CHAPTER 40 Immunology of the Upper Airway and Pathophysiology and Treatment of Allergic Rhinitis
Innate Immunity and Adaptive Immunity
Broadly defined, the innate immune system consists of all aspects of the host defense mechanisms that are encoded in the germline genes of the host. These include barrier mechanisms, such as epithelial cell layers that express tight cell-cell contact, the secreted mucus layer that overlays the epithelium, and the epithelial cilia that sweep away this mucus layer. The innate response also includes soluble proteins and small bioactive molecules that either are constitutively present in biologic fluids (such as the complement proteins and defensins)1,2 or are released from activated cells (including cytokines, chemokines, lipid mediators of inflammation, and bioactive amines and enzymes). Activated phagocytes (including neutrophils, monocytes, and macrophages) are also part of the innate immune system.
Innate Immune System
Toll-Like Receptors
An important advance in our understanding of innate immunity to microbial pathogens was the identification of a human homolog of the Drosophila Toll receptor. Mammalian TLR family members are transmembrane proteins containing repeated leucine-rich motifs in their extracellular portions. Mammalian TLR proteins contain a cytoplasmic portion that is homologous to the interleukin-1 (IL-1) receptor, and can therefore trigger intracellular signaling pathways. TLRs are pattern recognition receptors that recognize pathogen-associated molecular patterns present on a variety of bacteria, viruses, and fungi. The activation of TLRs induces expression of costimulatory molecules and the release of cytokines that instruct the adaptive immune response. Finally, TLRs directly activate host defense mechanisms that directly combat the foreign invader or contribute to tissue injury.3
Expression and Distribution of Toll-Like Receptors
TLRs were initially found to be expressed in all lymphoid tissue but are most highly expressed in peripheral blood leukocytes. Expression of TLR messenger RNA (mRNA) has been found in monocytes, B cells, T cells, and dendritic cells.4,5 The expression of TLRs on cells of the monocyte-macrophage lineage is consistent with the role of TLRs in modulating inflammatory responses via cytokine release. Some TLRs are located intracellularly, like TLR9.
Activators of the Different Toll-Like Receptors
Lipopolysaccharides (LPSs) of gram-negative bacteria generate responses mediated via the TLR4 receptor.6,7 Microbial lipoproteins and lipopeptides have been shown to activate cells in a TLR2-dependent manner.8 Lipoproteins have been found extensively in both gram-positive and gram-negative bacteria as well as spirochetes. Mammalian TLR9 mediates the immune response to a specific pattern in bacterial DNA, an unmethylated cytidine-phosphate-guanosine (CpG) dinucleotide with appropriate flanking regions. These CpG-DNA sequences are 20-fold more common in microbial than in mammalian DNA; thus, mammalian TLR9 is more likely to be activated by bacterial DNA than by mammalian DNA. Human TLR9 confers responsiveness to bacterial DNA via species-specific CpG motif recognition.9 Mammalian TLR5 has been shown to mediate the response to flagellin, a component of bacterial flagella.10 Mammalian TLR3 mediates the response to double-stranded RNA, a molecular pattern expressed by many viruses during infection.11 Activation of TLR3 induces interferons IFN-α and IFN-β, cytokines important for antiviral responses. Finally, single-stranded RNA binds to TLR7 and TLR8.12
Toll-Like Receptors and the Adaptive Immune Response
Critical proinflammatory and immunomodulatory cytokines, such as IL-1, IL-6, IL-8, IL-10, IL-12, and tumor necrosis factor-α (TNF-α) have been shown to be induced after activation of TLRs by microbial ligands.3 Activation of TLRs on dendritic cells triggers their maturation, leading to cell surface changes that enhance antigen presentation, thus promoting the ability of these cells to present antigen to T cells and generate type 1 helper T cell (TH1) responses critical for cell-mediated immunity. Therefore, activation of TLRs as part of the innate response can influence and modulate the adaptive T-cell response and modify the shaping of the TH1/TH2 balance.13
Toll-Like Receptors and the Host
Like the Drosophila Toll receptor, mammalian TLRs have been shown to play a prominent role in directly activating host defense mechanisms. For example, activation of TLR2 by microbial lipoproteins induces activation of the inducible nitric oxide synthase promoter that leads to the production of nitric oxide, a known antimicrobial agent.8 In Drosophila, activation of Toll leads to the nuclear factor κB (NF-κB)–dependent induction of a variety of antimicrobial peptides.14 In a similar fashion, it has been shown that LPS induces β-defensin-2 in tracheobronchial epithelium, suggesting similar pathways in humans.15 The activation of TLRs can also be detrimental, causing tissue injury. The administration of LPS to mice can result in shock, a feature that depends on TLR4,6 and microbial lipoproteins induce features of apoptosis via TLR2.16 Thus, microbial lipoproteins have the ability to induce both TLR-dependent activation of host defense and tissue pathology. This might be one way for the immune system to activate host defenses and then down-regulate the response to avoid causing tissue injury.
Antimicrobial Peptides
Cathelicidins
Most cathelicidins undergo extracellular proteolytic cleavage that releases their C-terminal peptide containing the antimicrobial activity. The only known human cathelicidin, hCAP-18 (human cationic antimicrobial peptide, 18 kDa), was initially identified in granules of human neutrophils.17 Its free C-terminal peptide is called LL-37 and has a broad spectrum of antimicrobial activity in vitro against Pseudomonas aeruginosa, Salmonella typhimurium, Escherichia coli, Listeria monocytogenes, and Staphylococcus aureus.18 LL-37, a chemoattractant for mast cells19 and human neutrophils, monocytes, and T cells,20 induces degranulation and histamine release in mast cells.21 Thus, this AMP has the potential to participate in the innate immune response both by killing bacteria and by recruiting a cellular immune response and promoting tissue inflammation.
Defensins
Defensins are a broadly dispersed family of gene-encoded antimicrobials that exhibit antimicrobial activity against bacteria, fungi, and enveloped viruses.22,23 Defensins are classified into three distinct families: the α-defensins, the β-defensins, and the θ-defensins.
α-Defensins
The α-defensins are 29 to 35 amino acids in length. Human neutrophils express a number of distinct defensins.24 To date, six α-defensins have been identified. Of these, four are known as α-defensins 1, 2, 3, and 4 (also referred to as human neutrophil peptides HNP-1 through -4). The other two α-defensins, known as human defensins 5 and 6 (HD-5, HD-6), are abundantly expressed in Paneth cells of the small intestinal crypts,25,26 in epithelial cells of the female urogenital tract,27 and in nasal and bronchial epithelial cells.28 HNP-1 through HNP-4 are localized in azurophilic granules of neutrophils and contribute to the oxygen-independent killing of phagocytosed microorganisms. Furthermore, HNP-1 through HNP-3 can either increase the expression of TNF-α and IL-1 in human monocytes that have been activated by S. aureus or reduce the expression of vascular cellular adhesion molecule-1 (VCAM-1) in human umbilical vein endothelial cells activated by TNF-α.29
β-Defensins
In human beings, four types of β-defensins have been identified thus far; they are referred to as human β-defensins (HBDs) 1 through 4. They have a broad spectrum of antimicrobial activity, bind to CCR6, and are chemotactic for immature dendritic cells and memory T cells.30 Human β-defensin 2 can also promote histamine release and prostaglandin (PG) D2 production in mast cells, suggesting a role in allergic reactions.31,32 Thus, the defensins, like the cathelicidins, can contribute to the immune response by both killing bacteria and influencing the cellular innate and adaptive immune response.
Tissue Distribution of Antimicrobial Peptides
All of the β-defensins are present in the respiratory system. These are expressed at various levels in the epithelia of the trachea and lung as well as in the serous cells of the submucosal glands.33–35 Cathelicidins are also present in the conducting airway epithelium, pulmonary epithelium, and submucosal glands.36 Investigations have also documented the presence of these peptides in the nose and paranasal sinuses in health and diseases including rhinitis, chronic rhinosinusitis, and nasal polyposis.37–41
Adaptive Immunity: Cells of the Immune System and Their Responses
The human immune system consists of dispersed organs (spleen, thymus, lymph nodes) and of cells capable of moving from the bone marrow to the blood and the lymphatic system. An intact immune response includes many subsets of leukocytes that can be discriminated morphologically through the use of conventional histologic stains and, more accurately, by surface phenotype as defined by monoclonal antibody binding to registered differentiation antigens. These differentiation antigens are assigned cluster of differentiation (CD) numbers; updates are issued by the International Workshop on Human Leukocyte Differentiation Antigens (published at http://www.hcdm.org/Home/tabid/36/Default.aspx).
The pluripotent stem cells, which are derived from the yolk sac and ultimately reside in the bone marrow, are the progenitor cells from which all cells of the immune system are derived (Fig. 40-1). These pluripotent stem cells give rise to lymphoid and myeloid stem cells. Lymphoid stem cells differentiate further into the three major populations: T cells, B cells, and NK cells. T cells are defined by their cell surface expression of the TCR, a transmembrane heterodimeric protein that binds processed antigen displayed by APCs. B cells are phenotypically defined by their expression of the B-cell receptor for antigen, membrane-bound immunoglobulin. NK cells are defined morphologically as large granular lymphocytes. They are distinguished by their lack of either TCR or surface immunoglobulin. They recognize their virus-infected or tumor cell targets through the use of a complex collection of activating and inhibitory cell surface receptors.42 Lymphocytes represent about 25% of leukocytes in the peripheral blood. The relative contribution of each subtype to this percentage is as follows: T lymphocytes, 80%; B lymphocytes, 10%; NK large granular lymphocytes, 10%.
Differentiation of lymphoid and myeloid stem cells depends on their interaction through their surface receptors with soluble ligands (cytokines) or surface ligands (cell interaction molecules). Therefore, proliferation and differentiation along one of the myeloid or lymphoid lineages are controlled (1) through the spatially and temporally regulated exposure of these stem cells to different ligands or factors and (2) through the differential expression of receptors on the stem cells. Cytokines have pleiotropic effects on the development of lymphoid and myeloid cells, affecting both growth and maintenance of pluripotent stem cells and their development and differentiation to specific lineages. Stromal cells within the bone marrow and thymus also regulate cell growth and differentiation by releasing cytokines, such as IL-4, IL-6, IL-7, IL-11, and granulocyte-macrophage colony-stimulating factor (GM-CSF).43 They also participate in cell-cell interactions with progenitors through engagement of cell surface molecules that provide additional regulatory stimuli and participate in the development of the intercellular matrix (e.g., collagen, fibronectin).43,44
T Cells
T-Cell Development
Lymphoid stem cells leave the bone marrow and reach the thymus gland via the bloodstream. At this stage of development, these cells lack surface antigens that make up the TCR complex and do not express the CD4 or CD8 coreceptor (double-negative). These precursors begin recombination of TCR gene segments, of which there are four: α, β, γ, and δ. Recombination begins at the δ, γ, and β loci, and if expression of the γδTCR is successful, commitment to the γδ T-cell lineage results.45 γδ T cells remain double-negative and leave the thymus, populating lymphoid tissue and epithelia. A portion of the γδ T cells are generated in the thymus, with a major fraction generated in an extra thymic compartment.46 Alternatively, successful αβ loci recombination results in βTCR expression, which pairs with the surrogate α receptor and forms the pre-TCR. The pre-TCR provides ligand-independent signals enabling commitment to the αβ T-cell lineage and CD4/CD8 coexpression (double-positive). Assembly of the pre-TCR is followed by recombination at the α loci, which, if successful, generates the αβTCR. Ligand-dependent selection follows and is determined by double-positive cells binding peptide-loaded MHC molecules on thymic cortical epithelia. Double-positive cells that bind MHC with sufficient affinity are selected to survive (positive selection), whereas those that do not are eliminated by apoptosis. The interaction between the TCR and MHC molecules is restricted by the specificity of the TCR and the T-cell coreceptor: CD4 restricts interaction to class II MHC and CD8 to class I MHC. Surviving double-positive cells then lose the CD4 or CD8 coreceptor not involved in MHC recognition. These single-positive cells traffic to the thymic medulla, and those that react too strongly with self-antigens presented by medullary epithelia and bone marrow–derived APCs are deleted by apoptosis (negative selection). These processes of positive and negative selection promote viable T cells capable of recognizing and responding to peptide antigen in MHC while checking those that have autoreactivity. This is a rigorous selection, with approximately only 2% of the double-positive cells surviving. Approximately 90% to 95% of circulating T cells use the αβ TCR and are divided into two major subpopulations, CD4+ helper T cells and CD8+ cells.
T-Cell Priming
When T cells recognize their specific peptide/MHC ligand on APCs, TCR signals result in adhesion molecule changes that strengthen and prolong APC contact. During this interaction, a second costimulatory signal is provided by CD28 on T cells binding B7 molecules on APCs and facilitates activation and proliferation of naïve T cells. After costimulation, activated T cells produce IL-2, leading to T-cell proliferation and differentiation. Once primed, naïve T cells differentiate into effector cells that perform antigen-specific functions without the need for costimulation.47
Effector T Cells
Another pathway for cytotoxic cell function involves the cell surface molecule Fas, which is a member of the TNF receptor–nerve growth factor receptor–CD40 superfamily and mediates apoptosis.48 Binding of the cell surface Fas molecule on a target cell to the Fas ligand on the cytotoxic effector leads to apoptosis of the target cell. Fas-mediated cytotoxicity is thought to play a role in the negative selection process in T-cell development; however, the role of this pathway for cytolysis in various infectious diseases has not been defined.
Generally designated helper cells, CD4+ cells work to activate both humoral immune responses (B-cell help) and cellular responses (delayed-type hypersensitivity responses). After recognizing antigens presented by MHC class II molecules, CD4+ cells become activated to secrete IL-2, partly in response to monocyte-derived IL-1 and partly in response to autocrine stimulation by IL-2 as part of a positive feedback loop (Fig. 40-2). Activated CD4+ cells interact with other CD4+ or CD8+ cells by secreting IL-2, and with B cells by secreting B-cell growth and differentiation factors (IL-2, IL-4, IL-6). Thus, CD4+ cells augment immune responses by stimulating B cells sensitized by antigen, and by stimulating CD8+ cells sensitized by binding of antigen in the context of MHC class I molecules. CD4+ T-cell priming, unlike CD8+ T-cell priming, results in the differentiation of various subsets distinguished by the production of particular cytokines and effector functions. The activities of these CD4+ cells are largely mediated via the secretion of these cytokines, which are small protein hormones that control the growth and differentiation of cells in the microenvironment. Classically, CD4+ effectors were viewed in the context of the TH1/TH2 paradigm, but other subsets have emerged, including IL-17–producing T cells (TH17) and T cells with regulatory functions (regulatory T cells [Tregs]).49
TH17 cells are a subset of T cells that fills an essential gap in our understanding of inflammatory processes, because it was unclear how TH1 cells actually mediate inflammation in the tissues by the expression of IFN-γ. TH17 cells secret IL-17 (or IL-17A), IL-17F, IL-6, TNF-α, and IL-22. Neutralization of IL-17, but not genetic deletion of TH1 cells, resolves tissue pathology in autoimmune models.50 IL-17 is a potent inflammatory cytokine involved in the recruitment and proliferation of neutrophils. It is also known to induce proinflammatory cytokines, such as TNF-α, IL-1β, and IL-6, as well as chemokines CXCL1, 2, and 8, which together are hallmarks of acute inflammatory processes.51 The chemokines mobilize neutrophil recruitment, which is a characteristic feature of TH17-mediated inflammation. Anti–IL-17 reduces joint destruction in experimental arthritis,52 and decreases neutrophil infiltration in an experimental asthma model while increasing eosinophil infiltration.53,54 Exogenously administered IL-17 reduces pulmonary eosinophil recruitment and bronchial hyperreactivity, suggesting a regulatory role for this interleukin.55 Thus, the TH17-directed neutrophil infiltration is inversely linked to the TH2-mediated eosinophil direction, much like the inverse relationships between TH1 and TH2 cells. In the upper airways, IL-17 has been found in nasal polyps and, interestingly, is expressed in polyps from patients in South China, which have a neutrophil-predominant infiltration, in contrast to polyps from patients from Belgium, which express IL-5 and have an eosinophil-predominant infiltration.56
CD4+ T cells can also differentiate into cells characterized by the ability to suppress T-cell responses and prevent autoimmunity, or regulatory T cells (Tregs).57 Natural Tregs (nTregs) develop in the thymus and constitutively express the high-affinity IL-2 receptor (CD25) in accordance with their high dependency on IL-2 for survival. The transcription factor forkhead box P3 (Foxp3) is also stably expressed by natural Tregs and is required for their suppressive function. Natural Tregs account for 1% to 10% of the CD4+ T-cell population in healthy adult humans, and most investigators identify them from the expression of the preceding factors as CD4+CD25+Foxp3+ cells. The importance of these cells is underscored by the description of patients carrying rare loss-of-function mutations in the Foxp3 gene, in whom a range of autoimmune and inflammatory disorders develops, referred to as immunodysregulation, polyendocrinopathy, enteropathy, X-linked syndrome (IPEX). This syndrome includes type 1 diabetes, autoimmune thyroiditis, eczema, bleeding abnormalities, and chronic wasting. These individuals also demonstrate an increased susceptibility to infection and an elevated incidence of allergic-type symptoms.58 Other Treg populations may develop extrathymically from naïve CD4+ T cells under the influence of transforming growth factor-β (TGF-β) and are referred to as induced Tregs or TGF-β–producing type 3 helper T cells. These cells are Foxp3+ and are functionally indistinguishable from natural Tregs. Treg type 1 cells do not express Foxp3, but they do produce high amounts of the inhibitory cytokine IL-10 and develop outside the thymus under the control of IL-10–conditioned dendritic cells.
Approximately 5% to 10% of T cells in the peripheral circulation, lymph nodes, and spleen are CD4−CD8−. Some of these cells use αβ TCRs and others use γδ TCRs. Double-negative cells do not recognize antigen in the context of MHC class I or II. Some of these cells recognize antigen in the class I–related protein CD1 that is adapted to presentation of glycolipid components of mycobacteria and other microbes.59 A subset of double-negative γδ T cells recognizes the MHC class I−related chain (MIC).60 Both CD4+ and CD8+ T cells differentiate into functionally distinct subsets after exposure to antigen. This process is best described for the transition of CD4+ T cells from naïve to effector populations.61 Resting naïve CD4+
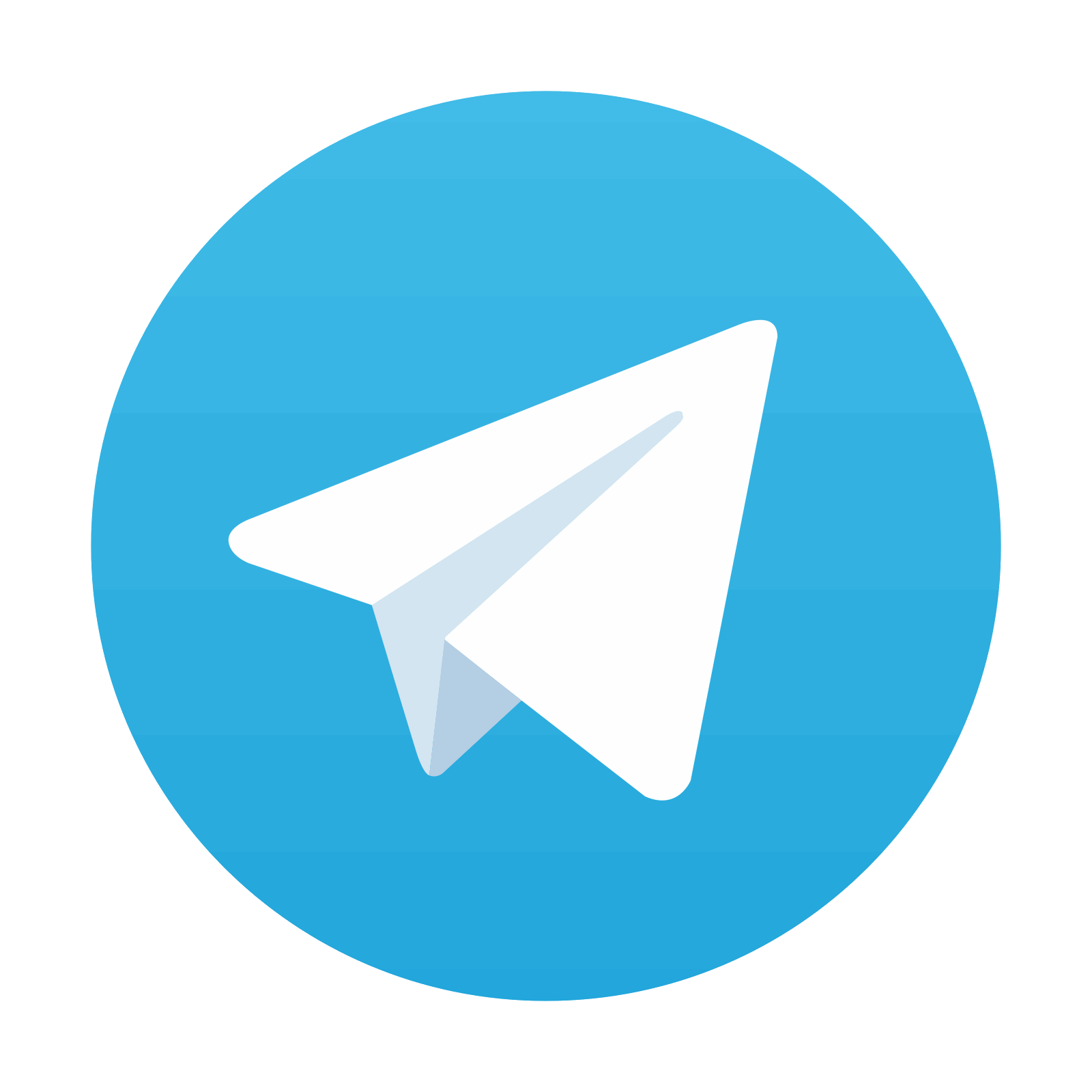
Stay updated, free articles. Join our Telegram channel

Full access? Get Clinical Tree
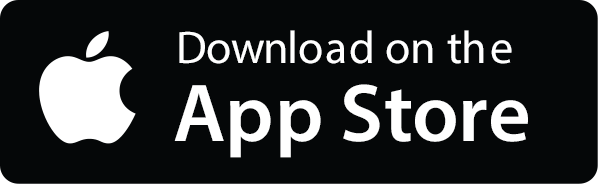
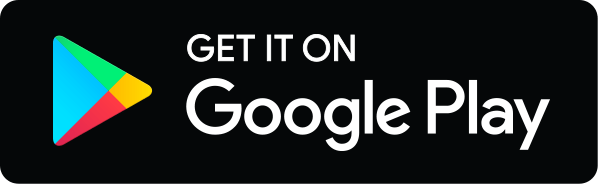