59.2.1 Flying Spot En Face (T-Scan) OCT
The differences between the longitudinal or axial priority scanning and en face or transverse priority scanning are presented below.
59.2.1.1 A-Scan-Based B-Scan
B-scan OCT images, as the first OCT image generated [3], are analogous to ultrasound B-scans and are obtained by collecting A-scans (Fig. 59.1) at adjacent transverse positions, as shown in Fig. 59.2 (top). The A-scans are one-dimensional sample reflectivity profiles acquired along the depth direction. The transverse scanning is performed in a transverse direction orthogonal to the axial direction (along the X or Y directions or can follow a circular pattern at constant radius ρ in polar coordinates in Fig. 59.1) at a slower pace than A-scanning as illustrated in Fig. 59.2 (top). The majority of reports on time domain OCT [4] in literature refer to this method of operation.
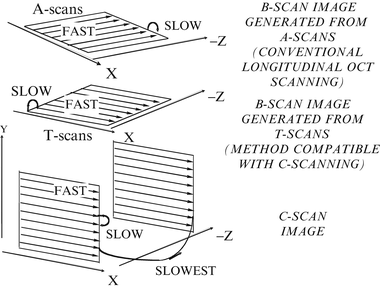
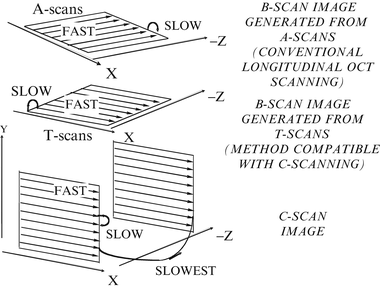
Fig. 59.2
Different modes of operation of the three scanners in a flying spot OCT system. (Reproduced with permission from OSA [19])
59.2.1.2 T-Scan-Based B-Scan
In this case, the transverse scanners sample the target at a fast rate [5, 6], and each line in the raster is a reflectivity profile in the lateral direction (T-scan) at a given depth in the sample as shown in Fig. 59.2 (middle). Transverse scanning can be performed either parallel to the X- or Y-axes, radially (at a given value of the polar angle θ) or circularly (at a given polar radius ρ). During acquisition of one T-scan, the position of the axial scanner is fixed. The example in Fig. 59.2 (middle) illustrates the generation of a B-scan from adjacent T-scans. In this particular example, the T-scans are parallel to the X-axis, while the axial scanner advances at a slower rate in depth, along the Z-axis.
59.2.1.3 C-Scan
The OCT T-scanning procedure has a net advantage over OCT A-scanning as it also allows acquisition of C-scans, i.e., of transverse or en face OCT images at a given depth determined by the length of the reference path as illustrated in Fig. 59.2 (bottom). C-scans are transversal sections of the sample made from T-scans, usually performed along the X- or Y-axes, repeated at adjacent locations along the other orthogonal transverse direction (Y, X). The repetition of T-scans along the other transverse coordinate is performed at a slower rate (frame rate) as shown in Fig. 59.2 (bottom). C-scans can be acquired at successive axial positions, either by advancing the optical path difference in the OCT in steps after each complete transverse (XY) scan or continuously at a much slower speed than the frame rate. Such images can be created based on the path modulation introduced by the transversal scanners, as explained above, or by using a phase modulator or a frequency shifter. A fast en face OCT method (T-scan-based OCT method) was reported [7] using a stable high-frequency carrier (40 MHz) generated by use of an acousto-optic modulator and a resonant scanning mirror (4 kHz) for the priority scan (x-direction).
Using a spectral scanning delay line for depth scanning [8], capable of scanning in depth at the same rate as that of transversal scanning, a multimode OCT imaging system was demonstrated [9], capable of producing depth B(A) or en face B(T) cross-sectional images, as well as en face constant depth (C-scan) images. The time required to switch from one regime to the other is limited by the time required to operate the control software. Safety level calculations for maximum optical power allowed to the eye, in retinal imaging, showed that T-scan-based imaging, for lateral sizes larger than 100 pixels, allows at least 4 times more power to be delivered into the eye than in B(A) scan. Here the lateral pixel size is considered as 10–15 μm. For larger image size, of 500 pixels, even more power could be used in T-scan regime than in the A-scan regime to generate B-scans. This has immediate consequences in terms of signal-to-noise ratio.
59.3 Simultaneous En Face OCT and Confocal Imaging
As T-scans are instrumental in generating both time domain en face OCT scans and confocal images, there are similarities and complementarities between the two imaging principles when applied to imaging the eye:
1.
2.
In both en face OCT and SLO, the depth scanning (optical path change in case of the OCT and focus change in case of the SLO) is much slower than the T-scan rate (performed at the frame rate).
3.
The depth resolution in an SLO is limited by the eye aberrations, while the transversal resolution in OCT is affected by random interference effects from different scattering centers (speckle). Therefore there may be some compensating advantages to combining SLO with OCT technology for retinal imaging.
4.
The higher depth resolution of OCT C-scan images tends to make structures in the retina appear more fragmented. In a single C-scan image, tissue features may also appear distorted by tilt making interpretation somehow difficult. However, ophthalmologists have extensive experience with the en face perspective of ocular disease as seen in the SLO images. In order to take advantage of their familiarity with the SLO imaging perspective in the interpretation of the OCT transversal images, it is useful to acquire and display pairs of OCT C-scans and SLO images simultaneously. High-quality SLO images can be used as reference for interpreting retinal C-scan OCT data. Additionally, the integration of confocal imaging in an OCT instrument facilitates the targeting of B-scan OCT acquisition which otherwise would require auxiliary imaging equipment to select the lateral location in the retina to be subsequently imaged with OCT.
59.3.1 OCT/SLO
The combination of confocal imaging and interferometry has already been discussed in microscopy [11], and a comparison between confocal and OCT imaging through scattering media has also been reported [12]. However, (i) the object here is the tissue, which imposes a safety power limit and requires special interface optics, and (ii) the same low coherence source is used for both confocal and interferometer channels with implications in terms of the obtainable signal-to-noise ratio.
A possible configuration combining OCT with confocal microscopy for the eye [13, 14] is shown in Fig. 59.3. Light from a pigtailed superluminescent diode, SLD, is injected into a single-mode directional coupler, DC1. Light in the object arm propagates via the microscope objective C3 and plate beam splitter PB to a scanning mirror pair, MX, MY. A lens L1 images the galvanometer scanner aperture onto the eye pupil, EL. The reference beam is directed via microscope objectives C1 and C2 and a corner cube CC to a coupler DC2. The corner cube CC is mounted on a computer-controlled translation stage, TS, used to perform axial scanning. The light back reflected from the retina and transferred via couplers DC1 to DC2 interferes with the reference signal in the coupler DC2. Two silicon pin photodiodes, PD1 and PD2, in a balanced [15] configuration are used to detect the OCT signal, which is then demodulated by the demodulator block, DMOD, whose output is connected to the OCT channel input of the dual input variable scan frame grabber, VSG, controlled by a personal computer, PC.
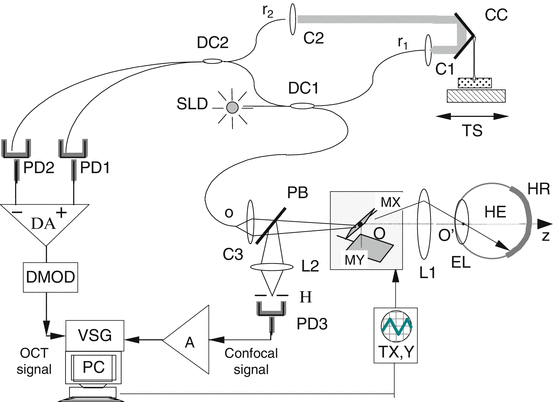
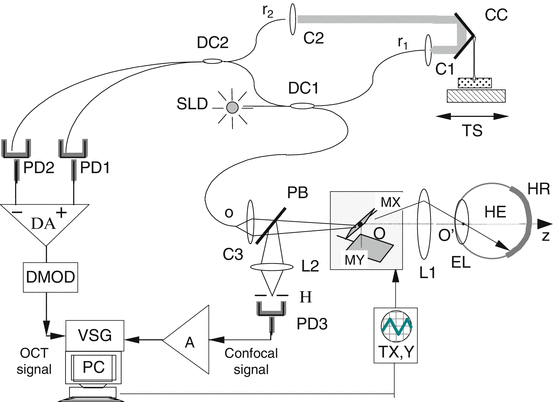
Fig. 59.3
Detailed schematic diagram of the time domain OCT/SLO apparatus using a plate beam splitter to divert light to a confocal receiver. SLD superluminescent diode; C1, C2, C3 microscope objectives; DC1, DC2 directional couplers; TS computer-controlled translation stage; CC corner cube; M1, M2 mirrors; MX, MY orthogonal axes galvanometer mirrors; TX(Y) ramp generators; DMOD demodulation block; L1 convergent lens; PD1, PD2 photodetectors; DA differential amplifier; PD3 and A photodetector and amplifier, respectively, for the confocal receiver; H pinhole; PB plate beam splitter; HE patient’s eye; EL eye lens; HR human retina; PC personal computer; VSG dual-input variable scan frame grabber (Reproduced by permission of the Institution of Engineering and Technology from [13])
A separate confocal receiver is built [16]. This consists of a plate beam splitter, PB, which reflects a fraction of the light backscattered by the retina via a focusing lens, L2, and a pinhole, H, to an avalanche photodiode, PD3, whose output is connected to the SLO channel input of VSG.
Ramp generators TX,Y drive the galvanometer scanner mirrors MX and MY and also trigger signal acquisition by the frame grabber.
Retinal images of a healthy eye, provided by the system described above, operating in the B- and C-scan regimes of operation, are shown in Fig. 59.4. In the left, images in the C-scan regime are shown, while in the right, images obtained in the B-scan regime.
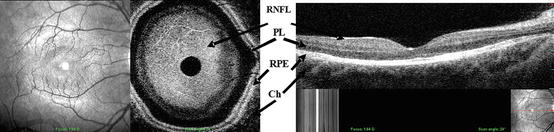
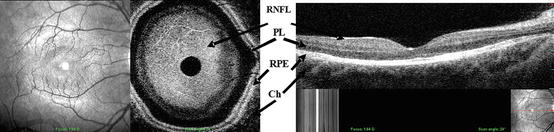
Fig. 59.4
Retinal images acquired with the OCT/confocal system. Left: C-scan regime with SLO image left and the OCT image right, collected at the level of the retinal nerve fiber layer, RNFL (which appears as a bright doughnut-shaped region in the center of the image); PL (dark): photoreceptor layer; RPE (bright): retinal pigment epithelium; Ch (bright): choroid. Right: B-scan regime with confocal images below, (left: simultaneous with the B-scanning and right: immediately preceding the B-scan acquisition). Lateral size in all images: 25°; in the B-scan regime image on the right, vertical size in the B-scan OCT image is 2 mm depth measured in air, while in the SLO image left bottom, it corresponds to the acquisition time of the B-scan OCT image, 0.5 s. The lateral variations of the shades in the SLO image indicate lateral movements of the eye during the acquisition. The retinal layers are clearly discernible in the OCT image and bears strong resemblance to histology [54]. (Republished with permission from Springer Science+Business Media, [55])
59.3.2 En Face Scanning Allows High Transversal Resolution
Due to T-scanning, the B-scan time domain OCT images acquired with the en face method are continuous along the line in the raster, which improves their quality. This is not the case with standard OCT B-scan OCT images generated using fast axial scanning, where the lateral scanning is discrete. Since the scanning direction parallels the anatomic fabric of the retina, connected structures or associations between scattering points in the transverse section are better conserved by transverse scanning. This ultimately ensures the visualization of small lesions above, within, or beneath the retina which might otherwise be chopped up and lost in the reconstruction of the image based on depth priority scanning OCT.
59.3.3 Synergy Between the Channels
The confocal image with its pixel-to-pixel correspondence to the C-scan OCT holds additional value for data acquisition. For small movements, the confocal image can be used to track lateral eye movements between frames and for subsequent transversal alignment of OCT images in a stack of C-scans collected from different depths. In cases of large saccadic eye movements and blinks, the confocal image gives a clear indication of the OCT frames which need to be eliminated from a collected stack. The first artifact-free confocal image in the stack is used as a reference for the aligning procedure.
The SLO image acquired in the B-scanning regime has the appearance of a pattern of parallel vertical traces. Each horizontal line in this image corresponds to a depth position in the OCT B-scan. Lateral eye motion during B-scan acquisition causes lateral deviations of the vertical trace pattern in the SLO image. For example, in Fig. 59.4 right and Fig. 59.5, movements of the eye are indicated by lateral shifts of the traces in the SLO image (lower left box), while the system operates in the B-scan regime. The recorded deviations in the vertical trace pattern in the SLO image can easily be utilized to correct the lateral shift of the lines in the B-scan OCT image (above).
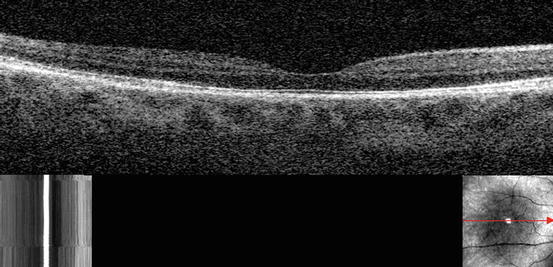
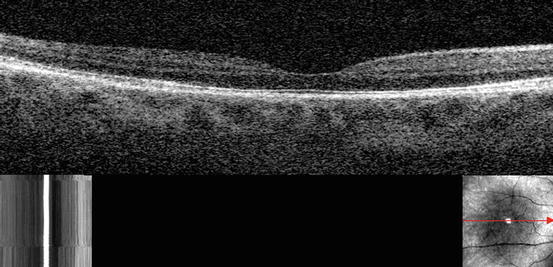
Fig. 59.5
B-scan section of the retina appears slightly elongated due to mild lateral eye movement during the scan. The SLO image in the lower left corner shows laterally displaced vertical trace pattern due to lateral eye movement during B-scan acquisition. The lower right image was collected in the C-scanning regime immediately before initiating the B-scan capture. (Republished with permission from Springer Science+Business Media, [55])
59.3.4 3D Imaging
3D imaging of the retina using SLO [17] is already common in clinical applications. En face sections collected from different depths [18] can be used to construct a 3D profile of the retina which can subsequently aid ophthalmologists in their clinical evaluations. In the same way, an OCT/confocal system can be used for 3D retinal imaging, however, with en face sections as thin as allowed by the source spectral width. To collect the reflectivity distribution from a volume in the retina, the OCT/confocal system is operated in the C-scan regime collecting en face images at different depths. Ideally, the depth interval between successive frames should be much smaller than the system axial resolution, and the depth change applied only after the entire C-scan image was collected. However, in practice, to speed up the acquisition, the translation stage in Fig. 59.3 is moved continuously, and the depth interval is set slightly larger than the OCT depth resolution [19]. For instance, using 20 μm axial separation between successive OCT C-scans, 60 frames of image pairs from a volume corresponding to a total depth of 1.2 mm in air (sufficient to cover in the axial direction the optic nerve region in the retina) can be acquired in 30 s when operating at 2 Hz frame rate. After acquisition, the images can be aligned transversally using the first confocal image (or the first motion artifact-free confocal image), and the stack of OCT C-scans can be used to construct a 3D OCT volume of the retina [20]. 3D retinal OCT imaging is more convenient than aligning individual OCT C-scans acquired at different depths.
59.3.5 Topography
Topography of the fundus is difficult to perform using A-scan-based B-scans since it requires interpolation in the en face plan [21]. Topographic maps are normally presented from an en face perspective which suggests that stacks of C-scan OCT images could be a natural choice for topography. This procedure was demonstrated in a previous report [22]. Based on en face OCT, topography rendering should be similar to the method using a confocal SLO [23]. Topography of a normal macula is shown in Fig. 59.6 obtained using en face OCT.
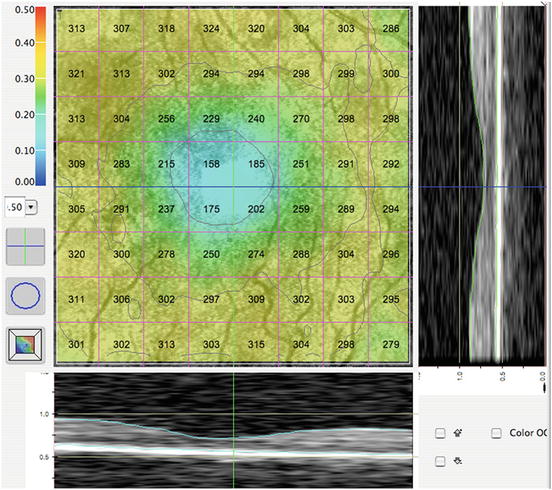
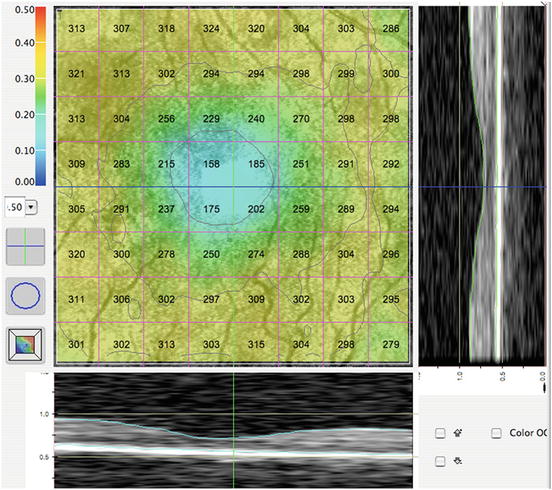
Fig. 59.6
Topographic map of a normal macula. 200 C-scans are aligned according to the vascular pattern with a grid showing the average thickness within each box superimposed on the SLO image. The B-scans below and on the right side of the map are reconstructed from the C-scan stack. Their lateral location is indicated by the green vertical and aqua horizontal cursors seen on the map. (Republished with permission from Springer Science+Business Media, [55])
59.3.6 New Challenges
New imaging technology brings new perspectives to the clinician, but also brings the requirement for new interpretations of imaging data. En face OCT is no exception to this rule. The higher depth resolution of OCT has the effect of fragmenting the C-scan OCT image [24] in comparison to a SLO image. As the imaging proceeds at a few frames a second, the inherent eye movements may result in significant changes in the size of fragments sampled from the tissue. The fragmentation is especially visible when imaging tissue tilted with respect to the system optical axis. The image on the right in Fig. 59.7 shows the challenges in interpreting and using en face OCT images. First, it is composed of structural features which are discontinuous and unfamiliar in shape.
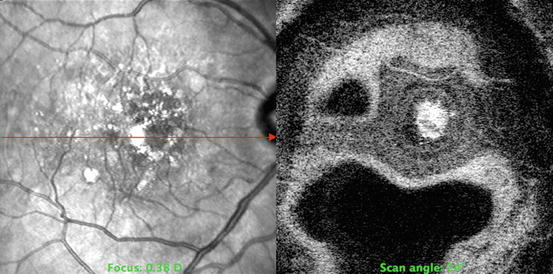
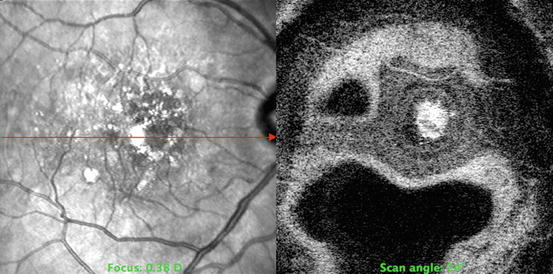
Fig. 59.7
Age-related macular degeneration with drusen and a choroidal neovascular membrane. The SLO image on the left demonstrates the superficial appearance lines of pigmentary change and scattered drusen. The OCT C-scan on the right demonstrates a tilting of the eye upward so that the image of the vitreous in the lower part of the image appears as a black chef’s hat surrounded by the highly reflective nerve fiber layer which appears white. Beneath the macular drusen lies a serous detachment surrounding a highly reflective white choroidal membrane. (Republished with permission from Springer Science+Business Media, [55])
Second, variations in tissue inclination with respect to the coherence wave surface alter the sampling of structures within the depth in the retina [25], producing novel section orientations with little resemblance to the ophthalmoscopic appearance of the fundus. The bright patches in the OCT image represent the intersection of the surface of optical path difference (OPD) = 0 with the tissue. Due to the specific way in which the retina is scanned, with the fan of rays converging onto the eye pupil, the surface of OPD = 0 is an arc circle with the center at the eye pupil. When we explore the depth, we practically change the radius of the arc. If the arc has a small radius, it may just only intersect the top of the optic nerve or the fovea with the rest of the arc in the aqueous/vitreous. The radius of the arc is increased by extending the length of the reference arm of the interferometer to explore the retina down to the level of the RPE and choroid. The orientation of the retinal tissue at the back of the eye is not perfectly circular, and this complicates the interpretation of the image even further.
Another confounding phenomenon noted is that despite the en face scanning orientation, tilting movements of the eye result in images which display several depths within a single section and may appear like a longitudinal OCT image. These two effects, (i) fragmentation and (ii) depth structures displayed in the C-scan images, are present in a confocal SLO with high depth resolution as well, however, at a scale where they are regularly discarded. In a confocal SLO, the images do not look fragmented, and the depth structure is barely visible due to the coarse depth resolution, ∼0.3 mm, comparable to the retinal thickness. Going in and out of focus results in a smooth transition from dark to bright areas in the image. Both problems mentioned above are brought about by the high depth resolution of OCT. We address the fragmentation problem by providing the confocal image, which guides the user, and by collecting many en face images at different depths and subsequently building the 3D profile. The other problem that the en face imaging may also display the depth structure requires further development of the interpretation process.
59.3.7 Clinical Use of the OCT/Ophthalmoscope: Pattern Recognition
Several prototypes of OCT/SLO systems were used to study a variety of clinic pathologies [26] including age-related macular degeneration, central serous retinopathy, macular hole, macular pucker, cystoid macular edema, diabetic maculopathy, and macular trauma. Specific recognizable patterns [27] in the en face OCT images were identified which correlate to a variety of anatomic disease entities. The dual display is particularly helpful to the clinician in correlating the deeper morphology to the surface appearance and facilitating interpretation of the often challenging new images. The subject interface is comprised of a standard chin rest assembly which provides stability and comfort but allows some movement effects in the images to occur.
Figure 59.8 is a case of retinal angiomatous proliferation (RAP). Three pairs of C-scan OCT and confocal images are shown on the left. Each was taken at different depths as indicated by the lines in the B-scan OCT which was sampled along the line shown in the SLO image. By collecting a stack of OCT/SLO images, the volume of the retinal elevation could be evaluated.
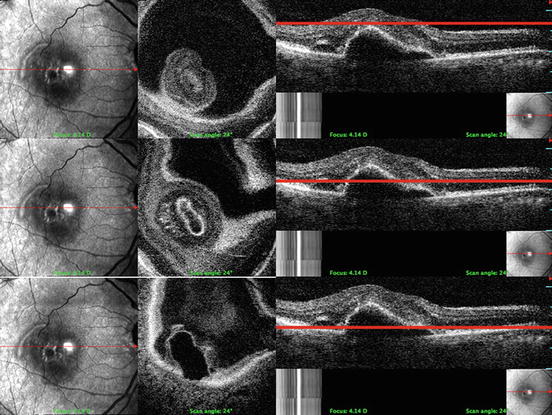
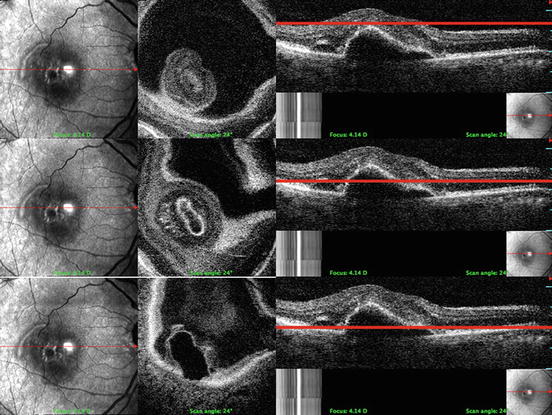
Fig. 59.8
Retinal angiomatous proliferation (RAP). The top image set demonstrates a C-scan slice superficial to the retinal pigment epithelial detachment. The small ovoid is the peak of the retinal elevation and is surrounded by a black circular space which is the vitreous. The middle slice cuts through the pigment epithelial detachment and the intraretinal neovascular complex. In the lowest series of images, the C-scan outlines the base of the pigment epithelial detachment and shows the choroid at the edges. (Republished with permission from Springer Science+Business Media, [55])
Accurate volume assessment requires collection of a serial stack of C-scans at progressive depths. While the current instrument is equipped with such a feature, we had variable success using it in eyes with pathology due to their limited ability to maintain consistent fixation. We have found another feature of the system more useful, that which allows an easy switch between the two modes, B-scan and C-scan. Successive C-scan cuts viewed in conjunction with selected B-scan images allow the viewer to create a good mental picture of the three-dimensional aspects of the lesion despite the eye movements.
Interpretation of these images requires some careful thought. Due to the inclination of the walls of the thickened retina, the C-scan images often display structures from different depths, as visible in the B-scan OCT images on the right. As such, the C-scan OCT images alone may lead to a wrong interpretation. Comparing them with either of the B-scan image in Fig. 59.8 is helpful in establishing the orientation and the depths of features depicted in individual scans. The images in Fig. 59.8 show the two challenging features of the OCT C-scan imaging: patchy fragmented planes and display of structures from multiple depths for the tilted parts of the tissue. The elongated parts visible in the B-scan OCT images in Fig. 59.8 show up as circles of different intensities in the C-scan images, which indicate different structure in depth due to the discontinuity of optical parameters such as the index of refraction and backscattering coefficient. When we follow the cuts along the straight lines indicated, we can infer the intensity level in the corresponding part of the C-scan OCT image. Evaluating how the radius of the dark circles in Fig. 59.8 varies with depth, the volume of the lesion can be easily inferred.
Similar challenges are presented in the interpretation of the images in Fig. 59.9, a case of parafoveal telangiectasia. Three pairs of C-scan OCT/SLO images acquired at increasing depth positions are shown. For ease in interpretation, the B-scan OCT is displayed on the right-hand side of each OCT/SLO pair. Here, the deepest C-scan OCT image (bottom pair) samples the choroid just touching the RPE. At this level only the shadow of the telangiectatic exudates can be seen. In the first raw, the dark circle in the C-scan is created by slicing through the foveal cone. The C-scan image in the middle raw cuts through the foveal floor which is the roof of an intrafoveal cyst. White edema residues are seen at the edge of the cone. The confocal image in each pair on the left displays the clinical appearance of the surface which reveals limited detail of the underlying pathological features.
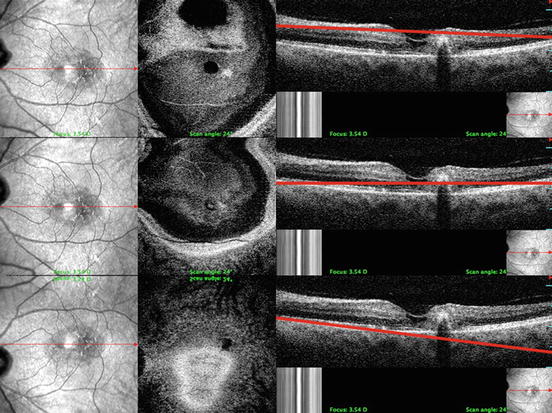
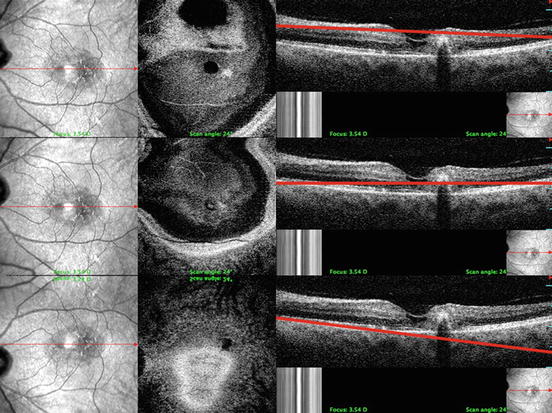
Fig. 59.9
Parafoveal telangiectasia. The lesion is temporal to the foveal depression. A series of confocal images and paired C-scan OCT images taken at different depths are shown. The B-scan OCT on the right of each pair shows the level and angle of the C-scan cut. Note the shadowing beneath the hyperreflective exudative lesion. (Republished with permission from Springer Science+Business Media, [55])
Figure 59.10 demonstrates further how C-scan OCT images can enhance the understanding of anatomic relationships between different aspects of pathological processes that effect complex retinal structure. The images presented are from a case of exudative age-related macular degeneration with a choroidal neovascular membrane [28] and overlying cystoid macular edema. While these two aspects of the lesion can be identified in the cross-sectional aspect of the B-scan OCT, separate C-scan sections reveal a more complete picture at the various interfaces of the components. The transverse direction of scanning is particularly effective at differentiating structures oriented horizontally.
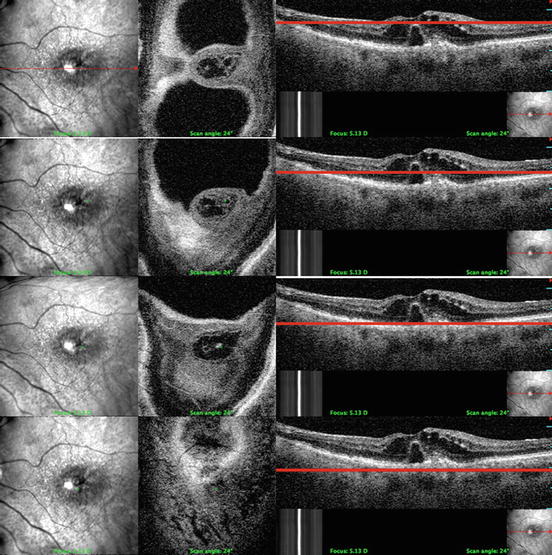
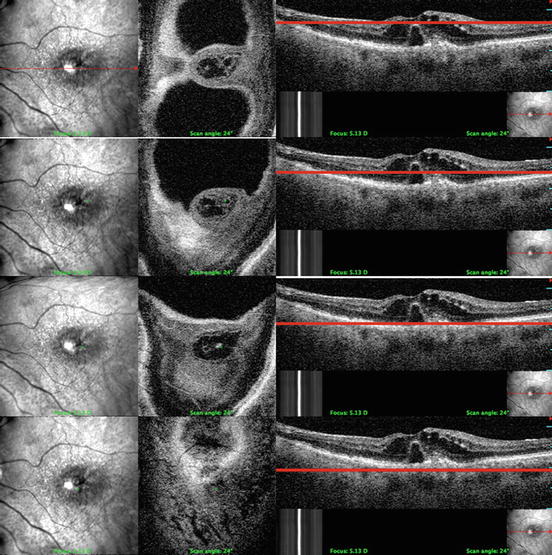
Fig. 59.10
Choroidal neovascular membrane with adjacent RPE detachment and overlying cystoid macular edema. The B-scan OCT images on the right feature red lines which indicate the level of the coronal slices. (Republished with permission from Springer Science+Business Media, [55])
The confocal view of the retina seen in each pair on the left demonstrates a diffusely increased reflex over a thickened central macula. From top to bottom, the image pairs demonstrate progressively deeper C-scan sections with paired B-scans marked with lines indicating the depth. The first section is taken though the inner retina and cuts through multiple perifoveal cysts. The section can be localized to the nerve fiber layer since it is at the same level of the retinal venule, which appears bright in the section. The second section cuts through the lower aspect of the cystic area which is seen surrounding the upper part of the neovascular complex. In this section the venule outline is dark since it is a shadow cast from the layer above. The third section cuts through the center of neovascular complex which appears highly reflective in the image. The bottom section shows the neovascular complex as a cystic area within the RPE bright ring surrounded by darker choroidal vessels.
Another important example of pathology, which demonstrates the clinical utility of the C-scan approach over the simple planar imaging of B-scan OCT, is seen in the case of a macular pucker [29] (Fig. 59.11). The confocal image shows the dragging of retinal blood vessels and loss of clarity characteristic of the epiretinal membranes that distort the macula. The B-scan OCT images have become standard clinical tools for displaying the bunching up of the vitreoretinal interface under the cellophane thin, but contracting blanketing membrane. The difficulty clinicians often encounter in approaching these membranes surgically is in defining their lateral extent in order to plan minimally damaging peeling. The C-scan sections in the pairs in the three raws highlight the tentacle-like extensions of the overlying tissue and help define the thickness and spread of irregularly shaped growth. The C-scan OCT image is patchy and displays fragments of depth structure for the tilted parts of the tissue.
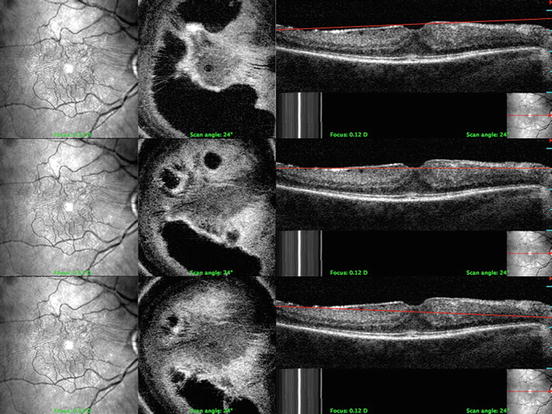
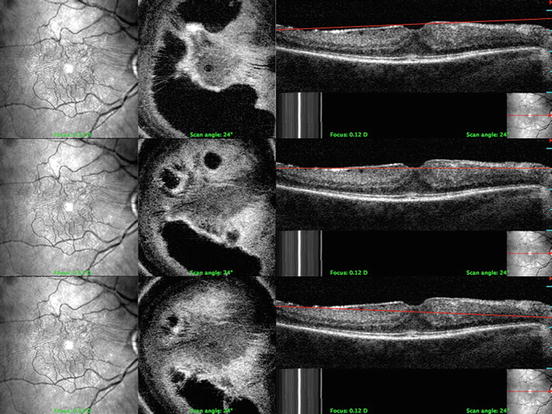
Fig. 59.11
Epiretinal membrane. The C-scan OCT images reveal the extent of the membrane and the presence of multiple cystic formations within the periphery of the membrane. Slight inferior tilting of the eye resulted in capture of irregular oval-shaped areas of vitreous within the three slice pairs shown. B-scan OCT images on the right show a red line which approximates the level and tilt of the scan angle. (Republished with permission from Springer Science+Business Media, [55])
59.3.8 Improved Resolution Using Ultrahigh-Resolution C-Scan Imaging (RR, MV, PG)
59.3.8.1 En Face Ultrahigh-Resolution OCT
Using a broadband light source in a system similar to the first generation of OCT/SLO systems allows for higher-resolution imaging and display of distinctive features. A SLO/en face ultrahigh-resolution (UHR) OCT prototype can acquire B- and C-scan OCT images with an axial resolution of about 3 μm and a lateral resolution around 15 μm. The system is based on a low-cost broadband lighter (Superlum, Russia) with a bandwidth (FWHM) of 150 nm at a central wavelength of 890 nm. Polarization controller paddles are added to input ports of the coupler to match the states of polarization in the reference and sample arm. Dispersion imbalance, introduced by the interface optics and the eye refractive media, is also compensated [30].
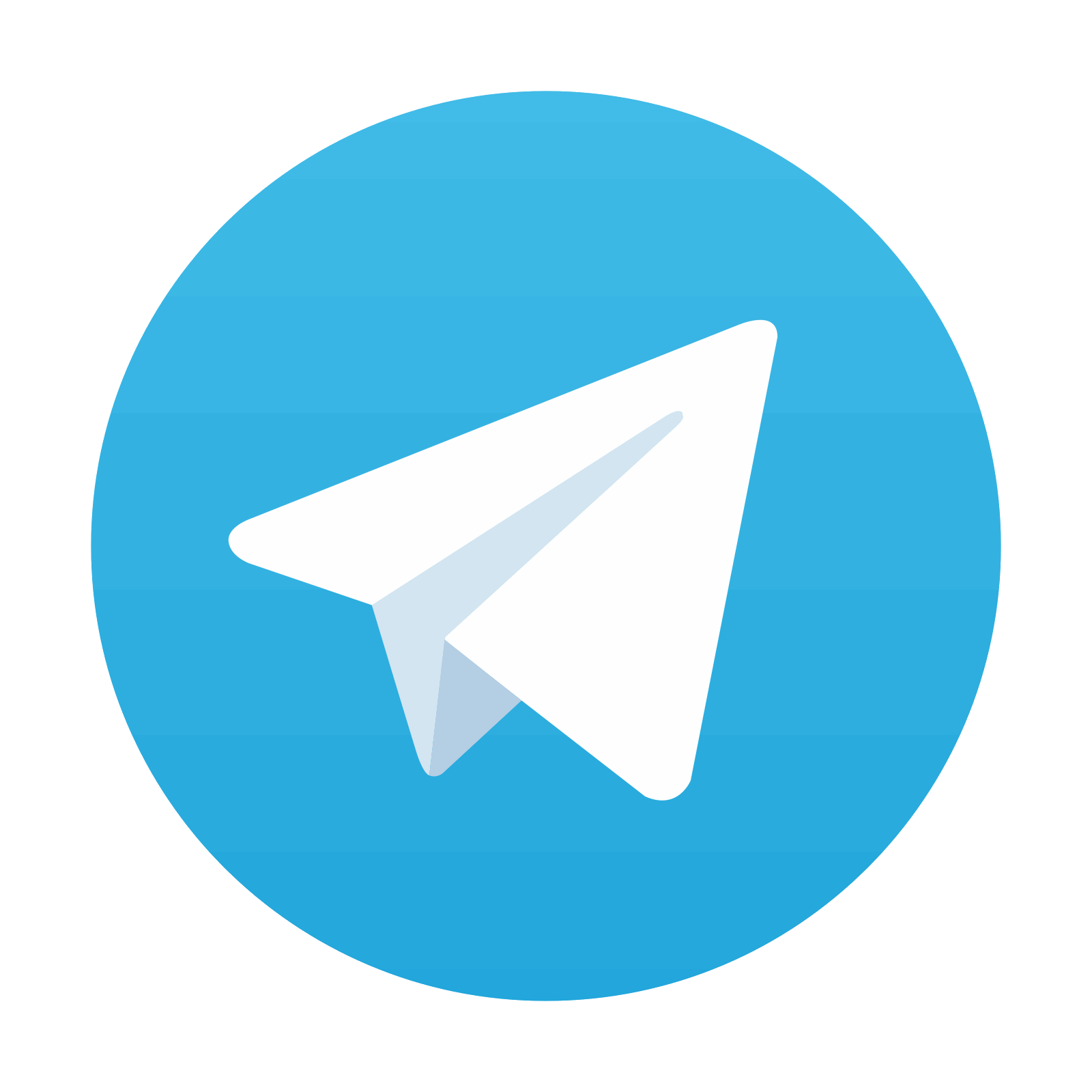
Stay updated, free articles. Join our Telegram channel

Full access? Get Clinical Tree
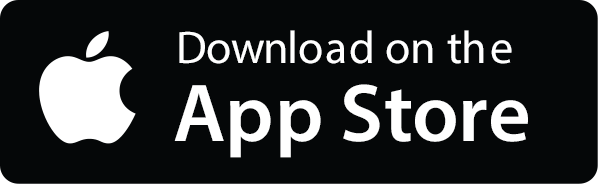
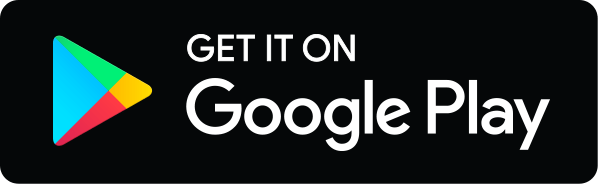