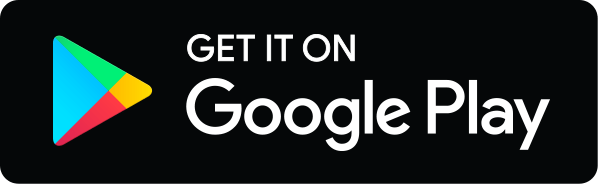