Functional Morphology of the Trabecular Meshwork
Elke Lutjen-Drecoll
Johannes W. Rohen
Anatomy
The chamber angle is formed by the iris root; the connective tissue, in front of the ciliary body (ciliary body band); and the trabecular meshwork (TM), up to Schwalbe’s line (Fig. 1). In the human, Schlemm’s canal is situated within the sulcus sclerae. Posteriorly the sclera protrudes inward by forming the wide, wedgelike scleral spur where the anterior ciliary muscle tips end and most of the TM begins (so-called corneoscleral portion). The inner part of the TM is fixed to the connective tissue in front of the ciliary muscle and to the iris root and is continuous posteriorly with the uvea (so-called uveal part of the TM). Internal to the uveal meshwork, radially oriented tissue strands are seen that run from the iris root up to the cornea or to the TM. These interconnected strands represent remnants of the pectinate ligament, which is well developed in most mammalian eyes (iridial meshwork). Adjacent to Schlemm’s canal a loosely arranged meshwork is formed, which is rich in cells and extracellular material and is termed the cribriform layer (juxtacanalicular tissue, or endothelial meshwork). Since Schlemm’s canal is smaller in the anteroposterior direction than the entire distance from scleral spur to Schwalbe’s line, one can differentiate between a filtering and a nonfiltering portion of the TM (see Fig. 1).
Trabecular Meshwork and Site of Aqueous Outflow Resistance
Aqueous humor leaves the anterior chamber mainly by way of Schlemm’s canal after having passed through the TM. From Schlemm’s canal, the fluid is drained into 25 to 35 collector channels that are connected to the intrascleral and episcleral venous plexus.1,2 Some fluid also penetrates into the ciliary body, leaving the eye through the choroid and sclera (so-called uveoscleral flow) (Fig. 2).3,4
To maintain an intraocular pressure (IOP) of around 17 mm Hg against a venous pressure of 7 to 9 mm Hg, the outflow tissues have to provide the necessary resistance. There has been a protracted debate as to the exact anatomical location of the outflow resistance. From experimental studies on enucleated human and monkey eyes it can be concluded that most of the resistance is located internally to Schlemm’s canal in the TM, presumably in the cribriform or subendothelial region of Schlemm’s canal.5,6
Scanning electron micrographs of serial layers through the entire meshwork and tangential sections (parallel to the inner wall of Schlemm’s canal) reveal that the form of the trabecular lamellae and of the intertrabecular spaces changes markedly from the inner to the outer portions of the meshwork. The innermost iridial meshwork consists mostly of long, radial, interconnecting strands forming an irregularly arranged network with rather large openings (Figs. 3 and 4). In the uveal portion, flat sheets are evident that are still relatively irregularly arranged but frequently interconnect with each other. The holes within the uveal meshwork are somewhat smaller than in the iridial meshwork but still show diameters varying between 10 and 30 μm.7
Toward Schlemm’s canal the corneoscleral meshwork is more expanded and forms broad lamellae that run predominantly in an equatorial direction (Fig. 5). The trabecular lamellae interconnect with each other and are regularly arranged. The intertrabecular spaces appear now as elongated rhomboid-like pores, the long diameter of which averages 10 to 20 μm.7
Morphology of the Trabecular Lamellae
The simple trabecular lamellae or beams show more or less the same general architecture. Each lamella possesses a central core of densely packed collagen fibers running predominantly in an equatorial direction (Figs. 6 and 7). The trabecular lamellae are normally completely covered by trabecular cells that rest on a basement membrane. Often the trabecular cells bridge the intertrabecular spaces and therefore serve as a covering for two adjacent lamellae. The cells also have cytoplasmic extensions that are connected with similar processes of cells situated on neighboring lamellae. Cellular interconnections and cytoplasmic bridges establish a three-dimensional cellular network (Figs. 6 and 7). Adjacent trabecular cells are firmly connected to each other by desmosomes. Gap junctions allowing tonal coupling between the trabecular cells are also present.8 However, tight junctions are lacking and as a consequence tracers such as cationic ferritin freely penetrate into the central core of the trabeculae (Fig. 8).9
The central core of the trabecular lamellae contains numerous collagen and elastic fibers embedded in a homogeneous ground substance rich in hyaluronan and proteoglycans.10,11,12 The elastic fiber net of the corneoscleral meshwork is continuous posteriorly with that of the scleral spur and anteriorly with that of the sulcus sclerae. In the uveal meshwork the elastic fibers are continuous posteriorly with the elastic fibers that are located within the connective tissue between the ciliary muscle tips and in front of the circular muscle portion.13
The elastic fibers differ in structure and composition from most of the elastic fibers in the body so that they were termed elastic-like fibers.14 Ultrastructurally, they reveal an electron-dense core sparsely intermingled with electron-lucent material (Figs. 9 and 10A). These fibers are surrounded by a more or less homogeneous sheath. Treatment with pancreatic elastase (Fig. 10B)14 and immunohistochemical studies using antibodies against α-elastin and tropoelastin15 revealed that only the relatively small electron-lucent area in the central core of the elastic-like fibers contains elastin and its soluble precursor tropoelastin. These components are embedded in electron-dense material of yet unknown nature. The sheaths consist mainly of elastic microfibrils and collagenous fibrils, embedded in proteoglycans that can be digested by chondroitinases.14 In infant eyes, the sheath is very small, whereas in adult eyes the sheath is well developed and becomes continuously thicker with increasing age.10,14,15 In the adult the fibrous material of the sheath consists mainly of the so-called curly collagen (lattice collagen or long-spacing collagen). Type VI collagen fibers pass into this sheath material16 (Fig. 11).
![]() FIGURE 11 Note that the fine fibrils entering the sheath of the elastic fibers are immunogold labeled for type VI collagen. |
The collagenous fibers found in the central core of the corneoscleral lamellae show the normal periodicity of 64 to 68 nm, and clusters of long-spacing collagen have also been found in these regions. According to immunohistochemical studies of Murphy and coworkers,17 there is a pronounced staining for type III and type I collagen in the trabecular lamellae.
The basement membranes of the lamellae that stain for type IV collagen and laminin are continuous anteriorly with the basement membrane of the corneal endothelium.10,17,18,19,20
Criborm Layer and Aqueous Outflow Resistance
In contrast to the corneoscleral meshwork, the cribriform layer does not show a regular organization of trabecular lamellae and beams but the cells are distributed within the extracellular material. The material consists of an irregularly arranged network of fine fibrils, ground substance, and an elastic-like fiber system. In histologic sections oriented in a tangential plane, the fibrous network appears to be a mesh surrounding many holes or openings of different sizes (Fig. 12). This was the reason the term cribriform meshwork was introduced.21
Serial tangential sections showed in the electron microscopic dimension that the fiber network belongs to a complicated system that connects the inner wall endothelium with the trabecular lamellae. The elastic-like fibers of the cribriform layer form a regularly arranged network of interlacing fibers that are continuous with the elastic-like fibers of the adjacent corneoscleral lamellae (Fig. 13). Toward the inner wall endothelium, bundles of fine fibrils separate from the elastic-like fiber sheaths and connect the subendothelial network of elastic-like fibers either with the endothelium of Schlemm’s canal or with the subendothelial basement membrane material. Therefore, these fibrils were called “connecting fibrils” (Fig. 14).22 In the adult eye, the connecting fibrils are embedded in a homogeneous ground substance that is digestible with chondroitinases.14 Connecting fibrils also connect cribriform trabecular cells with the cribriform elastic network. In regions of attachment, the cribriform cells similar to myofibroblasts form basement membrane like material.23
![]() FIGURE 13 Electron micrograph of a tangential section through the inner wall of Schlemm’s canal showing the subendothelial elastic-like fiber network (arrows) of the cribriform layer (× 10,000). |
Electron microscopic investigations reveal that there are optically empty spaces between the connecting fibrils. In an experimental study on monkey eyes, we found that the area of such “empty spaces” in the cribriform region is positively correlated with outflow resistance values measured by anterior chamber perfusion.24 We therefore assumed that these spaces may represent preferential pathways for aqueous outflow through the cribriform layer. Calculations about the pressure gradient across the cribriform and subendothelial layers lead to the statement that, if the spaces were really empty, the extracellular material and cells in this region might provide only insignificant resistance to aqueous outflow.25,26 If, however, the preferential pathways are coated with a 0.35-μm thick glycosaminoglycan layer, the pressure gradient would rise to the physiologically observed values. It is possible that hyaluronan, glycosaminoglycan or proteoglycan gel—lost during the embedding procedure—also contribute to formation of outflow resistance.27 This hypothesis was supported by experimental studies in the living monkey where in fact 80% to 90% of outflow resistance was shown to be located in front of Schlemm’s canal, probably within the cribriform layer of the TM.6 But even if the knowledge about the composition of the extracellular material in the subendothelial region of Schlemm’s canal has increased,28 the exact site of aqueous humor outflow resistance is still not known.
Inner Wall of Schlemm’s Canal
The endothelial lining of the canal consists of a complete monolayer of flat endothelial cells staining for vascular endothelial markers.29 Unlike other vascular endothelium, the endothelial cells of Schlemm’s canal do not rest on a complete basement membrane. A basement membrane is formed initially during embryonic development, but it gradually disappears as aqueous circulation starts.30,31 In its final form Schlemm’s canal is more comparable with a lymphatic than a blood vessel. Lymphatic vessels do not usually possess basement membranes. Their endothelial lining is perfused from outward to inward and often connected with elongated cytoplasmic processes of adjacent pericytes. As in lymphatics the endothelial cells of the inner wall of Schlemm’s canal also develop cytoplasmic processes that interdigitate with similar processes of underlying cells of a second row underneath the canal endothelium (Fig. 15). The subendothelial cell layer is not complete and consists of elongated, starlike cells oriented predominantly in a radial anteroposterior direction. In contrast, the endothelial cells of Schlemm’s canal measuring about 160 μm in length and covering an area of 408 μm2 run mostly in an equatorial direction.32 The cellular and fibrillar connections with the cribriform layer may stabilize the inner wall during IOP variations and changes in the perfusion rate. The double-layered structure of the inner wall is often seen to be elevated and protrudes into the lumen of the canal when IOP is particularly high at the time of tissue fixation. If the IOP is low, the two cell layers are pressed together from the luminal side, thus presumably preventing a reflux of blood into the TM and anterior chamber.33,34
If the IOP is slightly elevated, only the endothelial cells bulge into the lumen of the canal, thus forming large vacuoles, usually called “giant vacuoles.” Since Holmberg35 first described these “giant vacuoles” within the inner-wall endothelium of Schlemm’s canal, the discussion about their functional significance has never been resolved.36 Many of the giant vacuoles communicate with the subendothelial space by way of an often large opening, but very few communicate with the lumen of Schlemm’s canal. If there is a communication with the canal, it is usually by a small pore on the canal side of the cell.37 When serial sections are employed it is possible to demonstrate that some “vacuoles” have openings on the inner and outer sides, thus forming transcellular microchannels. Tripathi36,38 proposed that the endothelial cells lining Schlemm’s canal have the ability to transfer aqueous humor in bulk by a cyclical pressure-dependent process that starts initially as an invagination from the trabecular side and proceeds to the formation of a transcellular channel by a perforation in the outer membrane of the vacuole. This hypothesis has, however, not yet been verified. On the other hand, it is now generally accepted that both the frequency and size of giant vacuoles are proportional to the pressure in the anterior chamber at the time of fixation.39 Nearly 20,000 pores have been counted at the luminal side of the inner wall endothelium in human eyes. Therefore, as calculations showed, only 5% to 10% of the entire resistance can be localized within the endothelial lining of human eyes.37,40 Since occasionally, ground substance or particles are found within the giant vacuoles, the transcellular channel system may provide a mechanism by which extracellular material can be “cleared” or “washout” from the cribriform layer. Inomata and associates41 have demonstrated in cynomolgus monkeys that particles suspended in gelatin solution pass through the transcellular microchannels of the endothelial lining as do erythrocytes if perfused through the anterior chamber.
The endothelial cells of Schlemm’s canal are bound together by maculae adherentes and tight junctions.8,39 However, these tight junctions do not form zonulae occludentes but in places the adjacent cell membranes are separated, forming meandering pathways between interdigitating cell processes. These pathways are often open toward both the cribriform layer and the lumen of the canal (Fig. 16). Anterior chamber perfusion with cationized ferritin in rhesus monkeys has shown that these particles predominantly pass through the paracellular routes.8,9 Often these routes are locally expanded, so that in sagittal sections “vacuole-like” structures are formed.9 According to our observations, macrophages and leukocytes mostly pass through the paracellular routes to leave the eye rather than through the transcellular channels (Fig. 17).
Connections of the Trabecular Meshwork with the Ciliary Muscle
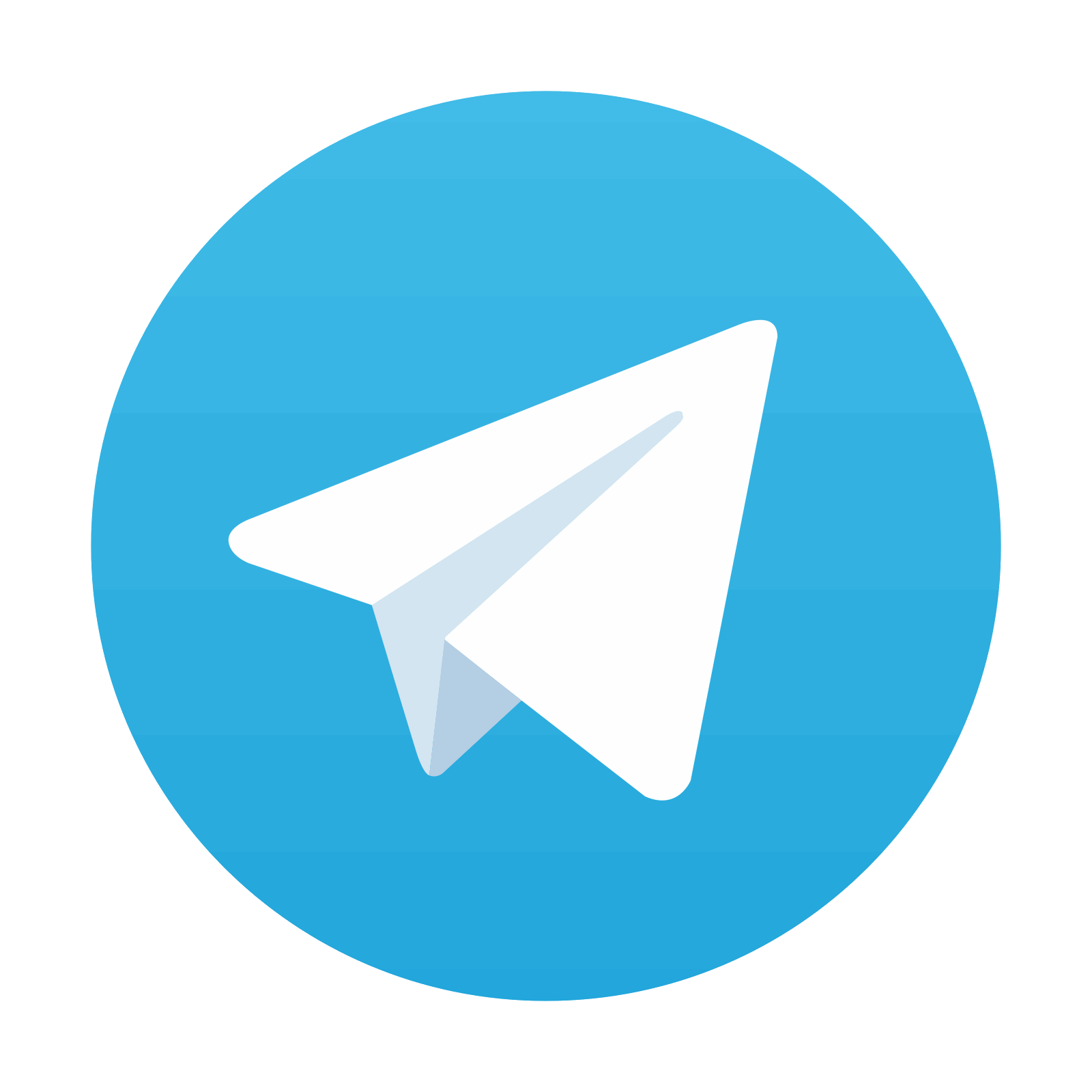
Stay updated, free articles. Join our Telegram channel

Full access? Get Clinical Tree
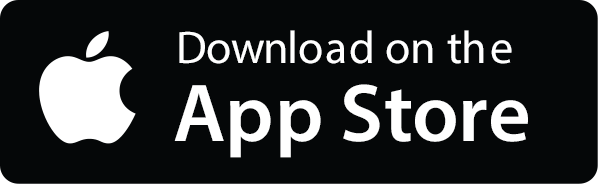
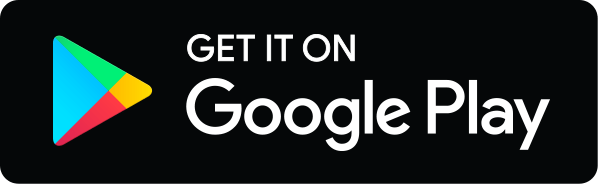