Vitamins
Joel S. Mindel
In 1994, the United States Congress excluded the Food and Drug Administration (FDA) from regulating advertisements for vitamins, minerals, and food supplements. These substances were placed under the control of the Federal Trade Commission (FTC), which does not provide the same level of scrutiny as does the FDA. However, the FDA continues to maintain control over both the container labeling and the claims made in the package inserts. This dichotomy created no major inconsistencies for patients or ophthalmologists until the AREDS-8 study in 2002.1
In 2002, the FTC permitted the pharmaceutical company that cosponsored (with the National Eye Institute) the AREDS-8 study to run advertisements claiming a benefit for a multivitamin and mineral combination used to treat macular degeneration. The American Academy of Ophthalmology has taken a position in support of multivitamin use in treating macular degeneration. However, the FDA does not find these claims to be justified by the scientific evidence. So, although newspapers carry advertisements claiming, “The only eye vitamin proven effective by the National Eye Institute,” the FDA package insert information continues to state, “This product is not intended to diagnose, treat, cure, or prevent any disease.” The section on Multivitamins deals with this controversy.
Vitamin A
Vitamin A (retinol) is a fat-soluble alcohol important for corneal and retinal function. Humans ingest preformed vitamin A or its precursors, carotenoids. (-Carotene is the most common and most active of the carotenoids. One international unit (IU) of (-carotene is defined as having the same activity as one IU of vitamin A.
Vitamin A is absorbed from the intestine and stored in the liver in various ester forms (e.g., retinyl acetate). Vitamin A normally is transported in the blood while bound to a specific protein; one molecule of retinol is bound to one molecule of retinol-binding protein. The pigment epithelium may store vitamin A.2 The human retina has two different receptors for vitamin A.3 Once in the retina, vitamin A aldehyde (retinal) is incorporated into the visual pigments. Its active form here is in the 11-cis configuration. When light strikes the retina, the 11-cis form is converted to the trans form, and the latter is released by the photoreceptors to enter the pigment epithelium cells. Here it is reconverted to the cis form. This shuttling of vitamin A between photoreceptors and pigment epithelium occurs via a glycoprotein, interstitial retinol-binding protein, which is synthesized by the photoreceptors.4 Vitamin A aldehyde is irreversibly oxidized to vitamin A acid (retinoic acid). Different intracellular proteins bind retinol and retinoic acid, suggesting that each has a different physiologic role. Retinoic acid can partially overcome systemic vitamin A deficiency, but it will not correct the retina’s dysfunction. Bowling and Wald observed that vitamin A acid allowed rats to grow normally, although their retinas continued to deteriorate.5
The suggested daily requirement for vitamin A has been revised downward because of the known toxicity of retinoids in early pregnancy. The recommended intake for adults is the equivalent of 1,200 IU trans-(-carotene for men and 1,000 IU trans-(-carotene for women.6
Deficiency
Vitamin A deficiency manifests itself in the eye in two ways: night blindness and xerophthalmia. The lower limits of normal vitamin A are approximately 65 IU/100 mL in plasma and 135 IU/g in liver. Night blindness usually occurs before xerophthalmia. In night blindness, a reduction in the electroretinogram occurs, ultimately causing death of the photoreceptor cells. Vitamin A deficiency in the rat has been shown to decrease the rate of rod outer segment synthesis.7 The rate of recovery following vitamin A therapy is variable. It is usually rapid. Brown and coworkers reported a 33-year-old man with night blindness following intestinal bypass surgery.8 Initially, his electroretinogram was markedly reduced and his visual field constricted to 10 degrees. Three days after beginning oral therapy with 25,000 IU vitamin A, four times a day, the patient’s electroretinogram, dark adaptation curve, and visual fields were normal. However, it took 7 months from the time of receiving 500,000 units of vitamin A parenterally for another patient9 with a deficiency from intestinal bypass surgery to regain normal rod sensitivity, dark adaptation, and scotopic ERG responses.
Xerophthalmia, or dryness of the eye, also can lead to loss of vision. The dryness is due to defective growth and differentiation of conjunctival and corneal epithelial cells. A marked decrease occurs in the synthesis of glycoproteins and glycosaminoglycans.10 Keratinization and punctate epitheliopathy occur first, followed by stromal edema and keratomalacia. Vitamin A therapy results in an increased rate of glycosamine incorporation into glycoproteins.11,12 Mucus-secreting goblet cells are reduced markedly in number in xerophthalmia, but their absence may not be a key factor. The cornea begins to heal 1 to 4 days after starting systemic vitamin A therapy, even though conjunctional goblet cells cannot be found for more than 1 week.13
Vitamin A deficiency is a major problem in underdeveloped parts of the world. An especially harmful synergy is created when children with hypovitaminosis A develop measles (rubeola); preexisting xerophthalmia can progress to corneal ulceration and so-called “measles blindness”.14 In developed countries, vitamin A deficiency usually is associated with alcoholism, malabsorption conditions,15 or extreme diets.16
The British Medical Research Council found that 1,330 IU of vitamin A restored scotopic vision in depleted subjects, but, because the serum and liver vitamin A levels remained low, it suggested a minimum adult daily allowance of 2,500 IU.17 When 200,000 IU (60 mg) vitamin A is given orally as a single dose every 6 months to children 1 to 4 years of age, satisfactory serum levels are obtained in areas where deficiency is prevalent.18
Therapy for night blindness and xerophthalmia usually begins with the intramuscular administration of vitamin A, 100,000 IU a day for 1 or 2 days. Care must be taken to avoid inducing toxicity by overdosing. Intramuscular therapy has been preferred because of the fear that the diarrhea and low serum protein that often accompany this deficiency would impair oral absorption. The latter is due to a generalized nutritional deficiency associated with hypovitaminosis A, and patients may have inadequate retinol-binding protein. Sommer and coworkers 19 treated children with either 200,000 IU oil-miscible vitamin A orally or 100,000 IU water-miscible vitamin A intramuscularly; both groups received a second dose of 200,000 IU, orally, the next day. No difference in their responses was noted, even though the serum levels were significantly higher during each of the first 7 days following intramuscular treatment. With either treatment, improvement or recovery occurred in 95% of corneas within 6 to 8 days. When children with diarrhea or hypoproteinemia, defined as <3 g albumin/100 mL serum, were analyzed, there was still no difference noted using either form of treatment. The reason appeared to be that only vitamin A bound to retinol-binding protein was therapeutic. Both oral and intramuscular treatment saturated the carrying capacity of this protein. A single, oral dose of 200,000 IU improved 40% of corneas within 3 days and produced complete resolution within 4 weeks.20 Those few eyes that had a delayed or transient response to vitamin A appeared to be in patients with severe generalized protein deficiency, irrespective of the serum retinol-binding protein level.21
Smaller doses are effective if given for a prolonged period. A patient with a serum vitamin A level of 20 IU/100 mL had a conjunctival biopsy demonstrating total absence of goblet cells. After 3 weeks of 25,000 IU/day oral therapy, his serum vitamin A level was 150 IU/100 mL. Goblet cells and reduced keratinization were evident in the conjunctival biopsy. After 8 weeks of treatment, his serum vitamin A level was 210 IU/100 mL, and the epithelial structures had returned to normal.22 In the past, the presence and disappearance of Bitot spots have been assumed to be synonymous with, respectively, the presence and correction of a vitamin A deficiency. However, occasionally, Bitot spots represent a permanent alteration in the conjunctiva and, as such, do not correlate with the patient’s vitamin A nutritional status.23,24,25
Because corneal disease may not respond for several days to systemic treatment, concomitant topical therapy has been investigated. Pirie treated rat xerophthalmia with topical retinoic acid and obtained good results.26 Sommer and Emran treated eight children unilaterally with up to 10 drops/day of 0.1% retinoic acid in arachis oil;27 the children also received systemic vitamin A therapy. Improvement was believed to occur more rapidly in the eyes receiving topical therapy. However, Bors and Fells reported minimal improvement in patients given vitamin A eye drops.28 For example, one patient with low serum vitamin A (13 IU/100 mL), conjunctival xerosis, and corneal ulcers was given vitamin A eye drops, 150,000 IU/mL, every 2 hours for 2 days. Photophobia and ocular discomfort were reduced, but it was not until 3 to 4 days after receiving 200,000 IU vitamin A intramuscularly that dramatic improvement occurred.
Infants fed a diet deficient in vitamin A can develop hydrocephalus and a syndrome mimicking the pseudotumor cerebri of vitamin A toxicity.29,30 Increased intracranial pressure, bulging of the fontanelles, and separation of the cranial sutures occurs. Calves fed a diet deficient in vitamin A also will develop increased cerebrospinal fluid pressure.31
Retinitis Pigmentosa
Several findings suggest that abnormal retinoid metabolism may play a role in the etiology of retinitis pigmentosa: Both vitamin A deficiency and retinitis pigmentosa produce reduced electroretinogram responses and night blindness; an esterification defect of vitamin A has been identified in pigment epithelial cells of RCS rats with inherited retinal dystrophy;32 and coadministration of vitamins A and E seems to retard the progression of the retinitis pigmentosa seen in abetalipoproteinemia.33 Gouras and coworkers found that two patients with abetalipoproteinemia had serum vitamin A deficiencies, with levels below 67 IU/100 mL, despite apparently normal diets.34 The electroretinograms and vision of both patients were impaired. Oral vitamin A 200,000 IU, one dose to one patient and two doses to the other patient, reversed these changes. Cone function recovered more rapidly than rod function.
However, not all the data support a therapeutic role for vitamin A. Vitamin A deficiency does not have the ophthalmoscopic picture of retinitis pigmentosa, although small defects in the pigment epithelium and retina have been reported;28,35 these lesions disappeared 2 to 3 months after therapy was begun. Nor do patients with retinitis pigmentosa have vitamin A and (-carotene serum levels significantly different from those of control groups.36,37 Raki had found small and probably not significant reductions in the retinol-binding protein levels of patients with retinitis pigmentosa.38 In a more exhaustive study of various genetic forms of retinitis pigmentosa (i.e., autosomal recessive, autosomal dominant, sex-linked recessive, Lawrence-Moon-Bardet-Biedl syndrome, Usher syndrome, and Winkelman syndrome), the levels of carrier protein were not significantly different from normal controls.39
In a masked study, Chatzinoff and coworkers concluded that vitamin A therapy was of no value in retinitis pigmentosa.40 Twenty-seven patients received 11-cis-vitamin A and 30 “control” patients received trans-vitamin A for 3 years. Both groups received twice weekly intramuscular injections of 100,000 IU of their respective drugs. Seventy-eight percent of the group receiving 11-cis-vitamin A had an additional loss of vision of one or more lines and/or progressive visual field loss, compared with 57% of the group receiving trans-vitamin A.
Retinitis pigmentosa patients were divided into four groups that received combinations of daily oral vitamin A palmitate 15,000 IU or 75 IU and vitamin E 3 IU or 400 IU.41 There were 596 patients followed for 4 years, and 261 patients followed 6 years. An ERG benefit was found for the group receiving vitamin A 15,000 IU plus vitamin E 3 IU, but no benefit was noted in terms of visual acuity or visual fields. The recommendation that this limited response justified vitamin A use has been criticized.42,43
Cataract
A single measurement of plasma β-carotene in 372 volunteers suggested that a higher carotenoid level might protect against the development of nuclear cataracts.44 A randomized, masked placebo-controlled trial was performed on male physicians using alternate-day therapy over a 20-year period. 5,244 subjects received 50 mg β-carotene, 5,251 subjects received a placebo, 5,231 subjects received 50 mg β-carotene and 325 mg aspirin, and 5,242 subjects received 325 mg aspirin.45 Subjects were followed a mean of 12 years. No overall benefit to therapy in terms of cataract incidence or rate of cataract surgery was noted. Among the subgroup of smokers, β-carotene appeared to lower the increased risk of developing cataracts by 25%. However, no recommendation was made because of two prior studies showing an increase in lung cancer in smokers receiving β-carotene.46,47
Healing
Corneal epithelial healing occurred more rapidly in rabbits treated for 3 days with topical vitamin A acid ointment 0.1% or 1% twice a day.48 When herpes simplex keratitis was stimulated by subconjunctival injections of triamcinolone acetate, rabbits receiving multiple intraperitoneal injections of 100,000 IU vitamin A just before and up to 15 days after applying the virus had significantly less severe epithelial and stromal disease.49 Corneal endothelial cell migration also appeared to be stimulated by retinoic acid.50 Trans-retinoic acid appeared more effective than cis-retinoic acid.51
Based on these findings, topical trans-retinoic acid (tretinoin), 0.01% to 0.1% in arachnis oil or ointment, and retinol palmitate were tested in a variety of human disorders characterized by squamous metaplasia and keratinization of the conjunctival epithelium: keratoconjunctivitis sicca, ocular pemphigoid, radiation-induced sicca, superior limbic keratoconjunctivitis, Stevens-Johnson syndrome, and Bowens-like epithelial dysplasia.52,53,54,55 The initial reports were variably favorable. However, subsequent studies, including one that was multicentered and masked, failed to confirm the initially positive results.56,57
Toxicity
Excessive intake of vitamin A is toxic. Evidence exists that this occurred in prehistoric man.58 Symptoms and findings include papilledema and paresis of the sixth cranial nerves from elevated intracranial pressure (pseudotumor cerebri); yellowish skin with an erythematous eruptions, scaling, and fissures; migratory bone pain; hair loss; enlarged liver and spleen; hypoprothrombinemia; irritability; and weight loss. Work in rats suggested that excess vitamin A circulates in the serum as retinyl esters bound to lipoproteins instead of in the more physiologic manner, as retinol bound to retinol-binding protein.59 The upper limit of normal in humans is <500 IU vitamin A/100 mL plasma. Less than 5% circulates as retinyl esters. Smith and Goodman found that patients with hypervitaminosis A had up to 67% circulating as retinyl esters.60 The concentrations of retinol-binding protein were normal.
Most toxicity occurs in adolescents ingesting vitamin A to prevent acne and in children given vitamin supplements by well-intentioned parents. Cutaneous absorption has been accused of causing hypervitaminosis A in infants.61 Eating chicken liver, polar bear liver, or shark liver can produce intoxication.62 Cases do occur in adults, and one has been reported in a 62-year-old. The daily intake in adults with toxicity has ranged from 25,000 IU for 2 years to 600,000 IU for shorter periods. Toxicity occurred in as little as 2 months when 200,000 to 275,000 IU was ingested daily. Serum vitamin A levels ranging from 320 IU/100 mL (which is usually considered within the normal range) to 6,600 IU/100 mL have been reported.60,62,63,64,65
On discontinuing the drug, serum levels of vitamin A slowly declined. In one patient, the percent of vitamin A in the form of retinyl esters remained more than twice that normally found 9.5 months later.60 The papilledema may resolve relatively quickly (e.g., within 6 days) or may be prolonged (e.g., 4 to 6 months);64 this probably depends on the magnitude of storage in the liver and extrahepatic sites as well as the blood level.
Vitamin A is teratogenic. Increased neural crest defects appeared at a threshold daily dose of 10,000 IU vitamin A if begun before the seventh week of pregnancy. Approximately 1 infant in 57 had a malformation attributable to the supplement.66
Vitamin B1
Vitamin B1 (thiamine) is a water-soluble, heat-labile vitamin. The recommended daily allowance is 0.3 to 0.5 mg in infants and 1.0 to 1.5 mg in adults. The therapeutic dose in cases of dietary deficiency is about 5 to 50 mg/day.
Nutritional Deficiency Amblyopia
Optic nerve damage due to poor nutrition is well established. However, it is not clear which specific deficiency is the cause of the condition (see vitamin B12). There has been variable success in finding an appropriate animal model. Rats fed a thiamine-deficient diet for approximately 6 months developed optic atrophy.67 If the diet lacked all the vitamin B complex components, optic atrophy developed in 70 days.68 However, Leinfelder and Robbie could not produce optic atrophy in rats fed a vitamin B1-deficient diet for 5 months or a vitamin B complex-free diet for 70 days.69 If thiamine deficiency were sufficient to produce nutritional amblyopia, it is surprising that optic nerve dysfunction is so uncommon in the Wernicke-Korsakoff syndrome.
The importance of diet became apparent during the 1940s, when prisoners of war and concentration camp internees developed centrocecal scotomas, reduced visual acuities, and pallor of the temporal optic nerve heads. Involvement was usually bilateral and often irreversible, depending on the degree of optic atrophy. For example, Bloom and coworkers fed prisoners of war with bilateral optic atrophy high-caloric diets supplemented by daily multivitamin capsules and vitamin B1 injections of 50 mg/day.70 Two months of therapy did not improve vision.
The evidence supporting a specific role for thiamine in human nutritional amblyopia is limited. Dreyfus demonstrated a reduced whole blood transketolase activity in two patients with nutritional amblyopia.71 This enzyme is thiamine dependent. Carroll supplemented the diets of patients with nutritional amblyopia with yeast, a rich source of thiamine, or with thiamine itself.72 Yeast, however, contains small amounts, 1 ng/g dry weight, of vitamin B12.73 Because vitamin B12 deficiency may be a cause of amblyopia, the beneficial effects of yeast could not be attributed entirely to thiamine. The group fed thiamine itself consisted of only five patients. After receiving 15 to 43 mg vitamin B1 daily for 3 to 6 weeks, four patients had 20/20 (six eyes) or 20/30 (6/9)( (two eyes) acuities. Vision in the fifth patient improved bilaterally from 20/200 to 20/70 (6/21). When the entire B complex was given to this patient, vision improved to 20/20. Occasionally, childhood seizures have been treated with a high-fat, ketogenic diet. Two children, ages 5 and 7 years, developed centrocecal scotomas with normal-appearing discs while on this diet.74 Thiamine deficiency with a reduced serum transketolase activity was detected. It was attributed to the diarrhea that accompanied the diet and to the failure of the parents to give supplemental vitamins. The serum folic acid and vitamin B12 levels were normal. Thiamine and vitamin B supplements were begun. The visual acuities in one patient improved within 6 weeks from 20/80 (6/24) to 20/40 (6/12). In the other patient, they improved within 12 weeks from 20/120 (6/36) for the right eye and 20/80 for the left eye to 20/15 (6/5) for each eye. The centrocecal scotomas disappeared.
Leigh Disease
Leigh disease (infantile necrotizing encephalomyopathy) is a fatal mitochondrial DNA-inherited disease usually found in children.75 Feeding difficulties and psychomotor retardation progress to ophthalmoplegia, impaired pupillary light reflexes, unconsciousness, and respiratory death.76 Pathologically, the condition resembles Wernicke encephalopathy except for the absence of degeneration in the mammillary bodies. Abnormalities have been reported in the metabolism of thiamine triphosphate. An endogenous inhibitor of the enzyme adenosine triphosphate, thiamine pyrophosphate phosphoryltransferase has been identified in patients with Leigh disease. This inhibitor prevents the synthesis of thiamine triphosphate. Increased cerebral thiamine diphosphate accumulates.77 Several investigators have treated patients with massive doses of thiamine propyldisulfide and thiamine tetrahydrofurfuryl disulfide. Initially, the patients improved, and the inhibitor could not be demonstrated in the urine. Weeks later, the inhibitor reappeared, and the patients died despite continued therapy.78
Wernicke Encephalopathy
Ophthalmoplegia and nystagmus are prominent findings in Wernicke encephalopathy, a vitamin B1deficiency associated with chronic alcoholism, chronic malnutrition, or intravenous hyperalimentation when vitamins are not provided.79 Resolution begins within 24 hours of initiating therapy.
Vitamin B6
Weleber and coworkers, in a short-term study, gave vitamin B6 (pyridoxine) to four patients with gyrate atrophy of the retina.80 Gyrate atrophy is due to an inherited metabolic abnormality causing hyperornithemia. Patients received 18 to 750 mg/day, which is considerably more than the recommended daily dose of 1.6 to 2.5 mg. Three patients responded with a significant reduction in serum ornithine levels, which still remained abnormally high. The electroretinogram and electro-oculogram responses transiently improved. Pyridoxine presumably acted by increasing the activity of the defective enzyme, ornithine aminotransferase, which converts ornithine to glutamic acid or proline. Several forms of the disease may exist, which might explain why some but not all patients respond to pyridoxine treatment.81 However, at best, the reductions in serum ornithine levels are only relative, remaining abnormally high, and the disease progresses.82
A similar situation exists for homocystinuria, a disease producing ectopia lentis and caused by a defect in the enzyme cystathionine synthetase. Cystathionine synthetase requires pyridoxine, in the form of pyridoxal phosphate, as a coenzyme. Some cases of homocystinuria respond to large doses of vitamin B6 and some do not. Presumably, those that respond have a reduced affinity for pyridoxine that can be overcome by daily doses of 250 to 1,500 mg. Responding cases can maintain normal levels of homocystine, and the progression of the disease can be retarded.83,84
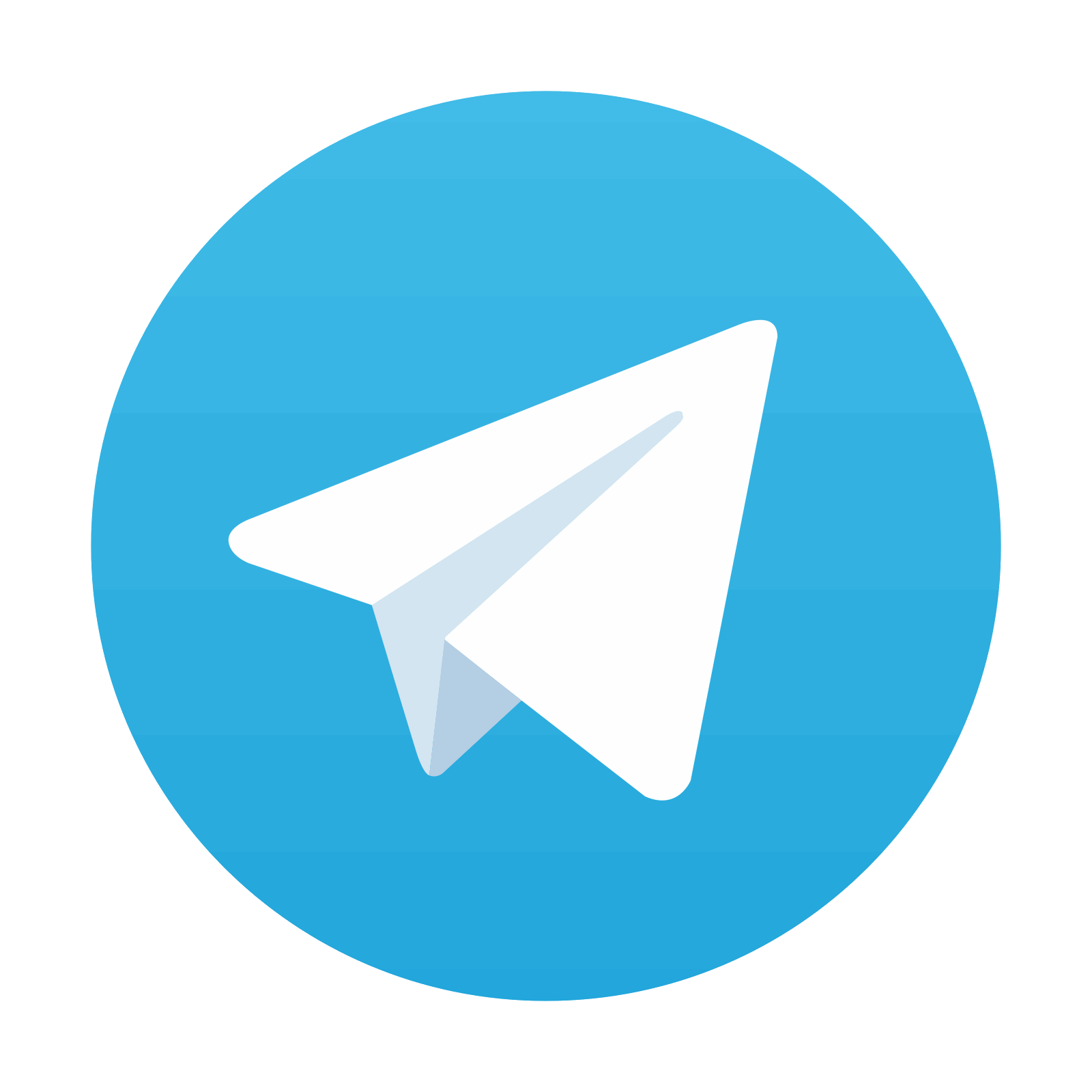
Stay updated, free articles. Join our Telegram channel

Full access? Get Clinical Tree
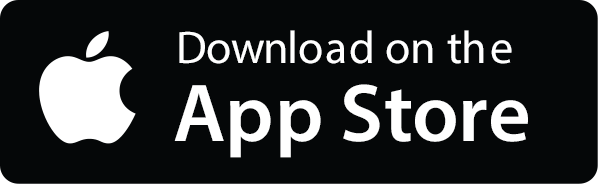
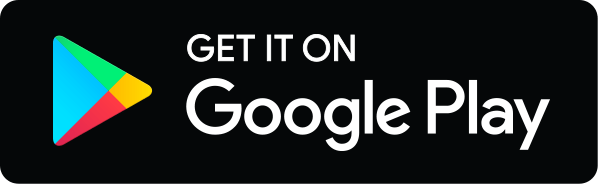