Host Defense against Ocular Infection
Bradley D. Jett
The eye possesses a diverse array of defense mechanisms that protect the visual field from the damaging consequences of infection. The relatively large number of nonspecific and specific defenses (Table 45-1), localized within this relatively small organ system, provides a formidable barrier to bacteria, viruses, fungi, and parasites. In this chapter, current information on the host defenses of the ocular surface and its surrounding tissues, as well as the defense mechanisms that exist in the interior of the eye, is discussed.
Table 45-1. Ocular Host Defense Mechanisms | ||||||||||||||||||||||||
---|---|---|---|---|---|---|---|---|---|---|---|---|---|---|---|---|---|---|---|---|---|---|---|---|
|
The immunologic defenses of the eye, according to the late Dr. J. Wayne Streilein,1 represent a “Faustian Dilemma.” Drawing an analogy between ocular immunology and Goethe’s famous drama, Dr. Streilein explores the deal the eye has made with the devil (the immune system); the eye enjoys just enough protection to limit recurrent infection, but gives up the robust immune responses observed in other body tissues as a means to protect the eye from potentially destructive inflammation and irreversible vision loss through scarring and cellular bystander damage.1 Decades of research have revealed that the eye, like other immune privileged sites, possesses complex mechanisms capable of regulating inflammatory responses. This phenomenon, known as ocular immune privilege, is a dynamic, rather than passive, state mediated by soluble and cellular factors within the ocular microenvironment, and a detailed discussion of this topic is beyond the scope of this chapter. For in-depth background on ocular immune privilege, the reader is referred to several excellent articles.2,3,4,5,6,7 Immune privilege is mentioned in this introduction simply to remind the reader that the immunologic defense mechanisms described herein are also dynamic processes and are susceptible to both activation and suppression.
ANATOMIC AND MECHANICAL DEFENSES
EYELIDS AND EYELASHES
The first line of defense against ocular infection is provided by the eyelids. This mechanical barrier, when closed, protects the ocular surface and its surrounding tissues from exposure to pathogens, allergens, and other foreign bodies. The ocular surface is highly innervated and exquisitely sensitive to mechanical disturbance. Even the slightest contact with the eyelids, eyelashes, or corneal surface elicits the blink reflex. This blinking action serves to wash pathogens and other foreign particles from the ocular surface and to renew the tear film.
Conditions that interfere with normal lid function, including conjunctivitis and keratitis, may render the eye more susceptible to infections.8
TEARS AND LACRIMAL DRAINAGE
The tear film (Table 45-2) serves multiple functions in the nonspecific defense against ocular infection. It bathes the ocular surface and provides a mechanical barrier to adhesion by pathogenic organisms. It lubricates the corneal surface and facilitates the washing action of the eyelids. Bacteria, fungi, foreign bodies, and desquamated epithelial cells are flushed nasally toward the two puncta by this highly efficient washing mechanism.
Table 45-2. The Tear Film | ||||||||||||||||||||||||||
---|---|---|---|---|---|---|---|---|---|---|---|---|---|---|---|---|---|---|---|---|---|---|---|---|---|---|
|
The 7-μm-thick tear film is composed of three layers: lipid, aqueous, and mucoid. These layers are derived from the meibomian glands, the lacrimal glands, and conjunctival goblet cells, respectively.9 Specific antimicrobial components of the aqueous and mucoid layers are discussed later. Lipids confer surfactant properties to the tear film, enhancing its ability to trap microbes and efficiently flush them into the lacrimal excretory system. It is, therefore, not surprising that patients suffering from aqueous tear film deficiencies (i.e., dry eye) are at increased risk for corneal infection.
OCULAR EPITHELIUM
The ocular epithelial surface prevents intraocular penetration by most microorganisms. This nonkeratinized squamous epithelium, consisting of five to six cell layers, is supported by a columnar basal layer. The basement membrane, with its numerous junctional complexes, presents a nearly impermeable anatomic barrier.10 The outermost layer of the corneal epithelium is covered by a layer of glycocalyx over which lies a layer of mucus. This glycocalyx serves as a scaffold that binds mucin, antibodies, and other tear film components. Specific components of the mucoid tear film are discussed later.
Relatively few microorganisms are capable of penetrating an intact corneal epithelium; these include Neisseria gonorrhoeae and Haemophilus aegyptius.8 Even those organisms commonly associated with bacterial keratitis, including Pseudomonas aeruginosa and Staphylococcus aureus, have difficulty establishing infection on an uncompromised ocular surface. Laboratory animal models of bacterial keratitis often require some form of preinoculation corneal injury or abrasion (Fig. 45-1), and/or prolonged contact with the pathogen.11,12 Keratitis caused by yeasts, filamentous fungi, and parasites are almost always associated with corneal abnormalities, injury, or surgery.9 Contact lens wear has also been implicated as a predisposing factor to fungal (predominantly Candida spp.) and parasitic (predominantly Acanthamoeba spp.) keratitis, presumably resulting from microabrasions of the corneal epithelium following prolonged contact with the lens polymers.9 Although little is currently known regarding the molecular mechanism for adhesion and invasion of the cornea epithelium by pathogenic organisms, a reasonable hypothesis may involve the unmasking of previously sequestered host molecules for which pathogens possess specific receptors.13,14 Recently, the laboratory of Suzanne Fleiszig has engineered artificial basement membranes and demonstrated their impermeability to Pseudomonas aeruginosa challenge. However, these scientists also found that the powerful proteases secreted by Pseudomonas were capable of compromising the integrity of the membranes.15
DEFENSES OF THE OCULAR SURFACE AND SURROUNDING TISSUES
OVERVIEW OF OCULAR MUCOSAL IMMUNITY
The hypothetical involvement of extraocular tissues (i.e., conjunctiva, lacrimal system, etc.) in ocular immunologic processes is not a new concept. It has been long recognized that mucosa-associated lymphoid tissue (MALT) constitutes an important component of an organism’s innate and adaptive defense against invasion of mucosal surfaces by pathogens. Specific examples of MALT include gut-associated lymphoid tissue (GALT) found in intestinal Peyer patches and bronchus-associated lymphoid tissue (BALT) of the respiratory tract. MALT, by definition, consists of clusters (follicles) of lymphatic cells situated within and beneath the epithelium of a mucosal surface. These follicles detect antigen and are capable of inducing both cellular and humoral immune responses. MALT follicles possess professional antigen-presenting cells (i.e., macrophages) and are the source of many secreted antimicrobial compounds and cytokines. Specialized vessels within MALT permit lymphocyte recirculation and communication with the central immune system. (For review of mucosal immunology, see references.4,16,17,18,19,20 Mucosal immunity has long been the subject of intense investigation, because these sites represent an important entry point for many pathogens. It is, therefore, somewhat surprising that research into the mucosal immunology of the eye has gained significant momentum only within the past decade.
Several recent histologic, immunohistochemical, and flow cytometric analyses have been published that suggest a role for extraocular tissues in regional mucosal immunity. Studies of conjunctiva-associated lymphoid tissue (CALT) revealed the presence of discreet follicles with germinal centers, close interactions between lymphocytes and surface lymphoepithelium, layers of IgA+ plasma cells in the lamina propria, and high endothelial venules (HEVs).20,21,22 Similar studies involving lacrimal drainage-associated lymphoid tissue (LDALT) and lacrimal gland-associated lymphoid tissue (LGALT) have revealed the presence of IgA+ plasma cells, T lymphocytes, major histocompatibility complex (MHC) class II-positive cells, and distinct lymphoid follicles in close association with HEVs.20,23,24 Because of the apparent biochemical and anatomic integration of lacrimal gland, lacrimal drainage, and conjunctival immune functions, these sites are collectively referred to as eye-associated lymphoid tissue (EALT).20,23 The various humoral and cellular components of the ocular mucosa, which serve both protective and regulatory functions, are discussed in greater detail later.
NORMAL OCULAR MICROFLORA
Colonization of the normal ocular surface and surrounding tissues by microorganisms is a dynamic phenomenon in both quantity and identity of microbes. Although samples taken from the conjunctiva of healthy individuals may be negative for microorganisms on culture, it is generally accepted that most humans harbor at least some normal bacteria on their periocular tissues. Among the normal ocular flora, Staphylococcus sp, Corynebacterium sp, and anaerobic Propionibacterium sp are most frequently isolated.19,25,26 Using 16s ribosomal DNA analysis of normal human conjunctival samples, Graham et al27 recently identified DNA from several species including Bacillus, Rhodococcus, and Klebsiella. However, in these studies coagulase-negative staphylococci were the only cultivatable bacteria.27 Most fungi isolated from the conjunctiva are thought to be airborne contaminants; and protozoans and helminths are not considered to be normal ocular flora.
The diverse spectrum of normal ocular isolates and the intricacies of colonization dynamics are beyond the scope of this chapter. Nevertheless, normal ocular bacteria may serve a protective role in inhibiting colonization by more pathogenic species. This phenomenon of competitive exclusion has been well studied at other anatomic sites, including the nares, skin, and gastrointestinal tract. Presumably normal ocular organisms may play a similar role; however, a direct relationship between ocular commensal colonization and pathogen inhibition has remained elusive. The normal flora described previously are ironically the most frequent causes of many ocular infectious diseases, which demonstrates the delicate balance of host–parasite relationships in the external ocular microenvironment.
MUCINS
The innermost layer of the preocular tear film predominantly consists of glycoproteins known as mucins. (For review, see references.28,29) Ocular mucins are thought to serve a protective role; the mucins trap pathogenic microorganisms until they are swept to the inner canthus by the blinking action of the eyelids. Mucins, by their nature, may also serve as a matrix within which secretory IgA and other antibacterial factors are concentrated (Fig. 45-2).
Mucin glycoconjugates are large (3 × 105 to >4 × 107 kilodaltons [kDa]) and contain many O-linked oligosaccharide side chains, which comprise approximately three fourths of their dry weight.30 Mucins are expressed by most specialized epithelial tissues of mucosal surfaces, and at least 10 membrane-associated mucin genes have been identified in humans.31 Because of their heterogeneous nature and high carbohydrate content, mucins are inherently difficult to purify and analyze. Results from multiple groups using techniques of glycoprotein purification, anti-mucin antibody reactivity, and reverse transcriptase-polymerase chain reaction (RT-PCR) have provided evidence that the preocular tear film may primarily consist of MUC1, MUC4, and MUC16.30,32 Mucins are generally thought to be produced by stratified epithelial cells of the ocular surface and conjunctiva and by conjunctival goblet cells.
Abnormal expression, distribution, and/or glycosylation of ocular mucins are thought to be major contributing factors to the pathophysiology of dry eye syndrome.33,34 More unclear, however, is the role of mucins in innate immunity to ocular infectious diseases. The results of a study using genetically engineered MUC1-null mice suggested that this mucin plays a protective role against bacteria-induced inflammation.35 Recent findings from studies using RNAi-mediated MUC16 silencing31 and chemically-based mucin blockers36 indicate the importance of mucins in preventing bacterial adherence to corneal epithelial cells. The exact role of mucins in protection against ocular infection remains to be determined.
LYSOZYME
Lysozyme is a low-molecular-weight protein (15 kDa) that demonstrates bacteriostatic and bactericidal activity against a wide range of primarily gram-positive bacteria (i.e., Streptococcus, Staphylococcus, etc.). It facilitates the breakdown of bacterial cell wall peptidoglycan by hydrolyzing glycosidic bonds between N-acetylmuramic acid and N-acetylglucosamine.19 Lysozyme is one of the major components of tears, accounting for approximately 30% to 40% of total tear protein, and is most likely synthesized by acinar cells of the lacrimal gland.19,37 Because gram-negative organisms (i.e., Escherichia coli, Pseudomonas, Salmonella, etc.) possess an outer lipid bilayer with high concentrations of lipopolysaccharide, they are generally more resistant to lysozyme compared with gram-positive organisms. Nevertheless, lysozyme may potentiate the antibacterial activity of complement in conjunction with secretory IgA.
PHOSPHOLIPASE A2
Although lysozyme has traditionally been viewed as the principal antibacterial substance of the tear film, recent findings indicate that phospholipase A2 may actually represent the most active component. Phospholipase A2 is found in mucous secretions at other body sites and is known to be produced by leukocytes; its synthesis by cells within the main and accessory lacrimal glands has been suggested.37 The concentration of phospholipase A2 in human tears was determined to be approximately 30 μg/mL, corresponding to 1,000 × and 30,000 × the concentration sufficient to kill S. aureus and Listeria monocytogenes, respectively.38,39
Unlike lysozyme, which attacks the peptidoglycan cell wall, phospholipase A2 directly attacks the phospholipids of bacterial membranes. This low-molecular-weight enzyme is less active against gram-negative bacteria, perhaps because of the nature of their double lipid bilayers. Until recently, it was unclear whether tear film phospholipase A2 was necessary and/or sufficient for bactericidal activity against gram-positive pathogens. Using radiolabeled S. aureus in a rabbit model, Moreau et al40 demonstrated that tear phospholipase A2 cleaved bacterial membranes, releasing arachidonic acid. They also demonstrated that phospholipase A2 could be blocked by polyamine inhibitors (i.e., spermidine). More research is clearly warranted into this potentially important antibacterial factor, including its role and molecular mechanism in the human tear film.
β-LYSIN
The presence of the antibacterial factor β-lysin in tears has been suggested for several decades. Its spectrum of activity is similar to that of lysozyme, but it tends to also be active against organisms that are less susceptible to lysozyme. The target of β-lysin is generally considered to be the bacterial lipid membrane, and β-lysin is known to act synergistically with lysozyme.41 Synthesized primarily by platelets, its presence in the ocular tear film is thought to be owing to leakage from the serum. In fact, its presence in the ocular tear film has been questioned.42 Confusion surrounding the expression, spectrum of activity, and identity of ocular β-lysin may stem from its inclusion within a larger family of small (<8 kDa) cationic platelet-derived microbicidal proteins, which are a somewhat heterogeneous group of defensin-type molecules with diverse mechanisms of action.43 As protein micropurification techniques become more sophisticated, perhaps the understanding of the expression and/or role of β-lysin in the eye will become more clear.
METAL CHELATORS
Lactoferrin is an 82-kDa iron-binding protein found in the human tear film. It is a major constituent of human tears (approximately 25% of total tear protein), and its expression has been observed in cells lining the nasolacrimal system.44 This multifunctional protein has been shown to enhance natural killer (NK) cell activity and to regulate complement activation.19 Its antimicrobial properties are likely owing to its ability to reduce the amount of free iron, which is an essential element for many pathogens.8 Lactoferrin was capable of interfering with herpes simplex virus-1 (HSV-1) infection of murine corneal epithelial cells both in vitro and in vivo. Once infected, however, lactoferrin had little effect on viral replication, suggesting that it may compete for viral binding sites on the host cell.45 Human immunodeficiency virus (HIV)-positive patients demonstrated tear lactoferrin deficiency and concomitant increases in levels of bacterial colonization of periocular tissue.46 The role of lactoferrin in the tear film has recently been reviewed.47
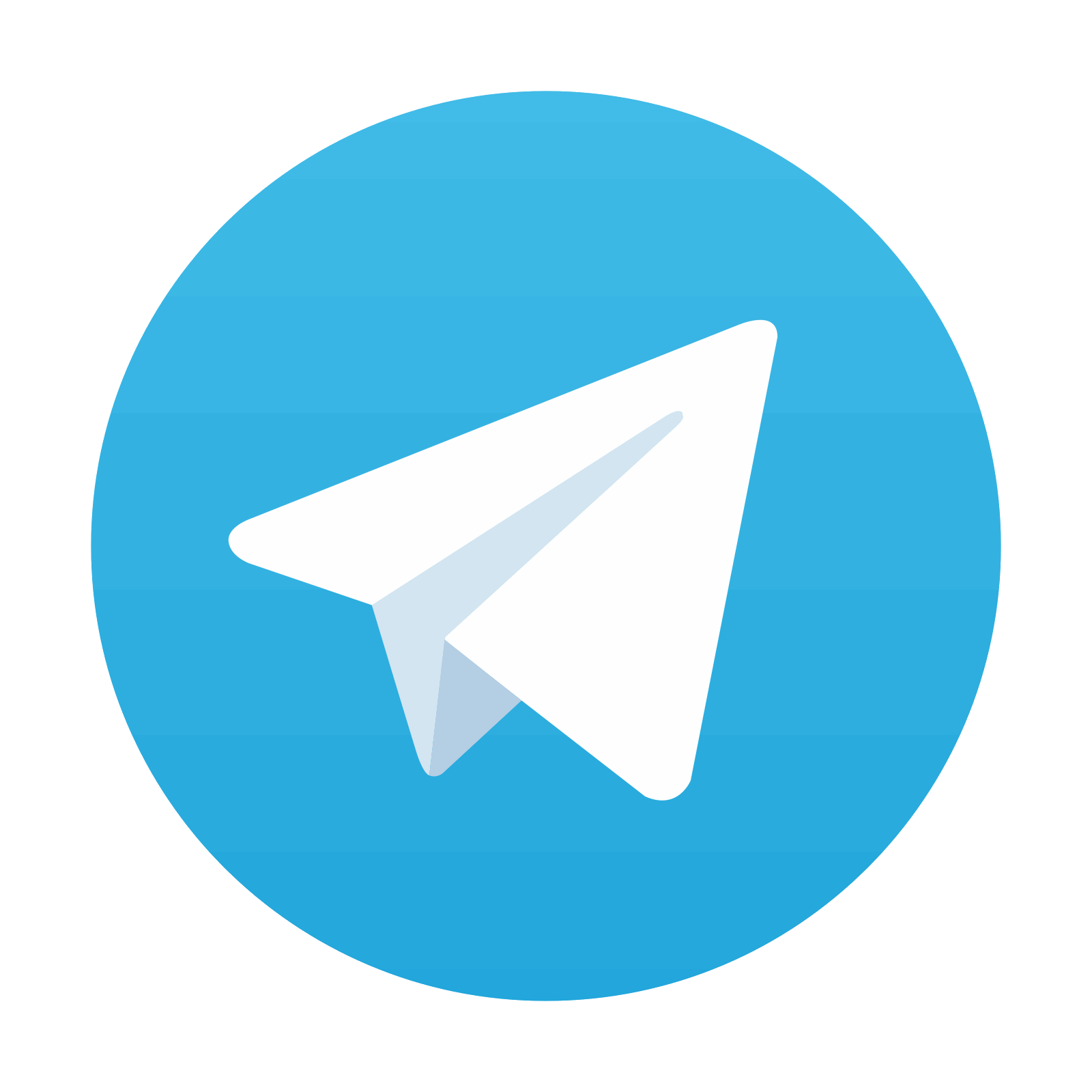
Stay updated, free articles. Join our Telegram channel

Full access? Get Clinical Tree
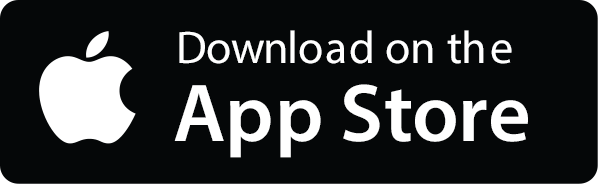
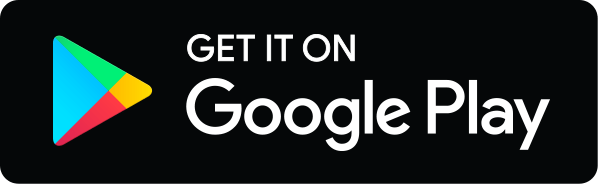