10.1 Basic Concepts of Fluidics
The balance of fluid inflow and outflow is very important in phacoemulsification cataract surgery. One of the goals of the surgery should be to maintain a stable anterior chamber. This can be done by making sure the fluid entering the eye is equal to the amount that exits. This will keep the anterior chamber pressurized. The inflow fluid originates in the irrigation bottle and is a balanced salt solution. The fluid travels from the irrigation bottle through plastic tubing, into the phaco handpiece, through a flexible sleeve around the phaco needle, and finally into the anterior chamber of the eye. To create a pressure gradient the bottle is placed at a height above the patient. Meanwhile, fluid leaves the anterior chamber through the phaco needle and the attached tubing. This fluid egress can be increased by increasing the aspiration flow rate. Another source of fluid loss is through wound leakage. If the balance of inflow and out flow is altered, the anterior chamber can be under- or over-pressurized. If under-pressurized this can lead to shallowing and/or collapse on the anterior chamber. This will cause forward movement of the iris, lens, and posterior capsule. This may lead to inadvertent rupture of the posterior capsule, due to its movement towards the phaco needle. One indicator of anterior chamber pressure imbalance is the bouncing movement of the iris and lens. Over-pressurization (bottle height too high) can cause misdirection of aqueous fluid or deepening of the anterior chamber with zonular stress. Both safety and efficiency of phacoemulsification cataract surgery are directly related to fluidics. Proper settings and use of the machine will improve the safety and efficiency of the surgery. Improper settings can create a dangerous situation.
The term aspiration flow rate (AFR) refers to the amount of fluid exiting the eye through the tubing. This is reported in cubic centimeters per minute (cm3/min). With a peristaltic pump, flow is determined by the speed of the pump. As flow increases the fluid movement in the anterior chamber increases the attraction of particulate matter to the phaco tip, also known as “followability.”
Vacuum refers to the difference in fluid pressure between two points. Negative pressure is measured in millimeters of mercury (mmHg). Vacuum determines how well nuclear material will be held at the phaco tip once it is occluded (holding power).
Compliance refers to the change in the tubing shape and volume in response to negative pressure. When the tip of the phaco needle is occluded, negative pressure will build in the tubing. The higher the tubing’s compliance, the higher the change in its volume. When negative pressure is created, highly compliant tubing collapses, reducing its inner volume. When occlusion breaks, the tubing lumen expands to its original shape and a temporary imbalance causes rapid exit of fluid from the anterior chamber. This is called surge. The higher the compliance, the greater the surge amplitude during occlusion break.
10.2 Basic Principles of Phacoemulsification Power Generation
The phaco handpiece incorporates a transducer for converting high-frequency, alternating current into mechanical vibrations. Piezoelectric transducers are based on the reversals of the piezoelectric phenomenon. Upon compression, certain crystals produce electric current. In reverse, electric current causes the crystal to contract. Applying current to a crystal at high frequency will cause it to oscillate at that frequency. The advantages of piezoelectric crystals include a high grade of efficiency and therefore little inherent heat generation, with no need for extra cooling. The crystals’ low mass allows rapid movement and precise control. Many handpieces use multiple crystals (usually 2 to 4 sets) to maximize responsiveness and provide adequate power to emulsify mature hard nuclei. Disadvantages include the connection points between crystal and electric current, the connections among the multiple layers of crystals that are needed to provide adequate stroke amplitudes, and the structural brittleness of the crystal itself. These properties limit the longevity of the transducers. They are delicate and deteriorate from accidental mechanical injury and the oscillation they produce. Modern phaco systems now have a built-in feedback loop that constantly adjusts or tunes the oscillating frequency to an optimal resonance. This is a function of the central processing unit (CPU) of the machine. It will read the change in resistance of the phaco needle and make minute adjustments in the stroke length or frequency. The greater the frequency of the corrections, the more effective the emulsification will be.
Power is the product of oscillatory frequency (hertz, cycles per second) and the work associated with a given stroke length. Frequency is defined as the speed of the needle movement. It is determined by the manufacturer of the machine. Phacoemulsification needles move at a frequency between 35,000 and 60,000 cycles per second (Hz). This frequency range is the most efficient for nuclear emulsification. Lower frequencies are less efficient and higher frequencies create excess heat.
Stroke length is defined as the length of needle movement. This length is generally 2–6 mil (thousandths of an inch). Most machines operate in the 2–4 mil range. Longer stroke lengths are prone to excess heat generation. The longer the stroke length, the greater the physical impact on the nucleus. Stroke length is determined by foot pedal excursion in position 3 during linear control of phaco. Although the frequency is unchanged, the amplitude of the sine wave is increased in direct proportion to the depression of the foot pedal [1, 2].
The action of phacoemulsification can include several mechanisms, including sonic wave propagation through the fluid medium, the tip’s axial oscillations through its stroke length (the jackhammer effect), and implosion of microcavitation bubbles, producing extreme yet brief instances of heat and pressure [2].
10.3 Fundamentals of Fluidics Study
10.3.1 Bottle Height
Standardization is a basic prerequisite for any comparative pressure study. To work under reproducible conditions and make valid comparisons between techniques that differ in their basic flow characteristics, absolute standardization of the bottle-height-dependent infusion volume is mandatory.
To align the eye with the 0 mark of the IV pole (vent valve position or center of cassette), the operating room (OR) table must be raised or lowered. Hydrostatic pressures for each case and each system are duplicated by adjusting the OR table height so the eye level is aligned relative to IV pole markings. Theoretically, the vent valve should correspond to the 0 mark on the IV pole [3]. With the transducer positioned at eye level, the monitor should be calibrated to zero at atmospheric pressure.
10.3.2 Flow Control
Air is highly compliant, considerably more so than water, balanced salt solution, or aspiration-line tubing. Therefore, it is important to remove all trapped air before laboratory testing, just as it is before surgery. Any temperature experiments must control for flow, especially in low-flow situations, because minute differences in flow can have a significant effect on temperature results. When trying to measure flow with ultrasound on, flow is much less consistent than without ultrasound. It should not be surprising that linear flow characteristics would be altered in the presence of an ultrasound-generating tip. To accurately control low aspiration rates through multiple probes simultaneously, 60 cm3 B-D plastic syringes and syringe infusion/withdrawal pumps can be used (Fig. 10.1) [4, 5].
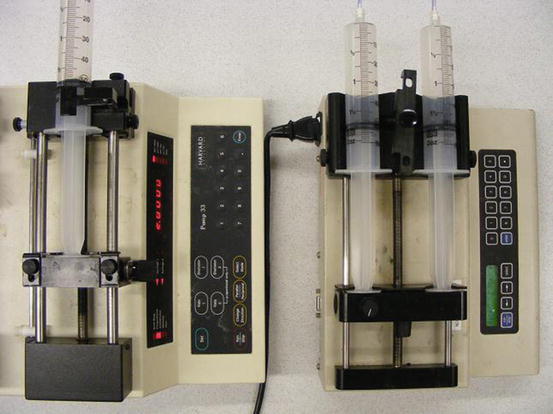
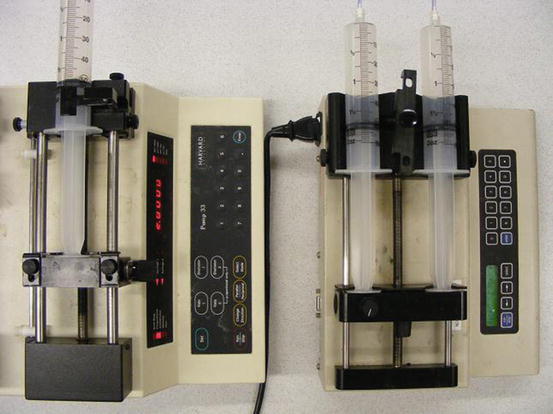
Fig. 10.1
To accurately control aspiration rates through all three probes simultaneously, two 60 cm3 B-D plastic syringes were mounted onto a dual-syringe infusion/withdrawal pump (Cole-Parmer Instrument Co.) and a third 60 cm3 B-D plastic syringe was mounted onto a Model 33 twin syringe pump (Harvard apparatus)
If the study is performed in vivo, repeatability is often difficult. If the study is performed in vitro using human globes, it can also be difficult to reproduce because of difficulties with setup, specimen change through repeated testing, variable incision leakage, and variability between specimens.
10.3.3 Phaco Power Setting
There is no industry standard for phacoemulsification power. Thirty percent power on one machine may be quite different than 30 % power on another machine. Not only that, but different machines have different mechanisms for regulating power delivery to the piezoelectric crystal.
10.3.4 Camera Calibration
Camera calibration should be verified by measuring warm- and cold-water baths using the infrared camera and then comparing the readings with those from a thermocouple at temperatures across the experimental range (Fig. 10.2). Temperature has been measured by using a subminiature microthermister sensor in some studies [6, 7]. The microthermister wire is glued to the phaco sleeve such that the end of the thermocoupled sensor is positioned at a distance of 1.0 mm from the middle of the sleeve irrigation ports. Thermal cameras have an advantage over thermocouple thermometers for thermal imaging studies. Measuring different manufacturers’ probes with different thermocouples may result in calibration- and vibration-induced noise issues that can easily affect the accuracy of the study [4, 5]. For thermal imaging studies, in vitro air environments have advantages over fluid environments. In water, thermal cameras are not able to record probe temperatures directly and it is difficult to achieve strictly controlled low-flow conditions in postmortem eyes.
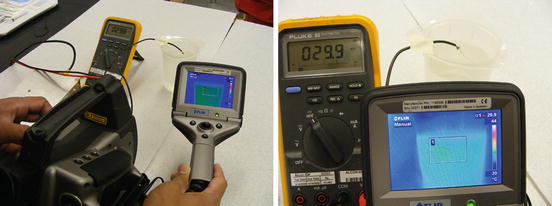
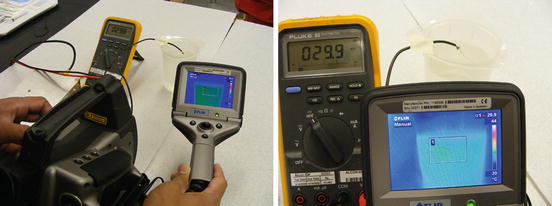
Fig. 10.2
Camera calibration was verified by taking measurements in warm- and cold-water baths by using the infrared camera and comparing the readings to those from a thermocouple at temperatures across the experimental range
10.3.5 Autoclave
Autoclave experiments have showed that residual heat can increase sleeve temperature, especially if minimal time elapses from when the handpiece is removed from the autoclave. Many clinicians have experienced a handpiece that was hot to the touch following a hurried turnover, so this finding is also not surprising. Any additional heat would be additive to ultrasound-related heat increases and could decrease the safety margin relative to the risk for incision burn [4].
10.4 Measuring the Compliance
A major determinant of fluidic system performance is compliance. Compliance is a measure of a system’s ability to expand or contract in response to applied fluidic pressure. Compliance, which is the inverse of stiffness, is defined as Δvolume/Δpressure, where Δ is the change in the parameter. In phacoemulsification systems, compliance is a function of the mechanical properties of the aspiration-line tubing and the cassette that mates the tubing to the pump mechanism. Highly compliant fluidics modules are undesirable because they increase the risk of post occlusion-break surge. At the moment a cataract fragment clears the tip of a needle and occlusion breaks, the potential energy stored in the tubing and cassette releases and fluid is suctioned from the eye to fill the rapidly expanded tubing lumen. Occlusion-break surges have the potential to damage the posterior capsule, cornea, and iris [8].
Dastiridou et al. [9] measured the compliance of the living human eye and found that the pressure/volume relation was nonlinear, with rigidity increasing at higher IOP levels. Nejad et al. [8] compared the compliance and capacity of seven fluidics modules used by six phacoemulsifiers from three manufacturers. They generated compliance curves for positive and negative fluid displacement by injection and withdrawal of balanced salt solution from the aspiration line in small aliquots. For this analysis, they found that the volume of practical interest was 0.05 mL of positive displacement. This corresponds to a positive pressure of approximately 100 mmHg (136 cm of water) in most cassettes, and 0.40 mL of negative displacement, which corresponds to a vacuum of approximately 400 mmHg in most cassettes. For experiments involving negative fluid displacement, aspiration-line pressure was recorded after the injection of 0.05 mL of balanced salt solution and the subsequent quick withdrawal of (9) 0.5 mL aliquots, for a net withdrawal of 0.45 mL. For experiments involving positive fluid displacement, aspiration-line pressure was recorded after the withdrawal of 0.40 mL of balanced salt solution and the subsequent quick injection of (9) 0.5 mL aliquots, for a net injection of 0.45 mL. In this experiment, the Infiniti intrepid system had the lowest compliance of the six units tested, which is optimum from a surge-control perspective.
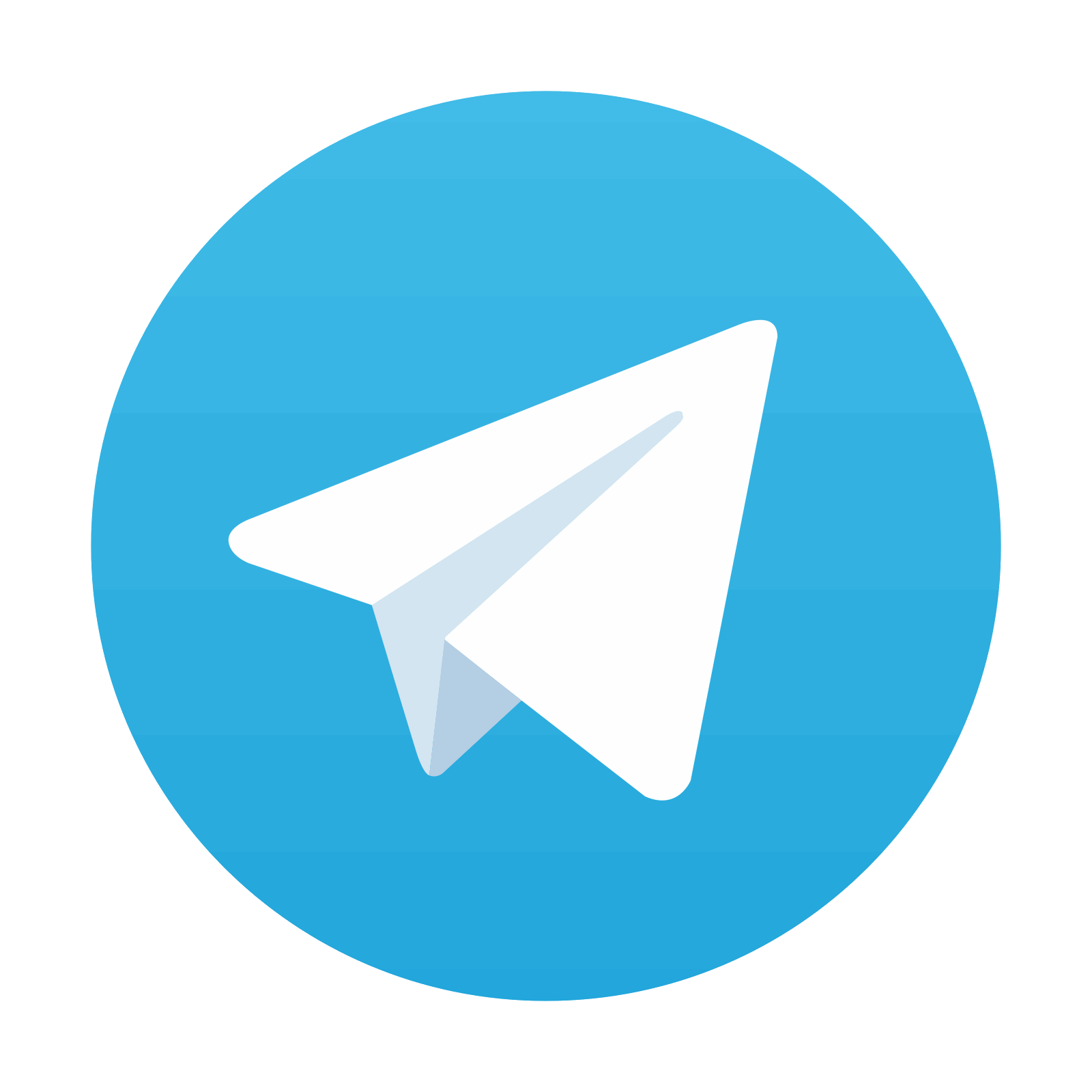
Stay updated, free articles. Join our Telegram channel

Full access? Get Clinical Tree
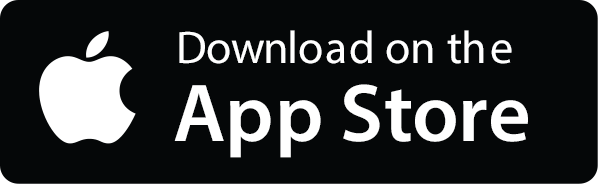
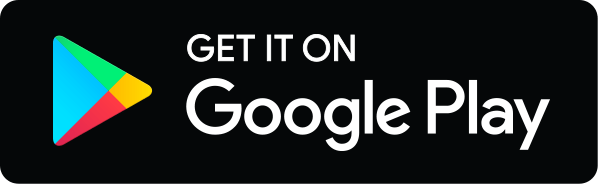