Fig. 80.1
Intraoperative OCT imaging during resection of glioblastoma (WHO grade IV), which recurred after initial surgery and radiation. A pilot laser was used to focus the OCT probe on the target volume. The center of the scan line was registered by the acquisition of marker points using a neuronavigation system. (a) Collagen-rich cortical scar: H&E stained (left) and with deposition of iron pigmented macrophages (right; Berlin blue reaction). (b) Microcystic changes within the tumor infiltration zone. (c) Highly cellular tumor with vascular proliferations and pleomorphic tumor cells. (d) Pleomorphic tumor cells (right) and tumor necrosis (left) within the center of the tumor (b–d H&E staining) (From Ref. [23], published by Springer Science+Business Media)
80.2 Applications in Breast Cancer
80.2.1 Intraoperative Assessment of Tumor Margins: Background
Early cancer detection with x-ray mammography, ultrasound, and MRI allows surgeons in most cases to remove smaller tumors while preserving as much of the normal breast tissue as possible (during lumpectomy procedures). After the surgery, histopathology is performed on the excised tissue to assess the margin status. If the margin contains cancer cells (“positive” margin) or the cancer is present within a certain depth of the resected surface (“close” margin), then the patient is advised to undergo re-excision surgery until a “negative” margin is achieved (Fig. 80.2a). In a survey, 31 % of radiation oncologists defined a close margin as having no cells within 1 mm and an additional 38 % defined it to be no cells within 2 mm of the surface [24]. Since then more studies correlated the width of uninvolved margins to local recurrence of cancer, which has allowed surgeons to use 1 mm to define a “negative” margin and encourage breast conservation surgeries [25, 26].
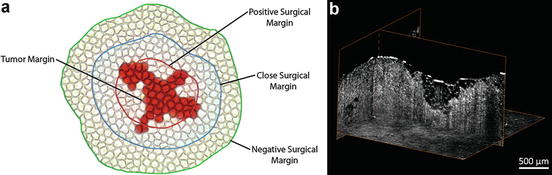
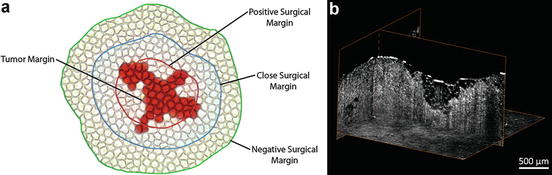
Fig. 80.2
Surgical margins and tumor margins in breast cancer surgery. (a) Surgical classification of the tumor margin status depends on the distance of cancer cells from the surface of the resected tissue. If the surgical margin is located along the tumor margin or the tumor cells are found beyond the surgical margin, the surgical margin is considered “positive” (red line). A “close” surgical margin (blue line) does not have tumor cells within 1 mm or 2 mm from the surgical margin. Otherwise, a surgical margin is considered “negative” (green line). The tumor margin follows the edge of the solid tumor itself, and it does not include any metastatic cells that may have migrated away from the primary solid tumor mass (Own work published in SPIE Proceedings, doi:10.1117/12.2040315) [70]. (b) Three-dimensional OCT image of a positive margin from an excised human breast tumor mass (Own work unpublished)
In a large number of studies, it was found that the margin status was the key predictor of local recurrence of cancer, and positive margins have contributed significantly to a high rate of repeated surgeries [27–38]. A positive margin, with cancer cells on the surface of the resected tissue, occurs in at least 30–35 % of cases, and an additional 10–15 % are classified as close margins [37].
Currently, no real-time intraoperative method exists to rapidly assess the status of lumpectomy margins to determine if additional tissue needs to be removed [39, 40]. None of the investigated techniques, including frozen section analysis, touch prep cytology, specimen radiography, and radiofrequency spectroscopy, satisfy the necessary requirements. Frozen section analysis has a sensitivity of 73 % and a specificity of 98 % compared to paraffin section analysis for breast cancer, but is not widely accepted due to difficulties in performing frozen sections on adipose tissue and increased operating room time (∼20–30 min) [39]. Overall, current histological methods cannot rapidly determine the surgical margin status due to time, cost, and technology limitations. Touch prep cytology with reported sensitivities of 75 % and specificities of 83 % can rapidly assess the entire margin area [41]. However, this technique requires that the tumor cells are present at the surface and that they detach and adhere to the slide for microscopic examination. Touch prep cytology does not provide information about the presence of cancer cells beneath the surface and thus is unable to determine close and negative margins. Surgeons can visualize tumor margins using intraoperative specimen radiography by displaying two-dimensional x-ray projections. Unfortunately, this technique has a rather low sensitivity (49 %) and specificity (73 %) which is primarily due to its inability to identify diffuse microscopic processes, especially where the tumor boundary is poorly defined [42, 43]. Radiofrequency spectroscopy with low sensitivity (71 %) and specificity (68 %), shallow penetration depth (100 μm), and low resolution offers very limited detection within 1–2 mm for margin classification [44].
Among all optical techniques, OCT has the greatest potential for in situ label-free intraoperative margin assessment in breast-conserving surgeries, since it acquires high-resolution images in the same physical range as that used in histological analysis to classify surgical margins as positive, close, or negative (Fig. 80.2b). Figure 80.3 shows some representative OCT images of negative and positive surgical margins obtained ex vivo from resected breast tumors. The observations made by OCT were confirmed by histopathology.
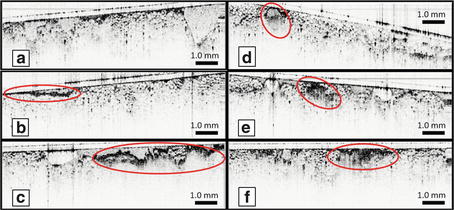
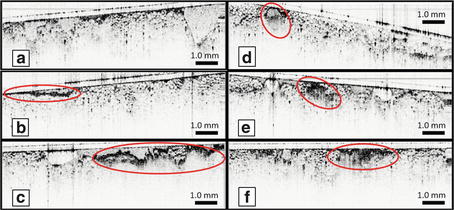
Fig. 80.3
Intraoperative OCT images of resected breast tissue specimens a human patient diagnosed with ductal carcinoma in situ (DCIS). (a) OCT of normal tissue was identified by the homogenous pattern of large adipose cells. (b–d) Readily identified surgical artifacts are also shown. (b) Surface blood appeared as a thin film of scatterers. (c) Cauterized tissue exhibited a distinct patch-like appearance. (d) Potential duct with a mixed distribution of low- and high-scattering areas. (e, f) Positive margins contained tumor cells and tissue represented by a highly scattering area that was more heterogeneous and disruptive compared to the surrounding tissue architecture (Adapted from [48])
80.2.2 Intraoperative Assessment of Tumor Margins: Results
The first study to demonstrate breast cancer imaging using OCT was conducted using a preclinical rat model [45]. Virgin female rats were injected with carcinogen N-methyl-N-nitrosourea (MNU), leading to the development of invasive mammary tumors. Three-dimensional imaging of the palpable tumors was performed with a time-domain OCT system centered at 800 nm with 2 μm axial and 10 μm transverse resolutions. Healthy mammary tissue was found to have a heterogeneous structure composed of mixed adipose and stroma. In the OCT data, individual adipocytes appeared as segmented oval-shaped objects, while stroma appeared dense and highly scattering. OCT imaging of early-stage tumors revealed thickening and stronger scattering in the ductal morphology, suggesting precancerous changes prior to full tumor invasion. Late-stage solid tumor was identified as dense and highly scattering, similar to the scattering intensity from stroma. Despite this similarity, the morphology of the tumor caused it to appear distinct from the surrounding tissue. While the tumor margin boundary was visible in the 2D representation, 3D visualization facilitated detection of the tumor boundary. Results from this study suggest that while single tumor cells may be challenging to locate, precancerous changes and significant tumor invasion can be detected using OCT.
For OCT to guide surgical procedures, it is necessary to understand how specific histological features appear in OCT images of human breast tissue. To accomplish this goal, a comparison study was conducted between OCT and the corresponding histology sections of human breast tissue [46]. Two-dimensional and three-dimensional spectral-domain OCT imaging was performed at a center wavelength of 1090 nm with 3.5 μm axial and 6 μm transverse resolutions. A total of 119 specimens were imaged from 35 patients covering several pathologies including ductal carcinoma in situ (DCIS), infiltrating tumor, and microcalcifications. Specimen radiography was performed on freshly excised tissue in the pathology lab to guide OCT imaging to suspicious sites. A non-blinded comparison was made between OCT and histology findings. Analysis of healthy tissue revealed clear visualization of adipose, stroma, ducts, and glands. Benign changes including cysts, fibroadenoma, and ductal hyperplasia were also visible. Comparison results for lobular DCIS are shown in Fig. 80.4a. Normal adipose tissue and stroma are visible along the surface of the sample. Enlarged, low-scattering DCIS lobules visibly distort the surrounding structure, and a microcalcification is visible as a small high-scattering area. OCT and histology comparison of invasive ductal carcinoma is shown in Fig. 80.4b. Tumor appears as highly scattering irregular “tongues” which protrude into the desmoplastic stroma. Separate calcifications are also visible. Solid infiltrative ductal carcinoma was also imaged, yet it was difficult to distinguish from stroma due to the highly scattering uniform structure. Results from the comparison study demonstrate that OCT is capable of detecting normal, benign, and malignant tissue structures in the human breast.
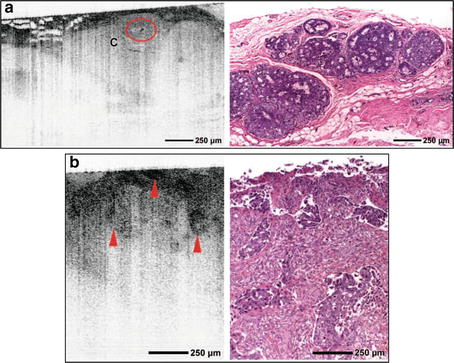
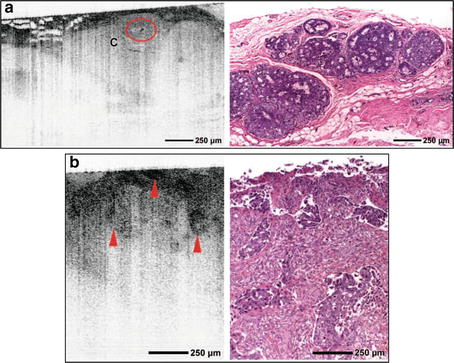
Fig. 80.4
Comparison of tumor features in OCT and histology. (a) OCT image and corresponding histology of DCIS lesions in lobules. Tumor cells within lobules appear uniformly low scattering. Dilatation and architectural distortion of the lobules are visible. A microcalcification (C, circled area) within the lobules appears highly scattering with pronounced shadowing. (b) OCT image and corresponding histology of infiltrating ductal carcinoma. Highly scattering regions (arrowheads), which correspond to tongues of invasive cancer, are visible (Adapted from [46], reprinted with permission from Radiological Society of North America)
A study comparing OCT, optical coherence microscopy (OCM), and histology of breast tissue has shown similar results [47]. An integrated OCT-OCM system was used with 4 μm axial resolution and 14 μm or 2 μm transverse resolution for OCT and OCM, respectively. A total of 34 benign and 10 malignant ex vivo specimens were imaged 2–6 h post resection. A non-blinded comparison of OCT with histology located adipose, stroma, benign lobules and ducts, cysts, and in situ and invasive carcinoma. These results were consistent with previous studies, with the addition of higher-resolution optical scattering data provided by OCM.
The first intraoperative OCT breast margin assessment study was published by Nguyen et al. [48]. A portable spectral-domain OCT system was constructed for imaging in the operating room. The system performed 3D imaging at a center wavelength of 1,310 nm with 5.9 μm axial and 35 μm transverse resolutions. A total of 37 patient specimens were imaged immediately following resection. Suspicious areas were located for OCT imaging by visual inspection and palpation. The imaged areas were then marked with surgical ink for correlation with histology. Seventeen of the patient specimens were used as a training set. The training set data included both negative (no tumor present) and positive (tumor present) margins and revealed confounding factors such as pooled blood and cauterized tissue which both appeared highly scattering, mimicking the scattering signature of tumor tissue. When classifying negative and positive margins, these confounding factors must be carefully considered (Fig. 80.3). Highly scattering blood on the tissue surface was easily removed by flushing the tissue with saline. Cauterized tissue was identified as a highly irregular, intensely scattering, and highly attenuating surface that blocked all signals from beneath the cauterized and charred surface. The remaining 20 patient specimens were used to determine the feasibility of tumor margin assessment using OCT. Out of the 20 margins, 11 were diagnosed histologically positive and 9 were diagnosed histologically negative. A blinded analysis of OCT data was performed and compared with histology results. OCT data sets containing tumor cells or tumor tissue were classified as positive, while data sets void of tumor were classified as negative. Representative tumor margins are shown in Fig. 80.5. As expected, tumor cells are identified by high scattering and heterogeneous structure, in contrast with normal fibroadipose tissue. Comparison of OCT analysis and histology resulted in 100 % specificity and 82 % sensitivity for using OCT to identify positive margins intraoperatively in real time, compared to postoperative assessment of histological tissue sections. This demonstrates that intraoperative breast margin assessment with OCT is feasible and warrants further study.
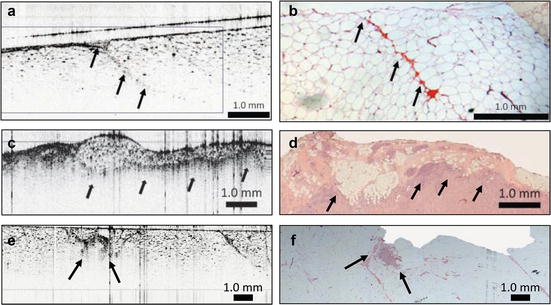
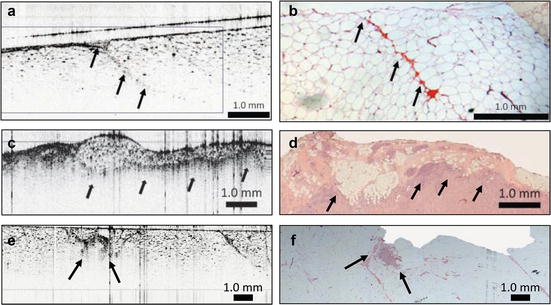
Fig. 80.5
Surgical margins imaged using OCT. (a) OCT and (b) histology of a negative tumor margin. Adipose cells and microvasculature (arrows) are present. (c) OCT and (d) histology of positive tumor margin. OCT shows a distinct heterogeneously scattering region (arrows) with small, highly scattering foci indicative of collections of tumor cells. (e) OCT and (f) histology of positive tumor margin with an isolated tumor focus (arrows) (Adapted from [48])
80.2.3 Intraoperative Assessment of Sentinel Lymph Nodes: Background
Lymph nodes are anatomically distributed along locoregional drainage patterns in the body and function as filters to capture and retain potentially harmful macromolecules and microorganisms and provide a tissue microenvironment to facilitate immunological surveillance of various antigens. Lymph nodes have a well-defined structure with an outer capsule, subcapsular sinus, cortex containing follicles, medullary sinuses, medullary cords, and hilus [49]. The organization of the lymph node is well suited for its filtering role. The lymph enters through the afferent lymphatic vessels into the marginal sinuses, under the capsule, and moves into the medullary sinuses (Fig. 80.6a). The system of sinuses retains the particles, facilitating immune cells in their monitoring functions.
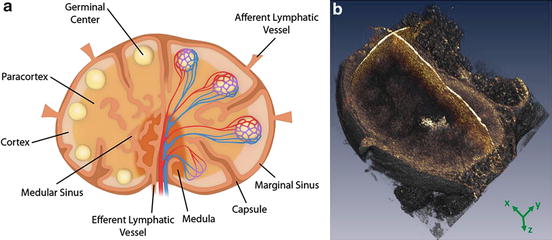
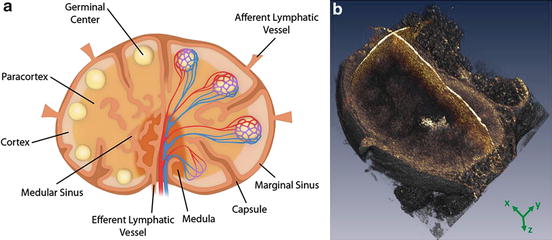
Fig. 80.6
Lymph node anatomy. (a) Diagram of a lymph node indicating basic structural components (Own work unpublished). (b) Three-dimensional transcapsular OCT of a normal rat lymph node featuring an intact capsule and follicular structures that were distinguishable from the background cortex based on their spheroidal pattern of optical scattering (Adapted from [64], reprinted with kind permission from Springer Science and Business Media)
Inactivated (normal) lymph nodes contain a relatively small number of active immune cells. Upon interaction with antigens, lymph nodes became enlarged mainly due to the recruitment and activation of lymphocytes and macrophages involved in the primary immune response. In the case of a metastatic node, in addition to the inflammatory response, there is also the presence of infiltrating and dividing cancer cells. It is well known that cancer cells from the primary metastatic tumor often travel via the lymphatic system and accumulate in the lymph nodes. Most of the lymph from the breasts (80–100 %) drains into the axillary lymph nodes, making them important in the staging of breast cancer. In the past, it was common to remove 10–30 axillary lymph nodes in order to stage disease progression using postoperative histopathological analysis and determine the most appropriate therapeutic treatment. However, removal of lymph nodes can cause disruption of the lymphatic drainage network and obstruction of lymphatic flow, leading to long-term complications such as numbness, sensitivity, and accumulation of lymph fluid or lymphedema. To decrease these postoperative morbidities and complications, most surgeons are now removing only the sentinel lymph nodes (the first nodes collecting the lymph from the tumor site, Fig. 80.6) for biopsy [50]. It was shown in clinical studies that sentinel lymph nodes are sufficient for staging breast cancer and assessment of metastasis [51].
There are several techniques that are currently used to identify and localize lymph nodes before their resection. A radioactive tracer (technetium-99) and a dye (methylene blue) are injected preoperatively near the site of the tumor [50, 52]. They are allowed to circulate, and the sentinel nodes are identified by the color or the level of radioactivity. After resection, lymph nodes are processed and analyzed using standard histological techniques. Fluorescence techniques using near-infrared dye [53, 54] that could provide better localization of the lymph node are also being investigated, but none of the mentioned techniques can provide the assessment of the lymph node status.
Intraoperative techniques currently used for the staging of cancer by assessing lymph nodes require lymph node resection and dissection to expose the internal structure for high-resolution imaging [55, 56] or prolong the surgery. Surgeons often send suspicious nodes for a quick assessment via frozen sections, which could take 20–30 min to perform, increasing the time that the patient is under general anesthesia [57, 40]. Most of the other noninvasive imaging modalities currently used in the clinical setting (x-ray, MRI, CT) can provide information only about the size and general morphology of the lymph nodes [58] and cannot provide any information about the presence of cancer cells in the early stages of metastasis [59, 60]. With a 1–2 mm imaging penetration depth, OCT has a potential for intraoperative in situ imaging of intact lymph node structure and assessment of status, primarily by imaging the capsule and the outer cortex containing the follicles and germinal centers (Fig. 80.6b) [45, 61, 48]. During metastatic progression, cancer cells first infiltrate sentinel lymph nodes in the subcapsular region, and the architectural changes in lymph node morphology all primarily occur in the outer cortex and capsular regions, which are within the imaging depth of OCT [61–65]. In addition, the proliferation of adipocytes in and around the lymph nodes has also been correlated with metastatic involvement, which can be identified and imaged with OCT [71].
80.2.4 Intraoperative Assessment of Sentinel Lymph Nodes: Results
Initial OCT research in lymph node imaging was published in 2005 where three-dimensional time-domain OCT imaging was performed on both rat and human lymph nodes [61]. Many important structures were detected such as the capsule, cortex, sinuses, and follicles. Two freshly excised metastatic human lymph nodes were also imaged and exhibited highly scattering tumorous and necrotic areas. This study demonstrated that although the entire depth of the node cannot be imaged, transcapsular OCT imaging of intact lymph node is still capable of detecting many diagnostically significant features.
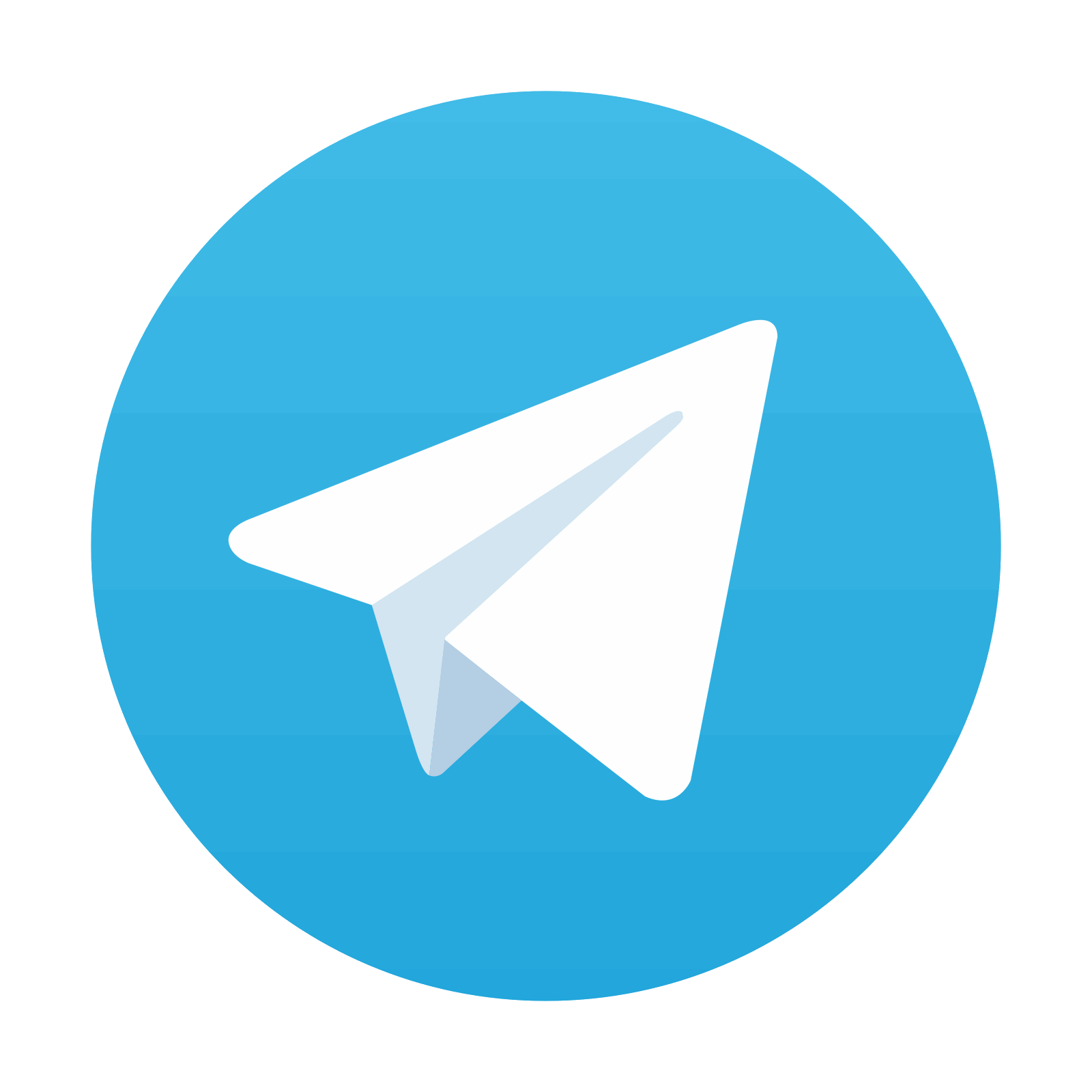
Stay updated, free articles. Join our Telegram channel

Full access? Get Clinical Tree
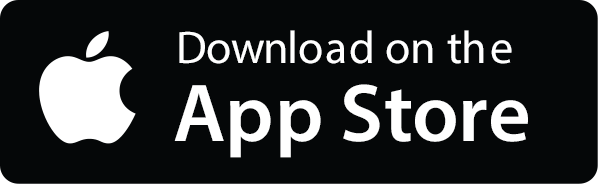
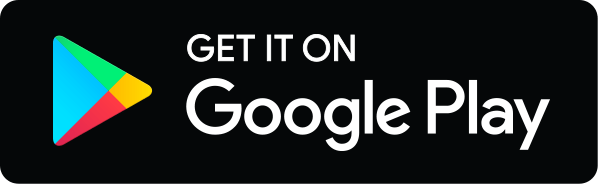