CHAPTER 133 Diagnostic Audiology
Audiologic Test Battery
In his book, Practical Observations on Aural Surgery and the Nature and Treatment of Diseases of the Ear,1 Wilde stated:
Wilde1 continued, “It is absolutely necessary, if we wish to watch the progress of a case not only to conduct these observations with great care, but also to take a written note of the hearing distance the first and each subsequent time we see the patient.” Wilde also recommended placing the watch “gently between the teeth of the patient, and the amount of hearing thus obtained likewise noted”—an early attempt at bone conduction testing.
Founded on such clear, practical thinking, the clinical science of audiology has advanced through the availability of instrumentation that accurately quantifies the sensitivity and acuity of human hearing. In his book, Disease of the Ear,2 Dench stated that “it is scarcely necessary to mention the more complicated instruments which from time to time have been devised for determining quantitative audition. Their use has never become universal on account of the complex construction.” Dench2 described and illustrated the electric audiometer developed by Urbantschitsh in 1890—a device that extended technology made available by the invention of the telephone. He further discussed the phenomena of crossover and interaural attenuation, cautioning against errors that may occur when a loud sound is presented to a profoundly deaf ear in the presence of a normal-hearing contralateral ear.
Tests for Hearing Sensitivity or Acuity
Pure-tone audiometry is the most commonly used test for evaluating auditory sensitivity. Auditory pure-tone signals are delivered primarily through air conduction and bone conduction. The American National Standards Institute (ANSI)2a defines the threshold of audibility as “the minimum effective sound pressure level of an acoustic signal producing an auditory sensation ‘in a specified fraction of the trials.’ ” Most often, threshold is defined as the lowest signal intensity at which multiple presentations are detected 50% of the time.3
When used clinically, audiometric threshold data are most often displayed on a graphic plot called an audiogram (see Figs. 133-2 through 133-4). Various symbols are used to represent data obtained for the right and left ears by use of air-conducted and bone-conducted signals. The current audiogram representation was recommended by the American Speech, Language, and Hearing Association in 1974, and was adopted by ANSI S3.21-1978. Data are presented in hearing level (HL), which is calibrated to referent sound pressures (ANSI S3.6-1969, 1970) that represent the hearing sensitivity of normal young adults when tested under reasonably quiet test conditions. An audiogram represents a patient’s ability to hear sounds compared with the hearing sensitivity of a group of normal young adults.
Pure-Tone Air-Conduction Testing
Pure-tone air-conduction thresholds measure the function of the total hearing system, including the external, middle, and inner ear. In typical audiometric testing, pure tones that range in octave spacings from 250 to 8000 Hz are presented to the listener by headphones or insert earphones. Threshold is usually determined by the use of a version of the Hughson-Westlake “ascending method,”4 in which sounds are initially presented well above threshold, and are then presented in decreasing steps of 10 to 15 dB until the sound is inaudible. The tone is increased in “up 5-dB, down 10-dB steps” until the single HL at which a response is obtained three times is reached.5
Because air-conduction thresholds measure the acuity of the entire hearing system, when evaluated alone they provide little information regarding the etiology of hearing loss and specific auditory pathology. When examined in conjunction with thresholds obtained by bone-conduction testing, however, they help determine the type and the severity of the hearing loss. When plotted on an audiogram, pure-tone thresholds also provide information regarding the severity of the hearing loss. Thresholds that fall into the 0- to 25-dB range are considered normal, whereas thresholds greater than 25 dB represent various levels of hearing loss (see Fig. 133-1).
Pure-Tone Bone-Conduction Testing
The location of the bone-conduction thresholds on the audiogram helps determine the severity of the hearing loss (Figs. 133-1). In bone-conduction testing, a bone oscillator is typically placed on the mastoid process. Although this placement does not guarantee that the responses obtained are from the ear located on the side on which the oscillator was placed, such placement provides an enhanced dynamic range compared with other placements, such as at the frontal bone.6 Most audiometers currently in use are calibrated for placement of the vibrator on the mastoid.
The relationship between air-conduction and bone-conduction thresholds is used to determine the type of hearing loss. When air-conduction thresholds are elevated relative to normal bone-conduction thresholds—a phenomenon referred to as an air-bone gap—the loss is classified as conductive (Fig. 133-2). When air-conduction and bone-conduction thresholds indicate the same amount of hearing loss, the loss is classified as sensorineural (Fig. 133-3). Finally, when air-conduction thresholds are elevated relative to abnormal bone-conduction thresholds, the loss is classified as mixed (Fig. 133-4).
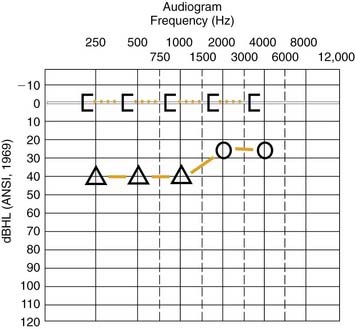
Figure 133-2. Example of an audiogram characterized by an air-bone gap indicating a conductive hearing loss.
Speech Testing
Another essential component of the audiologic test battery is the evaluation of the listener’s ability to detect and recognize speech. Three speech tests are commonly included in the audiologic test battery: determination of the speech detection threshold (SDT), determination of the speech reception threshold (SRT), and speech discrimination or recognition. The SDT indicates the intensity level at which a listener can barely discern the presence of a speech signal 50% of the time.7 With such a task, the listener is not required to recognize the stimulus, but is merely asked to acknowledge its presence. Conversely, the SRT represents the intensity level at which a listener can repeat 50% of the speech material.7 In contrast to the SDT, the SRT requires the listener to repeat the word that was presented. The SRT is usually 8 to 9 dB higher than the SDT,8 whereas the SDT usually coincides with the pure-tone average (PTA), an average of the pure-tone thresholds obtained at 500 Hz, 1000 Hz, and 2000 Hz. The SDT and SRT can be obtained using either air conduction or bone conduction.
Determination of speech discrimination is a more complex task than determination of either SDT or SRT. Speech discrimination is an important part of the audiologic test battery because it provides information regarding the listener’s ability to recognize speech under well-controlled conditions. Speech discrimination test results are used in conjunction with the findings of other tests to help determine the differential diagnosis of hearing loss; provide information about the listener’s ability to communicate effectively; aid in decision making regarding candidacy for various surgical procedures; provide useful information about rehabilitative needs, including the effectiveness of hearing aids or cochlear implants; and provide information regarding central auditory function.7
Various materials and formats have been used to evaluate speech discrimination. The most commonly used as part of an adult audiometric test battery include monosyllabic words presented in an open-set format, such as the CID W-22 word lists9 and the Northwestern University Auditory Test No. 6 (NU-6).10 Although taped materials are optimal when evaluating speech discrimination, materials are often presented through live voice for rapidity and ease of administration. Speech discrimination materials are usually presented 50 dB above the patient’s SRT—a level that should be well within their audible range.
Patients with a conductive hearing loss frequently have excellent speech discrimination scores when test stimuli are presented at a sufficiently loud level. Patients with a cochlear sensory hearing loss often have reduced scores on speech discrimination tests, even when the stimuli presented are well within their audible range. Patients with lesions of the eighth cranial nerve or beyond tend to have lower speech discrimination scores than patients with a cochlear lesion site, and may have reduced speech discrimination in the presence of normal auditory pure-tone thresholds. The extreme of this phenomenon may be found in patients with cortical lesions who are unable to understand speech or any type of complex auditory signal.11
Masking
Interaural attenuation refers to the reduction in sound when it crosses from one ear to the other. The lower limit for interaural attenuation for bone-conduction testing is essentially 0 dB across frequencies.12,13 Because of this, masking should be used routinely with bone-conduction testing when threshold levels between ears are asymmetric. Additionally, because the signal primarily travels to the nontest ear through bone conduction, the need for masking arises when air-conduction thresholds for the test ear are poorer than the bone-conduction thresholds for the nontest ear. Masking is useful in such situations because it shifts the sensitivity of the cochlea of the nontest ear to prevent it from hearing the signal that is delivered to the test ear.
The need for masking with air-conducted signals depends on how the signal is delivered. Interaural attenuation ranges from 35 to 50 dB for stimuli delivered via headphones and 60 to 65 dB for earphones,14 depending on the frequency of the test signal, with a tendency for greater interaural attenuation at the higher frequencies.12 Insert earphones have less contact with the lateral temporal bone than standard headphones, so less sound energy is delivered contralaterally. Greater levels of effective masking to the nontest ear are possible, with less crossover occurring to the test ear, when insert earphones are used.
Masking should be used with air-conduction testing when the air-conduction threshold of the test ear and the air-conduction or bone-conduction threshold of the nontest ear differ by the interaural attenuation factor for the frequency being tested. When determining the SRT, masking should be used whenever the SRT of the test ear and the SRT, air-conduction PTA, or bone-conduction PTA of the nontest ear differ by 45 dB or more. With speech discrimination testing, masking should be used whenever the presentation level used to deliver stimuli to the test ear is 45 dB or more above the SRT, air-conduction PTA, or bone-conduction PTA of the nontest ear.12
The critical bandwidth concept dictates that the extent of threshold shift produced by a masking signal is affected by its frequency and intensity.12 The term effective masking refers to the intensity level of the test signal that is barely masked by presentation of a masking noise to the ipsilateral ear. When selecting a masking signal, the goal is to select a signal that provides the largest shift in threshold with the least intense noise. Because the effective masking level depends on the structure of the test signal, various types of noises are used for clinical masking. Narrow band noises centered around a specific frequency identical to that of the pure-tone test signal are most often used with pure-tone testing, whereas speech noise of a more complex nature is most often used when masking for speech tests.
Tests to Evaluate Middle Ear Function
The term acoustic immittance is used to refer to either acoustic admittance (the ease with which energy flows through a system) or acoustic impedance (total opposition to the flow of sound energy). Acoustic immittance measures are used clinically as screening and diagnostic tools for the identification and classification of peripheral (particularly middle ear) and central auditory disorders, and may be used as a tool to estimate auditory sensitivity objectively.15 The acoustic immittance measures used most often clinically include tympanometry and stapedial reflex measures.
A commonly used procedure is employed in classification schemes for tympanogram shapes. It was first described by Liden16 and later modified by Jerger,17 Jerger and colleagues,18 and Liden and colleagues.19 This classification of tympanogram shapes is described in Figure 133-5.
Acoustic immittance tools also provide information regarding the volume of air medial to the probe. This information, when used in conjunction with that derived from the evaluation of a tympanogram’s shape, can provide useful diagnostic data. Generally, ear canal volumes normally range from 0.5 to 1 mL in children and 0.6 to 2 mL in adults.20 Volume measurements greater than 2 mL in children and greater than 2.5 mL in adults usually indicate a tympanic membrane perforation or a patent pressure equalization tube21,22 because the volume measurement includes the ear canal and the tympanic cavity.
Stapedial reflex measures are also part of the middle ear test battery, and provide important and useful diagnostic information. The two most commonly used stapedial reflex measures include the acoustic reflex threshold and acoustic reflex decay. Both of these tests measure changes in tympanic membrane compliance caused by contraction of the stapedius muscle and are particularly useful for differentiation of cochlear and retrocochlear lesion sites. The acoustic reflex threshold test determines the softest level of sound that elicits stapedial muscle contraction. This normally occurs bilaterally after either ipsilateral or contralateral stimulation when a pure tone or noise is presented to a normal-hearing ear at levels of 70 to 100 dB HL.23 The neural network of the acoustic reflex is located in the lower brainstem. The contralateral acoustic reflex arc includes the acoustic nerve and ventral cochlear nucleus, the medial superior olive, the contralateral motor nucleus of cranial nerve VII, and the contralateral stapedius muscle.23
Any type of middle ear disorder prevents the tympanic membrane from showing a change in compliance when the stapedius muscle is contracted. Ipsilateral and bilateral reflexes are absent bilaterally when the patient has a conductive hearing loss in the test ear. In cochlear pathologic conditions, the acoustic reflex often occurs when the impaired ear is stimulated at a level of 60 dB or less, indicating the presence of loudness recruitment. As the cochlear loss increases to greater than 60 dB HL, however, the chance of observing an acoustic reflex threshold decreases.23 In most cases of retrocochlear pathology, the acoustic reflexes are absent when the involved ear is stimulated. If acoustic reflexes are present, an ear with a retrocochlear disease frequently is unable to sustain contraction of the stapedius muscle as measured with the acoustic reflex decay test. Finally, the absence of contralateral reflexes, with ipsilateral reflexes intact, may be seen in patients with brainstem disease as a result of the presence of a lesion in the area of the crossed brainstem pathways.23
Objective Tests for Differential Diagnostic Applications
Otoacoustic Emissions
Until more recently the term objective test, when referring to the auditory system, denoted exclusively the measurement of neuroelectric events associated with auditory stimulation, beginning with Davis’ discovery of the cortical auditory evoked potential in 1939.24 The discovery of the ABR by Jewett and Williston25 marked the beginning of a revolution in the clinical application of auditory electrodiagnostic tests. The ABR changed the way hearing was tested in newborns and infants, and provides physicians and audiologists with a reliable and informative neurodiagnostic tool and a reliable technique to monitor auditory function during surgery. With the discovery of the evoked otoacoustic emissions,26 it became possible to analyze and investigate auditory function from hair cells to the auditory cortex. Otoacoustic emissions are not electric events, however. Rather, they are audiofrequency signals that can be recorded with a sensitive microphone by use of sophisticated signal processing, and they are thought to reflect the motility of the cochlea’s outer hair cells.
The active motility of outer hair cells is thought to serve as an amplifier of the displacement of the cochlear partition, resulting in various forms of otoacoustic emissions as by-products that are detectable at low intensities.27 These acoustic by-products of outer hair cell motility are often referred to as cochlear echoes. Acoustic emissions may be divided into spontaneous emissions, which occur without acoustic stimulation of the ear, and evoked otoacoustic emissions, which represent a response to an acoustic stimulus delivered to the ear.28
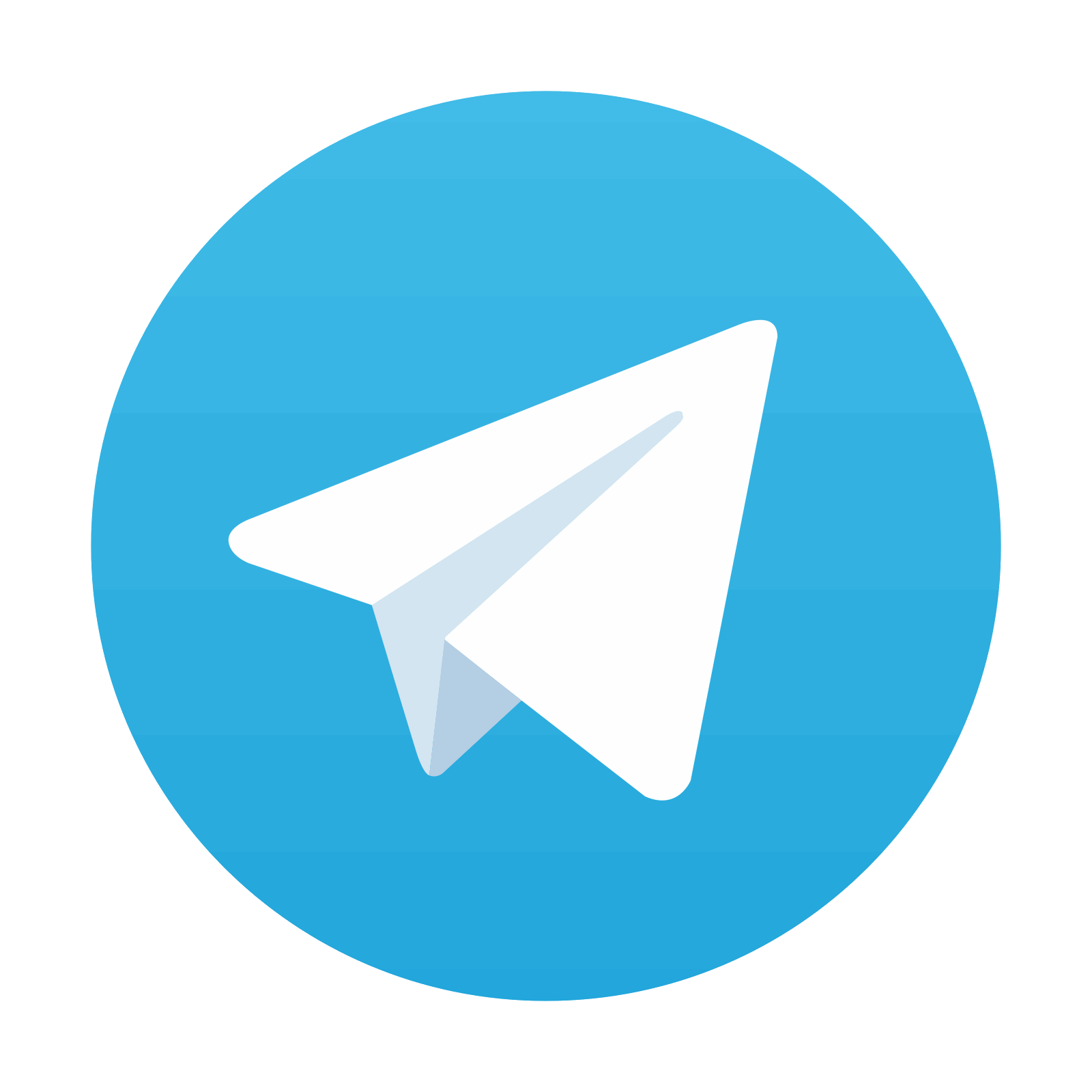
Stay updated, free articles. Join our Telegram channel

Full access? Get Clinical Tree
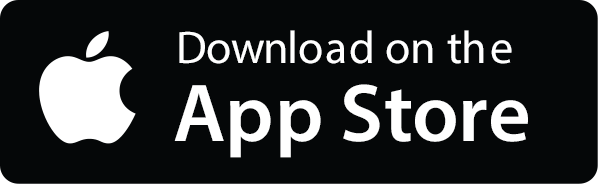
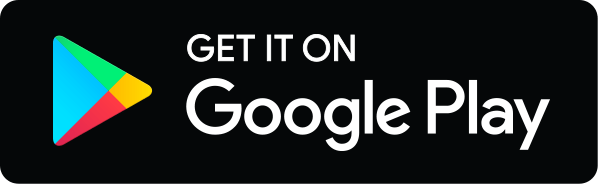