Eustachian Tube Normal Physiology
The Eustachian tube is part of a structure comprising the nose, palate, nasopharynx, and middle ear spaces.1 This includes the tympanic cavity, which contains the bony Eustachian tube and the mastoid cells system (▶ Fig. 2.1). The tympanic cavity and mastoid cells are connected via the Eustachian tube and permit gas and pressure exchanges. The Eustachian tube is a complex organ consisting of a dynamic canal with its mucosa, cartilage, surrounding soft tissue, peritubal muscles, superior bony support, and the sphenoid sulcus.
Fig. 2.1 Drawing of the cartilaginous and bony portions of the Eustachian tube and middle ear spaces.
Clinical experience as well as numerous patient studies and animal models show that the Eustachian tube plays a significant role in various middle ear pathologies.2,3,4 The understanding of its mechanical properties has improved significantly over the last years; however, there are still uncertainties due to its complex anatomy, various functions, and the impact of intrinsic and external factors (▶ Fig. 2.2).5,6 Intermittent transitory tubal dilation is probably the major mechanism for equilibration of middle ear cleft pressure with the ambient atmospheric pressure.7 Barometric and chemical receptors within the middle ear cleft are assumed to provide autonomic nervous system feedback that impact the frequency of involuntary tubal openings.8,9
Fig. 2.2 Frontal view of the most important anatomic structures of the Eustachian tube.
tvp m, tensor velipalatini muscle, medial lamina
tvp l, tensor veli palatini muscle, lateral lamina
lvp, levator veli palatini muscle
pm, medial pterygoid muscle
tc l, tubal cartilage, lateral lamina
tc m, tubal cartilage, medial lamina
of l, lateral Ostmann’s fat pad
of m, medial Ostmann’s fat pad
RSC, Rüdinger’s safety canal
tsl m, medial tubal suspensory ligament
tsl l, lateral tubal suspensory ligament
Recently, McDonald and coworkers showed that the Eustachian tube may have a sequential peristaltic-like mechanism.10 The proximal one-third of the tubal length is a bony funnel-shaped extension of the middle ear, which becomes narrowest at the isthmus region. The distal two-thirds of the tubal length is the pharyngeal portion, which is composed of cartilage. The cartilaginous portion is attached to peritubal muscles capable of a broad range of dynamic movements. Tubal dilation starts with the action of the levator veli palatine muscle to medially rotate the cartilaginous portion, primarily its mobile distal half of the medial cartilaginous lamina.9 Tensor veli palatine muscle contraction follows, causing the resting convexity of the anterolateral wall to become concave as the concluding step to transiently opening the lumen.10,11,12
Currently, the surgical interventions available for chronic obstructive Eustachian tube dysfunction (ETD) have been expanded to include balloon Eustachian tuboplasty (BET), laser tuboplasty, and microdebrider tuboplasty, but long-term results still have to be established.11,12,13,14,15,16,17
2.2 Eustachian Tube Dysfunction
Eustachian tube dysfunction (ETD) can lead to clinical symptoms, such as aural fullness, impaired pressure equilibration, altered middle ear aeration, hearing loss, and autophony. Because the most common cause of obstructive dysfunction is mucosal inflammation within the cartilaginous Eustachian tube, patients should be questioned about inflammatory processes, such as allergic rhinitis, chronic rhinosinusitis, laryngopharyngeal reflux (LPR), and smoke exposure.18,19 Pediatric ETD may be caused by adenoid tissue and mucosa swelling due to acute or chronic upper respiratory tract infections. Cleft palate, granulomatous disease, cystic fibrosis, Sampter’s triad, or Kartagener’s syndrome are facilitating factors. Eustachian tube dysfunction may be a contributing factor to vertigo.1,20,21 It is important to distinguish ETD from other causes of aural fullness, such as a patulous Eustachian tube, temporomandibular joint (TMJ) disorders, superior semicircular canal dehiscence syndrome, Meniere’s disease, and increased intracranial pressure.
Eustachian tube dysfunction is estimated to be present in about 1% of the general population.22 Nearly 40% of all children up to the age of 10 years develop a temporary ETD.23,24,25,26 Studies accessing pediatric and adult patient cohorts demonstrate that ETD is detectable in up to 70% of patients undergoing middle ear surgery.25 Eustachian tube function is believed to be strongly related to the outcome of middle ear surgery.25,26 Patients with a patulous Eustachian tube usually present with positional autophony, with autophony of both voice and breathing sounds, aural fullness, and, less commonly, otalgia.27 The precise distinction between both forms of ETD (obstructive and patulous) is pivotal for an appropriate medical or surgical treatment. Patients with patulous Eustachian tubes may benefit from augmentation or reconstructive procedures.27,28
2.3 Eustachian Tube Assessment
A large number of methods have been employed to assess Eustachian tube function. More than 40 methods can be found in the literature.29 None of these are proven to give detailed insights into all aspects of Eustachian tube physiology and pathology.30 Clinical tests, such as otoscopy, nasal endosocopy, the Politzer test, the Valsalva maneuver, and the Toynbee maneuver, may give an initial assessment. Manometric tests, such as tympanometry, reflex decay tympanometry, nine-step inflation-deflation test, modified inflation-deflation test, forced response test, and tubomanometry (TMM), are applicable diagnostic tests.3,31,32
TMM was described by Estève in 200133,34 and is a tool to measure the opening of the Eustachian tube and the transportation of gas into the middle ear by registering pressure changes (▶ Fig. 2.3). These are recorded by a pressure sensor in the occluded outer ear after applying the stimulus of a controlled gas bolus into the nasopharynx during swallowing. If tube opening is registered, the time of opening in relation to pressure applied can be measured (opening latency index or R). An R value of <1 indicates early opening of the Eustachian tube, which is considered optimal.32 The pressure chamber is an ideal device for simulating all possible situations regarding Eustachian tube function, regardless of an intact or defective ear drum.
Fig. 2.3 Eustachian tube measurement by tubomanometry. The upper line represents the pressure in the nasopharynx, the lower line the pressure in the external ear canal.
Acoustic testing employing sonotubometry applies sound via a probe in the nose. At the same time, the changes of sound intensity during tube opening are recorded in the external ear canal. There are numerous other approaches to assess Eustachian tube function, such as phototubometry, scintigraphy, ultrasound, application of dye and taste-bearing substances, electromyography, flowmetry, and (opto)tensometry.29,30 However, none of these methods has shown clinical significance.30 Imaging using computed tomography (CT), cone beam tomography, and magnetic resonance imaging (MRI) have long been established to assess anatomic and functional deficiencies as well as to rule out pathology in the nasopharynx or superior canal dehiscence syndrome.4,19,35,36
2.4 Middle Ear and Mastoid Gas Exchange
The middle ear space and mastoid exchange gases with four different components: (1) the inner ear via the round window membrane; (2) the ambient atmospheric pressure via the tympanic membrane; (3) the resident blood via the middle ear mucosa; and (4) the nasopharynx via the Eustachian tube.37,38 Gas exchange across pathways 1 to 3 is a passive, partial-pressure, gradient-driven, diffusion-based exchange process (▶ Fig. 2.4). The gas exchange via the Eustachian tube (pathway 4) is an active, total-pressure, gradient-driven bolus exchange of mixed gases in the air phase.
Fig. 2.4 Partial pressures (mm Hg) of the physiological gases for the middle ear spaces and surrounding structures.
Data are taken from Hergils L, Magnuson B. Regulation of negative middle ear pressure without tubal opening. Arch Otolaryngol Head Neck Surg 1988;114:1442–1444.
The components that are present in the middle ear cleft are identical to those found in the blood and in the atmosphere: oxygen (O2), carbon dioxide (CO2), nitrogen (N2), argon (Ar), and water (H2O). Their relative quantities are expressed by their partial pressure P(x). Nitrogen is inert at the level of the middle ear cleft. The P(O2) in the middle ear cleft is slightly lower and the P(CO2) is slightly higher compared with venous blood. This hints at a modest consumption of O2 and production of CO2 within the middle ear cleft. Oxygen and nitrogen are removed by a passive, partial-pressure, gradient-driven, diffusive exchange via the mucosa into the blood compartment. Carbon dioxide and water vapor are diffused passively from the blood compartment, via the mucosa, into the middle ear cleft. The steady exchange of gas through the mucosa depends on the functional properties of the cells of the mucosal lining, the constant specific diffusion rate of the gas, and the underlying vascular system. The main purpose of the mucosa is to facilitate gas exchange between the middle ear cleft and the blood compartment.
Sound transmission via the ossicular chain requires a stable air compartment within the middle ear spaces. This means that the intra-middle ear cleft pressure must be equivalent to the atmospheric pressure (760 mm Hg). In ambient air, this equivalent value is obtained by calculating the sum of the partial pressures of the four main constitutive components in the air: O2 (158 mm Hg), CO2 (0.3 mm Hg), N2 (596 mm Hg), and water vapor (5.7 mm Hg).38 However, in the middle ear cleft, the composition of gas varies for two main reasons.37 First, the middle ear cleft is a closed cavity that is connected with the nasal cavities by the Eustachian tube, and the gas in the nasopharynx consists of exhaled gas that contains less O2 and more CO2 compared with ambient air. The composition of gas in the middle ear cleft also varies because gas diffusion occurs between the middle ear spaces and the arterial and venous blood, via the mucosa. Arterial blood oxygen tension is approximately 93 mm Hg, whereas venous CO2 tension is 44 mm Hg. The composition of gas tension in the middle ear cleft differs from that in the ambient air. The gradient from middle ear spaces to the capillaries is 57 mm Hg for O2, and the gradient from the capillaries to the middle ear spaces is 39 mm Hg for CO2.
Despite the differences in gas composition, the pressure in the middle ear spaces approximates the atmospheric pressure to enable optimal sound transmission. This is due to the sum of the partial pressures of O2 and CO2 being lower in the middle ear cleft (90 mm Hg) than in ambient air (150 mm Hg); N2 exerts the higher partial pressure in the middle ear spaces (623 mm) because of its very slow diffusion to the capillaries. The composition of the exhaled gas that enters the middle ear spaces via the Eustachian tube contains less O2 and more CO2 compared with the ambient air. This reduces the passive diffusion of these gases through the mucosa. The blood flow through the middle ear mucosa is probably small. This limits the importance of gas diffusion and enables the P(O2) and P(CO2
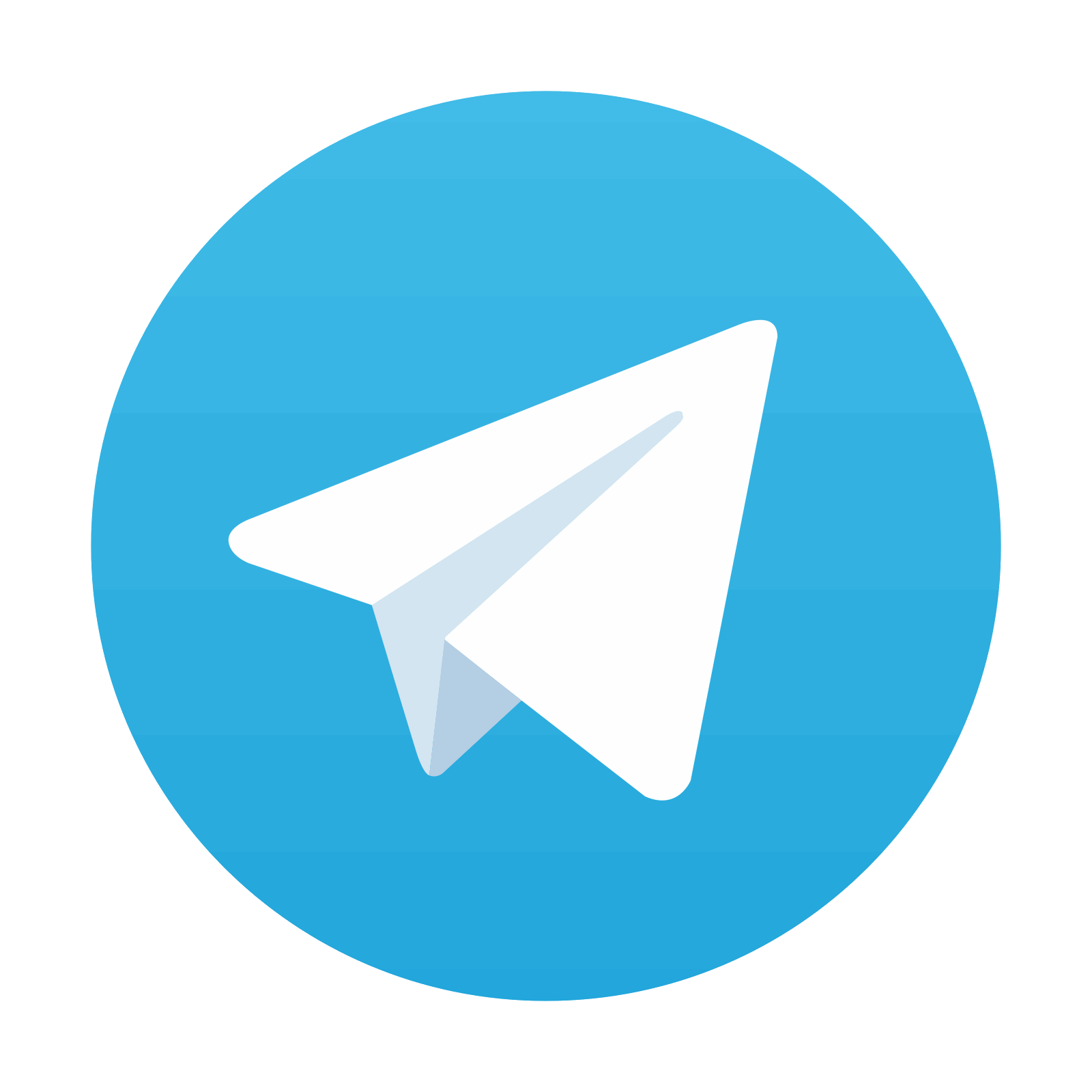
Stay updated, free articles. Join our Telegram channel

Full access? Get Clinical Tree
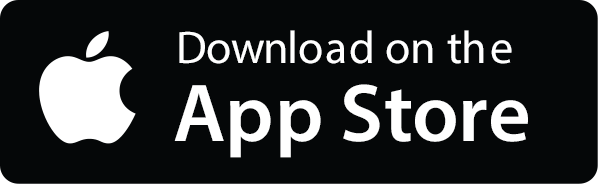
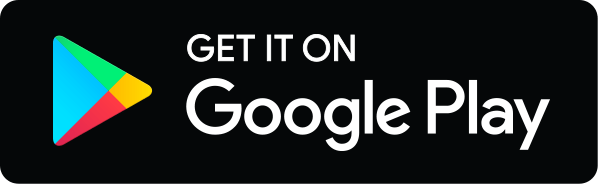