CHAPTER 164 Evaluation of the Patient with Dizziness
Background
Dizziness is the ninth most common complaint leading patients to visit their primary care physicians, rising to third among those 65 to 75 years of age and first among older patients.1,2 Despite its frequency, symptoms of dizziness can be difficult for the patient to describe and for the physician to categorize. Dysequilibrium, unsteadiness, vertigo, and light-headedness all are terms that patients may use to describe their sensations. Evaluation of balance signals from peripheral vestibular nerve afferents, the visual system, and somatosensory and proprioceptive receptors normally occurs below a patient’s level of awareness, making symptoms particularly difficult to describe.
This chapter outlines an approach to the evaluation of patients with dizziness that builds on an understanding of the pathophysiology of dizziness to develop an organized method for history taking and physical examination leading to the identification of specific abnormalities. A description of some of the physiological and functional principles underlying the symptoms and signs of vestibular disorders is presented first. (A comprehensive description of these topics is provided in Chapter 163.) Using an approach based on these considerations, the clinical evaluation, including the history, physical examination, and quantitative tests of vestibular function, is described next. Finally, the adaptive capabilities of the vestibular system and principles of vestibular rehabilitation are reviewed.
Fundamentals of Vestibular Function
Peripheral Anatomy and Physiology
The vestibular receptors are located in the labyrinth and consist bilaterally of three orthogonally oriented semicircular canals and two otolith organs. The semicircular canals sense angular acceleration and are arranged in roughly parallel pairs: the two horizontal (also called “lateral”) canals, the left anterior and right posterior canals, and the right anterior and left posterior canals. The orthogonal geometry of the semicircular canals in each labyrinth permits three-dimensional rotational head movements to be represented as the vector sum of components in each plane. Most vestibular nerve afferents have a spontaneous firing rate between 10 and 100 spikes/second. When the head is still, primary afferents in the right and left vestibular periphery discharge tonically at the same rate. During horizontal head rotation, the horizontal semicircular canal is stimulated in the ipsilateral labyrinth and inhibited in the opposite. During vigorous horizontal head rotations, many afferents and central vestibular neurons on the inhibited side are silenced.3,4
Over the physiologic range of head movements (0.1 to 15 Hz), the intrinsic mechanical properties of the semicircular canals integrate this acceleration signal into a velocity signal. This signal is further modified by individual afferents, providing central vestibular neurons with an array of signals carrying velocity and acceleration information. Activity in the vestibular nerve is the major source of sensory input to the vestibular nuclei, with almost 30,000 afferents projecting from each labyrinth to the brainstem in humans.5 Neurons in the vestibular nuclei typically receive bilateral inputs from the coplanar semicircular canals (direct projections from afferent nerve fibers on the ipsilateral side and commissural projections from central vestibular neurons that cross from the contralateral side), with excitation of peripheral afferents causing increased firing rates of the neurons in the ipsilateral vestibular nucleus.
Eye Movements
Vestibular information is vital to controlling both eye movements and body posture, but eye movements are the more easily studied and the better understood of the two. All eye movements are aimed at ensuring optimal visual acuity (Fig. 164-1). Vestibular and optokinetic eye movements work to hold eye position in space (i.e., gaze) constant by producing compensatory eye movements that keep images stable on the retina during head movements. Saccadic, pursuit, and vergence eye movements change gaze so that images of objects of interest are brought to or kept on the fovea, where visual resolution is highest. Saccades rotate the eye to bring an image onto the fovea, whereas pursuit maintains that image on the fovea as it moves across the visual field. Vergence movements are disjunctive, causing the eyes to move in opposite directions to place the image of an object simultaneously on both foveae during movements of the head or the object (Table 164-1).
Table 164-1 Functional Classes of Human Eye Movements
Class of Eye Movement | Main Function |
---|---|
Visual fixation | Holds the image of a stationary object on the fovea |
Vestibular | Holds images of the seen world steady on the retina during brief head rotations |
Optokinetic | Holds images of the seen world steady on the retina during sustained head rotations |
Smooth pursuit | Holds the image of a moving target on the fovea |
Nystagmus | The repetition of a compensatory slow phase and quick phase resetting movement of the eyes; in quick phases, gaze directed toward the oncoming visual scene |
Saccades | Bring images of objects of interest onto the fovea |
Vergence | Moves the eyes in opposite directions so that images of a single object are placed simultaneously on both foveas |
Visual acuity is degraded if images slip across the retina even as slowly as 2 to 3 degrees/second.6 Vestibulo-ocular reflexes (VORs) are responsible for maintaining binocular fixation and stabilizing binocular foveal images during head movements.7 The VORs are divided into two types: angular and translational. Angular reflexes are initiated by activation of the semicircular canals. The canals are aligned with the pulling directions of the three pairs of extraocular muscles,8 so that activation of a single semicircular canal (as in benign positional vertigo or canal dehiscence) leads to motion of the eyes about an axis that aligns with the axis of the semicircular canal (Fig. 164-2). The latency of the angular reflex is approximately 7 msec in humans.9
Translational VORs are driven by otolith afferents and can be grouped into two categories according to the eye movement responses: tilt responses, which compensate for lateral head tilt with respect to gravity, and translational responses, which produce eye movements compensatory for linear movements of the head (Fig. 164-3). Lateral head tilt produces ocular counter-rolling, a torsional movement of the eye about the line of sight. The torsional deviation of the eyes in response to 60- to 75-degree static head tilts is 6 to 8 degrees.10 The small amplitude of eye movement produced by relatively large tilts of the head appears to indicate that ocular counterrolling is a vestigial response with little compensatory function, but during lower-amplitude sinusoidal head rolls, the torsional gain is significantly greater. Compensatory eye movements in these situations seem to result largely from canal-related reflexes, although the otolith organs also are activated.11 Translational VORs produce eye movements that maintain the point of binocular fixation for each retina during linear head movements.
Otolith afferents cannot distinguish between tilt and translation. Information from the semicircular canals, comparison of the phasic and tonic components of modulation in otolith afferent activation, frequency-selective organization of otolith-mediated responses, or other sensory signals such as vision or proprioception must be involved in determining appropriate reflex responses. For example, tilting of the head involves both canal and otolith activation, and the combined activation of these receptors provides cues to the brain that the modulation in otolith activity is due to a tilt and not to a linear head movement. Frequency-selective filtering of otolith afferent signals provides another complementary mechanism. Head movements that change slowly with respect to gravity (frequency content less than 0.25 Hz) may be interpreted as tilt stimuli, whereas those with higher frequency content lead to responses that are directed to maintaining stable gaze during linear head movements.12,13
Head Movements
Compared with the relatively simple task of the six muscles moving each eye, the 20 muscles moving the neck must contend with more complicated planar relationships, the substantial inertia of the head, and the effect of stretch reflexes and muscular co-contraction. The situation is even more complicated because the trunk and lower extremities are involved in maintaining posture and upright stance. Two reflexes are important for stabilizing the head. The vestibulocollic reflex (VCR) governs the activation of neck muscles in response to vestibular input,14 and the cervicocollic reflex (CCR) governs the activation of neck muscles in response to stretch receptors.15 Studies of head stability during locomotion in humans have shown that the peak velocity of these rotational head perturbations generally does not exceed 150 degrees/second, but their predominant frequency can be as high as 5 Hz, with a frequency for some components of head movements up to 15 Hz.16–19 Studies of head movements in patients who have lost vestibular function indicate that head stability can be achieved by way of the mechanical properties of head and neck muscles, rather than neural reflexive factors.20,21 The CCR, consisting of compensatory eye movements during head-on-body rotation caused by neck proprioception, contributes little to gaze stability in healthy people, although it may become more important if labyrinthine function is lost.22,23
Combinations of eye and head movements are used to acquire and track a target of interest, as well as to stabilize gaze in response to perturbations of the body. Voluntary eye-head movements can be thought of as either saccadic or pursuit in nature. During small eye-head saccades (less than 30 degrees), the shift of gaze may be achieved by superposition of an internal saccadic command and the VOR.21 For larger eye-head saccades (more than approximately 40 degrees), the VOR appears to be “turned off” or “disconnected.” Nevertheless, the vestibular signal caused by the head movement is still available so that an accurate gaze change can be achieved.24 During smooth eye-head tracking, more than one mechanism may operate to override the VOR so that gaze follows the moving target smoothly. Evidence suggests that an internal smooth pursuit signal partly cancels the VOR, and that an additional mechanism may be reduction in the activity or partial suppression of the VOR during such eye-head tracking.25–28 Disorders causing impairment of smooth pursuit usually, but not always, also cause deficits of smooth, combined, eye-head tracking.29 Patients who have lost vestibular function, on the other hand, often show combined eye-head tracking that is superior to ocular pursuit.26
Contributions of the Brainstem and Cerebellum
The cupula of the semicircular canal returns to neutral position with a time constant of approximately 6 seconds, but the associated decay in eye movements is closer to 15 seconds in normal humans because of the effect of a process termed velocity storage. The neural center responsible for this process is located in the brainstem. This mechanism improves the capability of the system to respond to low-frequency head motion30 and to orient vestibulo-ocular responses to gravity.31,32 Velocity storage is (transiently) lost in cases of acute unilateral vestibular loss and remains diminished in the long term.
The neural integrator is another brainstem mechanism vital for proper eye movements.33 It produces a steady command to the extraocular muscles to hold the eyes eccentrically, instead of allowing them to drift back to neutral gaze position because of the elastic restoring forces of the orbital tissues. Proper function of the neural integrator also is necessary for the VOR to have the appropriate phase (timing) relationship to head rotation. The neural integrator is not able to hold gaze appropriately in cases of acute unilateral deafferentation.
The cerebellum plays an important role in immediate and long-term adaptive ocular motor control.34 Lesions of the flocculus impair smooth pursuit and VOR cancellation, which normally allows the eyes to move with head rotations to follow a target.35 Floccular lesions may cause horizontal gaze-evoked nystagmus, downbeat nystagmus, rebound nystagmus, increased or decreased amplitude (gain) of the VOR, and postsaccadic drift, or glissades.
The vestibulocerebellum also is important for ensuring that the eyes rotate in a plane parallel to head rotation so that images can be stabilized appropriately on the retina.36 The dorsal vermis and underlying fastigial nuclei are important in the control of saccadic amplitude and smooth pursuit; lesions here produce saccadic inaccuracy (dysmetria) and impair the ability to make long-term adaptive adjustments in saccade accuracy.37 Other “cerebellar” eye signs, which cannot be localized precisely, include square-wave jerks, defective convergence, divergence nystagmus, and alternating hyperdeviation (skew) on lateral gaze.38
Approach to the Patient with Dizziness
History
From the history, the clinician should have a general idea of whether the symptoms are attributable to a vestibular disorder and, if so, whether this disorder is central or peripheral in origin. An outline of diagnostic possibilities based on symptoms and signs is shown in Figure 164-4.
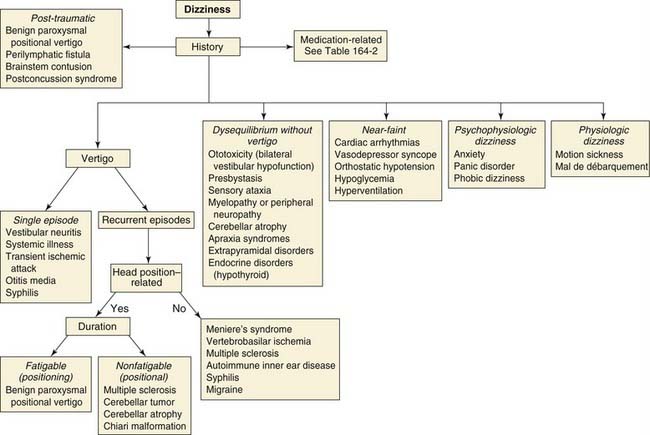
Figure 164-4. Algorithm for the differential diagnosis for dizziness, based on information from the patient’s history.
(Modified from Baloh RW, Fife TD, Furman JM, Zee DS. The approach to the patient with dizziness. In: Mancall EL, ed. Continuum: Lifelong Learning in Neurology. Vol 2. Cleveland: Advanstar Communication; 1996:25-36.)
Table 164-2 Type and Mechanism of Dizziness Associated with Commonly Used Drugs
Drug(s) | Type(s) of Dizziness | Mechanism(s) |
---|---|---|
Aminoglycosides, cisplatin | Vertigo, dysequilibrium | Damage to vestibular hair cells |
Tranquilizers | Intoxication | Central nervous system depression |
Antiepileptics | Dysequilibrium | Cerebellar toxicity |
Antihypertensives, diuretics | Near-syncope | Postural hypotension, reduced cerebral blood flow |
Amiodarone | Dysequilibrium and oscillopsia | Unknown |
Alcohol | Intoxication, dysequilibrium, positional vertigo | Central nervous system depression, cerebellar toxicity, change in cupular and endolymphatic specific gravity |
Methotrexate | Dysequilibrium | Brainstem and cerebellar toxicity |
Anticoagulants | Vertigo | Hemorrhage into inner ear or brain |
From Baloh RW, Fife TD, Furman JM, Zee DS. The approach to the patient with dizziness. In: Mancall EI, ed. Continuum: Lifelong Learning in Neurology. Vol 2. Cleveland: Advanstar Communications; 1996:25-36.
Physical Exam
Bedside Exam
Nystagmus
Eye movements indicative of a vestibular disorder commonly take the form of nystagmus. Several different types of nystagmus are recognized (Table 164-3). The most common is jerk nystagmus, which consists of so-called slow phases, which represent vestibular signals for eye movements, and fast phases, which represent resetting actions bringing the eyes back toward the center of the orbit but are not as relevant for diagnostic purposes. The direction of nystagmus often is named for the direction of the fast phases, because the fast phases are more obvious on clinical examination. The trajectory of the slow phases should be scrutinized for the following characteristics of individual slow phases: (1) an increase in velocity, as is commonly found with congenital nystagmus; (2) a decrease in velocity, as is seen in patients with cerebellar lesions; and (3) a constant velocity, as is typical for vestibular disturbances.
Table 164-3 Clinical Observations and Causes of Nystagmus
Observation | Cause(s) |
---|---|
Sinusoidal oscillation without fast phases: “pendular nystagmus” | Multiple sclerosis, toluene intoxication, or brainstem infarction with inferior olivary hypertrophy (the syndrome of palatomyoclonus)a,b Acquired pendular nystagmus frequently disconjugate; may even be horizontal in one eye and vertical in the other May be responsive to memantinec |
Purely torsional nystagmus | Intrinsic brainstem involvement within the vestibular nuclei; suggestive of syringomyeliad |
Downbeat nystagmus | Arnold-Chiari deformity or degenerative lesions of the cerebellume Treated with 4-aminopyridine |
Upbeat nystagmus | Lesions at the pontomedullary or pontomesencephalic junction or within the fourth ventriclef |
Horizontal jerk nystagmus that changes direction approximately every 2 minutes: “periodic alternating nystagmus” | Lesions in the nodulus of the cerebellumg,h Treated with baclofen |
Nystagmus on attempted eccentric gaze and with slow phases that show a declining exponential time course | Side effect of certain medications, especially anticonvulsants, hypnotics, and tranquilizers, and in patients with disease of the vestibulocerebellum or its brainstem connections in the medial vestibular nucleus and nucleus prepositus hypoglossii |
Accelerating slow phases accentuated by attempted fixation, decreased by convergence or active eyelid closure, associated with a head turn, and sometimes accompanied by “reversed” smooth pursuit: “infantile” or “congenital” nystagmus | May be related to central or peripheral deficitsj May be associated with albinism, retinal diseases, and early visual deprivation May be responsive to neurontin or memantinej,k |
Gaze-evoked nystagmus that may dampen and actually change direction (“centripetal nystagmus”) and often is followed by rebound nystagmus (slow phases are directed toward the previous position of eccentric gaze) when the eyes return to the primary position | Peripheral vestibular lesions, drug intoxication, and cerebellar lesionsl Rebound nystagmus associated with many cerebellar lesions, including olivocerebellar atrophym |
Slow phases with exponentially increasing waveforms | Congenital nystagmus and acquired lesions of the cerebellum |
Nystagmus of greater intensity or present only in the abducting eye: “dissociated nystagmus” | Commonly occurs in patients with internuclear ophthalmoplegia (INO) Mechanism unknown for abducting nystagmus in INOn |
One eye goes up and the other goes down: “seesaw nystagmus” | Midbrain lesions May be related to an imbalance of activity in structures that receive projections from the labyrinthine otolith organso |
Slow phases directed centrally and with retraction of the eye into the orbit: “convergence-retraction nystagmus” | Midbrain lesions May be a failure of saccades or of vergencep Usually coexists with upgaze paralysis (Parinaud’s syndrome) |
Skew deviation and the ocular tilt reaction (one half-cycle of seesaw nystagmus) | Occurs with acute peripheral vestibular (otolith organ) lesions and with lesions in the medulla, the pons, and the midbrain Often associated with head tiltq |
Nystagmus occurring in a plane other than that of vestibular stimulation: “perverted nystagmus” | Central vestibular disorders; multiple sclerosisr |
a Data from Hain TC, Zee DS, Maria BL. Tilt suppression of vestibulo-ocular reflex in patients with cerebellar lesions. Acta Otolaryngol. 1988;105:13-20.
b Data from Nakada T, Kwee IL. Oculopalatal myoclonus. Brain. 1986;109(Pt 3):431-441.
c Data from Stahl JS, Rottach KG, Averbuch-Heller L, et al. A pilot study of gabapentin as treatment for acquired nystagmus. Neuroophthalmology. 1996;16:107-113.
d Data from Noseworthy JH, Ebers GC, Leigh RJ, et al. Torsional nystagmus: quantitative features and possible pathogenesis. Neurology. 1988;38:992-994.
e Data from Baloh RW, Yee RD. Spontaneous vertical nystagmus. Rev Neurol (Paris). 1989;145:527-532.
f Data from Ranalli PJ, Sharpe JA. Upbeat nystagmus and the ventral tegmental pathway of the upward vestibuloocular reflex. Neurology. 1988;38:1329-1330.
g Data from Leigh RJ, Robinson DA, Zee DS. A hypothetical explanation for periodic alternating nystagmus: instability in the optokinetic-vestibular system. Ann N Y Acad Sci. 1988;374:619-635.
h Data from Waespe W, Cohen B, Raphan T. Dynamic modification of the vestibulo-ocular reflex by the nodulus and uvula. Science. 1985;228:199-202.
i Data from Lewis RF, Zee DS. Ocular motor disorders associated with cerebellar lesions: pathophysiology and topical localization. Rev Neurol (Paris). 1993;149:665-677.
j Data from Jacobs JB, Dell’Osso LF. Congenital nystagmus: hypotheses for its genesis and complex waveforms within a behavioral ocular motor system model. J Vis. 2004;4:604-625.
k Data from McLean R, Proudlock F, Thomas S, Degg C, Gottlob I. Congenital nystagmus: randomized, controlled, double-masked trial of memantine/gabapentin. Ann Neurol. 2007;61:130-138.
l Data from Leech J, Gresty M, Hess K, Rudge P. Gaze failure, drifting eye movements, and centripetal nystagmus in cerebellar disease. Br J Ophthalmol. 1977;61:774-781.
m Data from Bondar RL, Sharpe JA, Lewis AJ. Rebound nystagmus in olivocerebellar atrophy: a clinicopathological correlation. Ann Neurol. 1984;15:474-477.
n Data from Zee DS, Hain TC, Carl JR. Abduction nystagmus in internuclear ophthalmoplegia. Ann Neurol. 1987;21:383-388.
o Data from Nakada T, Kwee IL. Seesaw nystagmus. Role of visuovestibular interaction in its pathogenesis. J Clin Neuroophthalmol. 1988;8:171-177.
p Data from Rambold H, Kompf D, Helmchen C. Convergence retraction nystagmus: a disorder of vergence? Ann Neurol. 2001;50:677-681.
q Data from Parulekar MV, Dai S, Buncic JR, Wong AM. Head position-dependent changes in ocular torsion and vertical misalignment in skew deviation. Arch Ophthalmol. 2008;126:899-905.
r Data from Minagar A, Sheremata WA, Tusa RJ. Perverted head-shaking nystagmus: a possible mechanism. Neurology. 2001;57:887-889.
The characteristics of nystagmus when the eyes are directed away from center often adds additional diagnostic information. The patient is asked to maintain eccentric horizontal and then vertical positions of gaze (approximately 30 degrees from center orientation). Minimal drift normally should be encountered for periods up to 15 seconds. Nystagmus arising from peripheral lesions and some central lesions is more intense (slow-phase velocity is higher) when the eyes are turned in the direction of the quick phase.39 This effect, known as Alexander’s law (Fig. 164-5), is due to the combination of gaze-evoked nystagmus (caused by the initial loss of the neural integrator after a peripheral lesion) and with the vestibular nystagmus caused by the static asymmetry of the lesion itself. The two factors add on looking away from the lesion and cancel each other on looking toward it. Bruns’ nystagmus, found in patients with cerebellopontine angle tumors, is a combination of gaze-evoked nystagmus with low-frequency, large-amplitude, fast phases on looking toward the side of the lesion and jerk nystagmus with high-frequency, small-amplitude, fast phases on looking the other way.40 The gaze-holding component of the nystagmus may represent an adaptation to the jerk nystagmus caused by vestibular imbalance.41 Horizontal gaze–evoked nystagmus is a hallmark of lesions in the medial vestibular nucleus and nucleus prepositus hypoglossi complex. Low-amplitude gaze-evoked nystagmus often is a side effect of many types of medications, including hypnotics, sedatives, and anxiolytics. Many healthy persons may exhibit some physiologic, horizontal, end-gaze nystagmus. It usually disappears once the target is brought back into view of both eyes (i.e., at approximately 30 degrees of eccentricity).
Skew Deviation and Ocular Tilt Reaction
Skew deviation is a vertical misalignment of the eyes that cannot be explained on the basis of an ocular muscle palsy (Fig. 164-6). It is the hallmark of an imbalance in tonic levels of activity along peripheral or central pathways mediating otolith-ocular reflexes.42 Patients with skew deviation often complain of vertical diplopia and sometimes torsional diplopia (one image tilted with respect to the other). The alternate cover test is used to detect skew deviation: The examiner covers one eye of the patient with a card and then moves the cover to the patient’s other eye while looking for a vertical corrective movement as an index of a vertical misalignment. A skew deviation also can be detected by covering one eye with a red glass (Maddox rod) to dissociate images seen in the two eyes and inquiring whether the patient sees one image above the other, indicating vertical misalignment. The effect of position of the eye in the orbit and of left and right head tilt on the skew also should be examined. Skew deviations tend to be relatively comitant (i.e., the degree of misalignment changes little with different direction of gaze), whereas a fourth cranial nerve palsy causes a vertical misalignment that is greatest with the affected eye down and medial. The adducting eye is higher and the misalignment is greater with the head tilted toward the side of the higher eye. Skew deviations in otolith-ocular imbalance do not show such a clear relation to changes in eye and head position, although they may be decreased or disappear when the affected person is supine.43 The lower eye is on the side of the lesion with peripheral or vestibular nucleus lesions. Otolith-ocular pathways cross at the level of the vestibular nucleus, so lesions above the decussation result in the higher eye being on the side of the lesion (such as with lesions of the medial longitudinal fasciculus causing internuclear ophthalmoplegia). The head usually is tilted toward the side of the lower eye. Skew deviations also can occur with cerebellar lesions.
Saccades
Rapid changes in gaze from one target to another are achieved by saccades. The neural signal for a saccade includes a pulse of activity, which brings the eye to its new position, and a step, which is a constant neural signal holding the eye at its new position in the orbit (Fig. 164-7). Saccades are examined by asking the patient to alternately fixate (with the head still) on the examiner’s nose and then on the finger, held at different locations at approximately 15 degrees away from primary position. Measures of saccades such as accuracy, velocity, and stability represent the effects of the neural pulse, the step, and the match between pulse and step.44 An error in the pulse amplitude creates overshoot or undershoot dysmetria. This is characteristic of disorders of the dorsal vermis or the fastigial nuclei of the cerebellum, although it appears with lesions in other parts of the nervous system. Wallenberg’s syndrome produces a specific pattern of saccadic dysmetria: Saccades overshoot to the side of the lesion, undershoot away from the side of the lesion, and, with attempted purely vertical saccades, show an inappropriate horizontal component toward the side of the lesion. Lesions of the superior cerebellar peduncle produce just the opposite: Saccades overshoot when directed away from the side of the lesion.45
Normally, saccades follow a relatively invariant relationship between peak velocity and amplitude termed the main sequence.46 A decrease in the height of the saccadic pulse causes slow saccades. Slow horizontal saccades usually imply disease of the pons such as olivopontocerebellar atrophy (spinal cerebellar atrophy type 2 [SCA2]). Slow vertical saccades usually imply disease affecting the midbrain such as progressive supranuclear palsy, Huntington’s disease, or Niemann-Pick disease. A mismatch between the size of the pulse and step produces brief (several hundred milliseconds) postsaccadic drift, or glissades. Postsaccadic drift occurs with disease of the cerebellar flocculus. The combination of slow, hypometric saccades and postsaccadic drift also occurs in patients with internuclear ophthalmoplegia, oculomotor nerve palsies, myasthenia gravis, and ocular myopathies. Hypermetric saccades with macrosaccadic oscillations (large saccades about the position of the target) typically are found in patients with lesions in the midline deep cerebellar nuclei.47 Saccadic oscillations without an intersaccadic interval (back-to-back, to-and-fro saccades) are called ocular flutter when they are limited to the horizontal plane and opsoclonus when they are multidirectional (horizontal, vertical, torsional). Either type of oscillation may occur in patients with various types of encephalitis or postinfectious immune-mediated processes, as a paraneoplastic remote effect of neuroblastoma or other tumors, or in association with toxins. Ocular flutter also may be voluntarily elicited by some normal people.48
The normal saccadic latency is approximately 200 msec, but this latency is increased by disorders of saccadic initiation. Impaired saccadic initiation has been reported in patients with a variety of conditions, including frontal lobe lesions, congenital or acquired oculomotor apraxia, Huntington’s disease, progressive supranuclear palsy, and Alzheimer’s disease. Patients with bilateral frontal lobe lesions or Parkinson’s disease also have difficulty in rapidly alternating gaze between two stationary targets. Patients with Huntington’s disease show a characteristic defect in initiating more voluntary, as opposed to more reflexive, saccades. They have particular difficulty in predictive tracking and are unable to suppress inappropriate saccades to the visual target.49
Inappropriate saccades disrupt steady fixation.50 They include square-wave jerks, which are small-amplitude (up to 5 degrees) saccades that take the eyes off target and are followed within 200 msec by a corrective saccade. Square-wave jerks may occur in healthy elderly persons or in patients with cerebral hemisphere lesions but are especially prominent in patients with progressive supranuclear palsy and cerebellar disease. Square-wave jerks may be an exaggeration of the microsaccades that occur in healthy people during fixation and can be detected most easily by ophthalmoscopy when the patient is instructed to fixate on a target seen with the other eye. Macro square-wave jerks (10 to 40 degrees in amplitude, with a short intersaccadic interval) have been observed in patients with multiple sclerosis and olivopontocerebellar atrophy.
