Fig. 26.1
Basic structures of breast tissue: lobule (a), milk duct (b), cutting cross milk duct with calcification (c), blood vessel (d), adipocytes (e), scar fibrous tissue (f), normal fibrous tissue (g), fibrous tissue surrounding carcinomatous cells in tumorous stroma (h)
In general, the structures containing spans of collagen (fibrous tissue and muscle) return an important backscattered signal (white on the FFOCM images). Instead, the epithelial structures (lobules and ducts) backscatter less and appear gray. Lobules structures (A) appear as dark gray and rounded. Longitudinal sections of mammary channels (B) appear as dark gray elongated structures with epithelial membrane of variable thickness. Their clear gray level in the thick elastic surrounding membrane distinguishes these glands, tangentially sectioned by FFOCM (C). We can also note the presence of calcifications that appear very white. Blood vessels in tangential section (D) do not have the thick epithelial membrane of ducts but are also distinguished by the presence of a thin elastic membrane. Adipocytes (E) are not backscattering features and appear as black rounded structures that differ only by the presence of the membrane that is more diffusive and appears white. The honeycomb structure characterizing adipose tissue is very well reproduced on the FFOCM images. Normal fibrous tissue (G) has a grainy and moderately backscattering level. In an area of cancerous stroma, fibrous tissue appears very different; it is composed of fine highly backscattering structures that appear white. In scar area (F) fibrous tissue is made of thick and wide spans that induce a low level of backscattering.
During a routine histological examination of a mastectomy, the nipple was excised and analyzed for the presence of Paget disease.
The FFOCM image of a nipple is shown in Fig. 26.2 with the corresponding histological image. The elongated structures are milk ducts cut transversely (E), and one can recognize their white elastic membrane. Sebaceous glands (D and F) are rounded structures along the nipple; they correspond to rounded structures on histology and scored on GS (B). The outer edge of the nipple is a layer of skin where one recognizes the dermoepidermal border by its reticulated appearance (C).
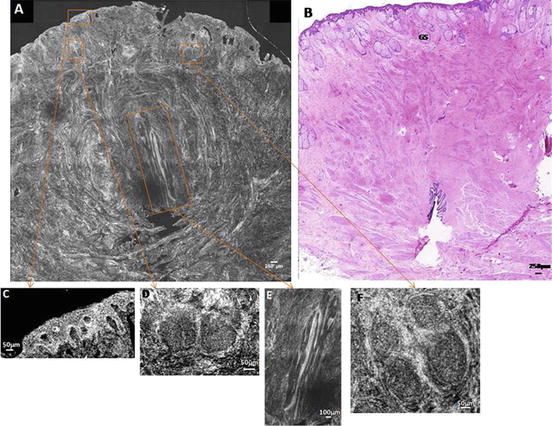
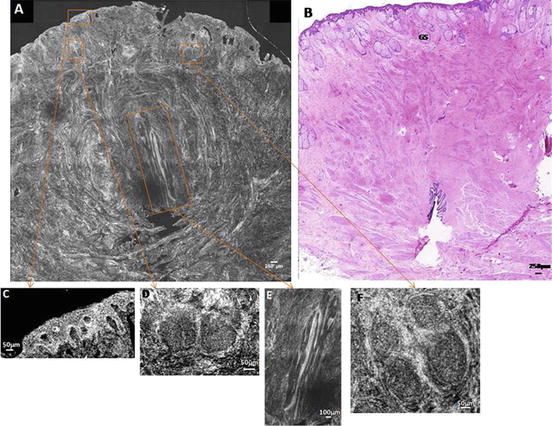
Fig. 26.2
Nipple: dermoepidermal junction of the outer skin layer (c), sebaceous glands (d) and (f), milk duct transected (e)
When imaging pathological tissues:
Malignant tumors can invade and destroy adjacent structures. A malignant tumor can spread through remote metastasis (cells detached from the original tumor to proliferate at a distance). Infiltrating cancer is when the proliferation of cancer cells exceeds specific limits histological and if metastatic cells spread to other organs by lymphatic channels.
Benign tumors are localized tumors. If cancer cells are contained within well-defined limits, the structure is called carcinoma in situ. However, such a tumor can develop into malignancy, which may be fatal if not treated.
Tumors that develop from glandular epithelial structures are called adenocarcinoma: If the spread is within a lobule and without exceeding the limits, we speak of in situ lobular carcinoma (often benign). If the tumor develops from a milk duct, it is called ductal adenocarcinoma; this tumor is malignant in most cases. The presence of a tumor in the breast results in the case of invasive cancer is revealed by a change in the appearance of breast tissue and of a structure having a stellar or nodular shape.
Examples of invasive cancers are represented in Figs. 26.3 and 26.4. Figure 26.3 is a tumor called stellar and Fig. 26.4 one nodular tumor. In both cases the fibrous and adipose tissue are separated; in the case of the stellar tumor, stroma tends to invade the adipose tissue (Fig. 26.3c). Fibrous tissue has a characteristic appearance of fine highly scattering spans (Fig. 26.3c, d), which is distinct from normal fibrous tissue (Fig. 26.4d). Adipocytes at the edge of the stroma (Fig. 26.3e) appear smaller and less rounded in form than in healthy tissue. In the case of nodular tumor, adipose tissue surrounds cancer cells that form a dense region of dark gray.
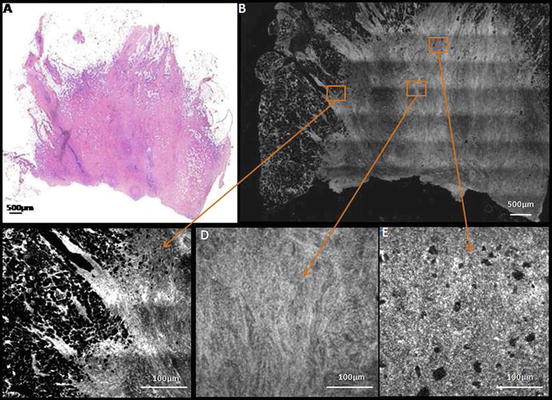
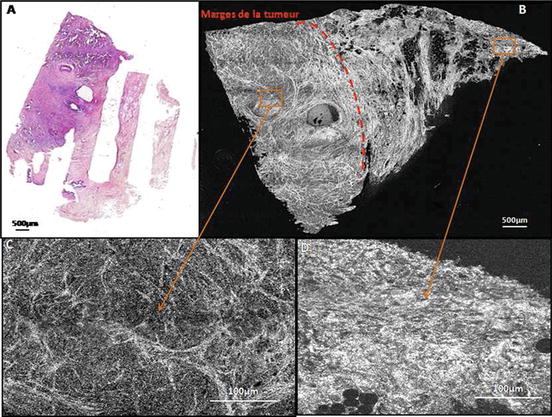
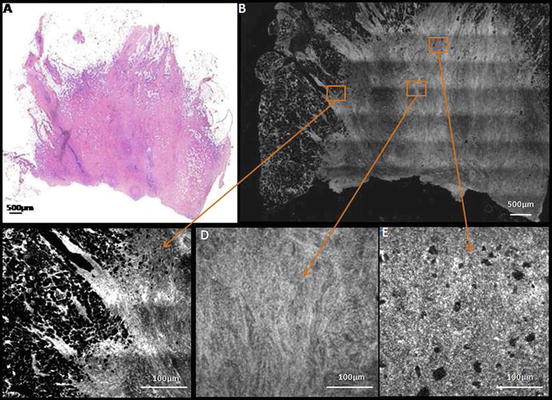
Fig. 26.3
Infiltrating carcinoma: stellar tumor (a, b). Invasion of adipose tissue (c), fibrous tissue tumor (d), small adipocytes, and deformed (e)
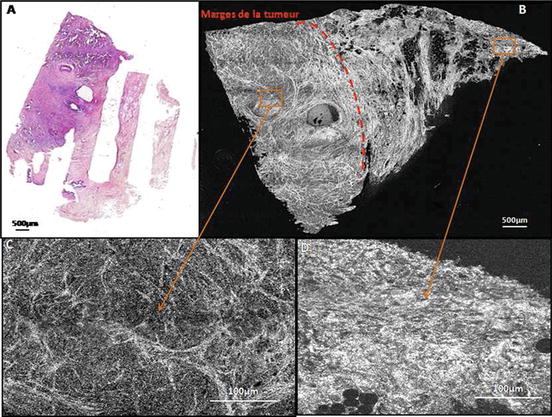
Fig. 26.4
Infiltrating carcinoma: tumor nodule (a, b). Fibrous tissue surrounding cancer cells (c), normal fibrous tissue (d)
The difference in appearance of the fibrous web can be used to mark the margins of the tumor. A circular channel dilated with secretions in the lumen is visible in the center of the sample. These changes in appearance of the fibrous tissue and the shape of the adipocytes are highly visible on the images FFOCM, while it does not appear clearly on the histological images. These characteristics are used as criteria for classification of breast tissue by the FFOCM imaging technique.
There are also cases where invasive cancer and carcinoma in situ are simultaneously developed for a patient, as in the example of Fig. 26.5. Ductal carcinoma in situ is characterized by channels extended by the presence of cancer cells in the interior (D). Invasive ductal carcinoma components result in the presence of fine highly scattering fibrous tissue (B) and foci of darker grey carcinoma cells (D). Ductal in situ components are recognisable by enlarged ducts and lobules filled with cancer cells.
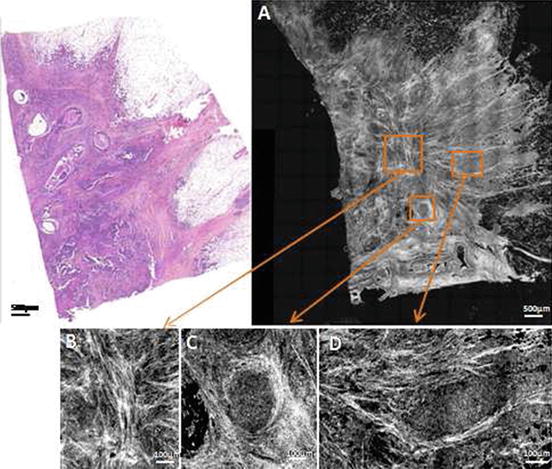
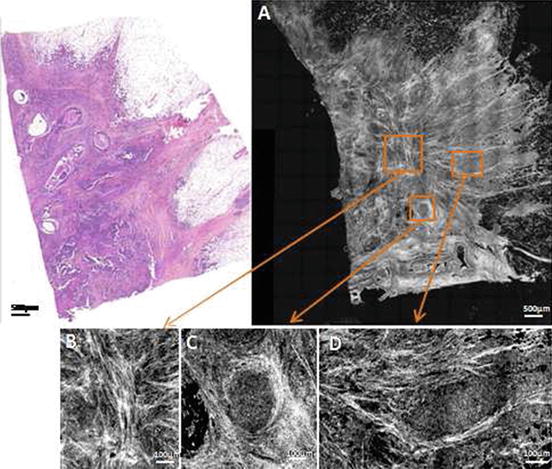
Fig. 26.5
Ductal adenocarcinoma with ductal in situ component: fibrous tissue finely spans highly diffusive (c), recognizable by its enlarged channel membrane elastic (c), fibrous tissue (white) surrounding cancer cells (dark gray) (d)
A fibroadenoma is represented in Fig. 26.6. This lesion is characterized by the presence of dilated ducts called tubular easily recognizable in the FFOCM image (B).
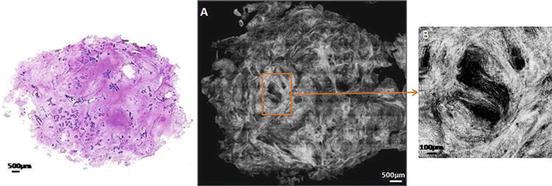
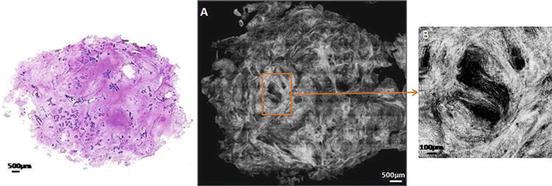
Fig. 26.6
Fibroadenoma (a) with characteristic enlarged channel (b)
Lobular carcinoma in situ is shown in Fig. 26.7, it is characterized by a proliferation of small cells widely dilated acini of lobules (C). This lesion is benign; however, in the example shown here, a milk duct (D) has at its center proliferation that is a sign of malignancy.
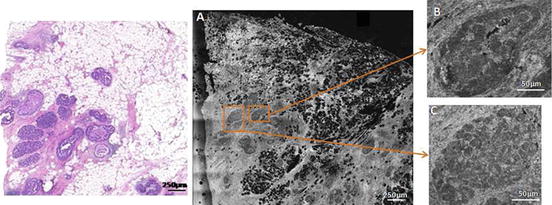
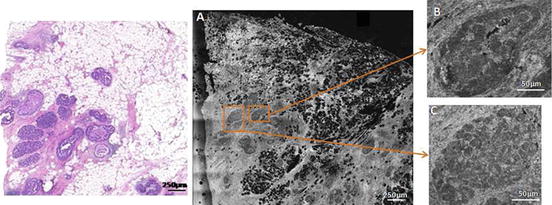
Fig. 26.7
Lobular carcinoma in situ (a) channel with expanded cellular proliferation in the center (b), with expanded lobule with visible acini (c)
Upon an analysis based on FFOCM images, it is possible to start a classification breast tissue. This approach is described in detail in [16].
26.1.5 FFOCM and Lymph Nodes
In this section, we intend to discuss the usefulness of FFOCM as a tool for classification of lymph nodes in order to assess whether this imaging technique could help surgical ablation of lymph more efficiently. Surgical treatment of breast malignancy is based on the removal of the primary tumor and the axillary dissection that involves the removal of more than half of the lymph nodes in the armpit on the same side. This process of removal of lymph is still considered as the standard even if it has several drawbacks. In fact, it often induces complications in the arm such as the decrease in the sensitivity of touch, pain in the upper arm on the operated side, a reduction in the function of the shoulder joint, or lymphedema of the arm in 80 % of cases [17]. In addition, it was shown for T1 tumors (tumor size less or equal to 2 cm) that the percentage of invaded axillary lymph nodes is 23 %, which means that 77 % of axillary dissection are unnecessary [18]. To limit the number of dissections, axillary sentinel node technique was introduced in 1998 [19]. The sentinel lymph node is a lymph node in the armpit. It is anatomically the closest to the breast tumor and, accordingly, will be the first node receiving lymphatic drainage from a tumor. If it is invaded, axillary dissection is performed; however if it appears not invaded, lymph nodes in the area are not removed.
The results of sentinel nodes analysis are used to determine the type of treatment to administer after surgery but are only available until several days after the operation, once achieving complete histology slides. It would be useful to the surgeon to have a quick picture of lymph to assess their invasion during the operation. It is in this spirit that FFOCM lymph nodes images have been made in order to estimate if this imaging technique is able to distinguish invaded lymph from healthy ganglions.
An initial study using the elastic scattering spectroscopy (ESS) showed the usefulness of intraoperative analysis lymph nodes [20], but this study provides only macroscopic information and not microscopic architectures. Normal breast node tissues are seen in Fig. 26.8: one can see dense fibrous tissue and spans (D) that appear white. Subcapsular sinus (C) stands below the capsule, while terminal centers (E) appear as areas gray rounded.
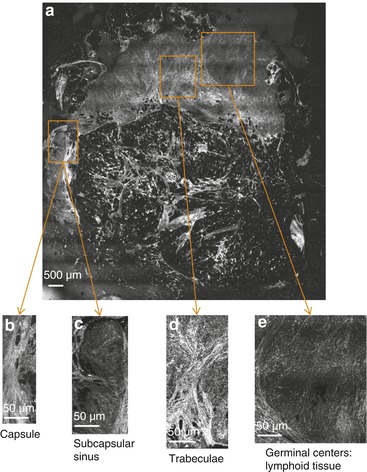
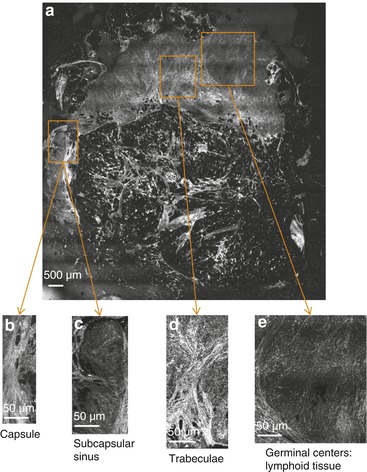
Fig. 26.8
Normal lymph node FFOCM images
The tumor lymph nodes are as recognizable in Fig. 26.9 as the structure of the ganglion is no longer respected and cell invasion within a zone lymphoid appears as light gray areas. These zones correspond to purple regions on the HES slice that are clusters of cancer cells (C bottom); healthy lymphoid appears very dark gray (C top). Also, by zooming in the FFOCM image, we note the presence of many vessels reflecting hypervascularity characteristic of the presence of metastases (A).
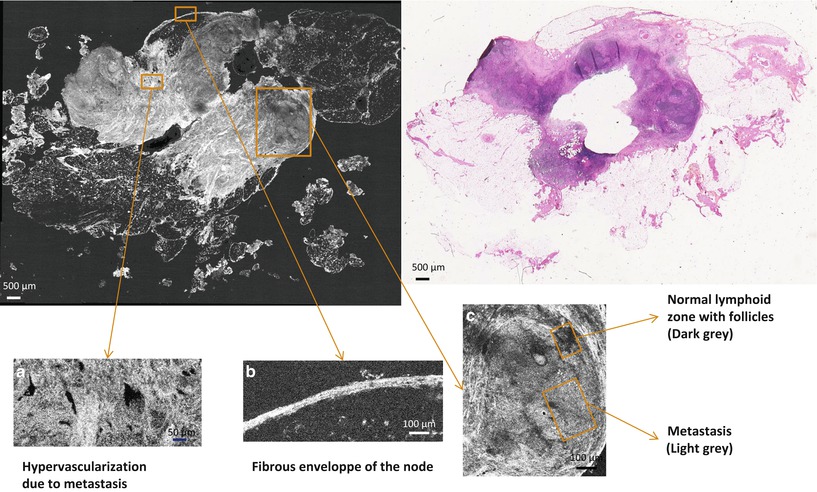
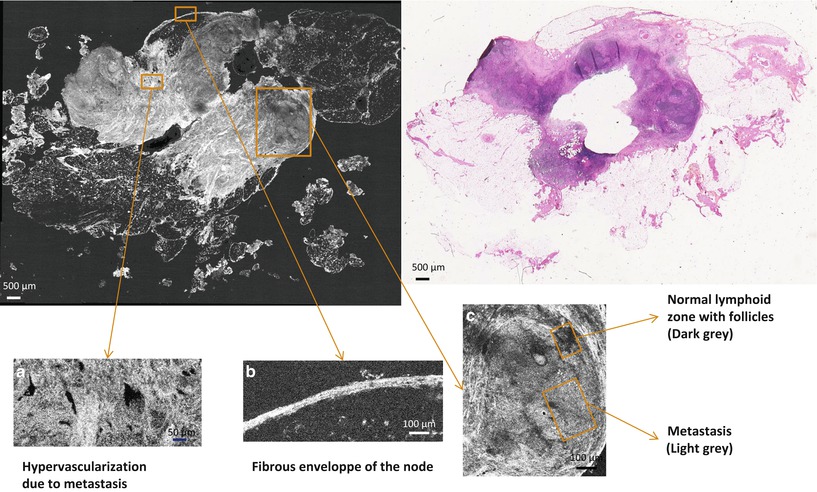
Fig. 26.9
Invaded lymph node FFOCM images
26.2 FFOCM Study of Brain Tissue
26.2.1 Specificity of Brain Tissue Surgery
The encouraging results on breast tissue prompted us to test full-field OCT diagnostic of the brain where resection is difficult and risky. In this section we offer a preliminary phase of preclinical evaluation with FFOCM as an intraoperative tool of brain tumor surgery. Despite significant progress in the diagnosis and treatment of tumors, the survival rate for malignant tumors of the central nervous system (CNS) remains low [21]. Awake surgery technique, which was developed in the 1990s by Professor Mitchell Berger at UCSF, has improved the quality of resection of tumors located near the functional areas of the brain. This surgery intends to avoid language disorders or sensorimotor functions penalties. However, when removing a tumor, the neurosurgeon should be able to identify the nature of the tissue that is removed. Current tools for the identification of tissue include intraoperative MRI, the use of a surgical microscope, the fluorescence-guided resection, and finally extemporaneous histological analysis.
Intraoperative MRI provides low-resolution images of the surgical field and complete section of the brain that are sufficient for a rough estimate of the abnormal tissue excised but offers low acquisition resolution (ranging typically between 0.5 and 1 mm) and artifacts occur at the air-tissue interface. Using an operating microscope illuminated by a xenon lamp gives a clear vision of the operative field but is limited by the low capacity of discrimination of white light. Fluorescence-guided resection allows a more accurate assessment of the tumor region with the specific binding of fluorophores on cancer cells and therefore allows a better selection of pathological tissues than the white-light operating microscope. However, this technique requires the absorption of an exogenous fluorophore by the patient before the operation and is only sensitive to solid tumors with a low sensitivity to detect infiltration of the brain parenchyma by cancer cells.
The results that we will show in this section are, to the best of our knowledge, the only large images with a resolution sufficient to distinguish microstructural cerebral parenchyma. The first published study using OCT for the study of brain tissue was performed with a surgical probe OCT system [22]. This in vivo system has axial and lateral resolutions limited to 16 and 30 μm, respectively, and is focused on a single sample of excised brain. Nevertheless, it was possible to recognize a melanoma and an artery. Later, SD-OCT systems have been favored for their better resolution (4/8 μm, transverse/axial) allowing distinguishing areas of solid tumors, areas invaded by the tumor and normal tissue [23] through the depth attenuation of signals. More recently, a study involving more patients with gliomas (9 in all) and using a TD-OCT system with 15 μm axial and transverse resolution was used to classify samples as malignant or benign with a good correlation with the histopathological diagnosis [24]. However, in this case too, the differentiation was based on the attenuation of the signal returned by the tumor zones relative to the healthy areas. The classification does not lead to the recognition of microscopic structures of the brain parenchyma in contrast to what we will show with FFOCM. A study published in 2005 showed images of brain microstructures using an ultrafast laser providing axial/lateral resolution of 1.3/3 μm, respectively. Thus, it was possible to differentiate malignant tissue from healthy tissue by the presence of blood vessels, microcalcifications, and cysts in the tumor tissue [25]. However, the images obtained are small (2 × 1 mm2), and they have only been performed on fixed tissue and require a femtosecond laser whose cost is high and size is rather large limiting its implementation under clinical conditions.
Our imaging technique presented here and described in Chap. 25, “Time Domain Full Field Optical Coherence Tomography Microscopy” combines several advantages; their large sizes are comparable to those of samples embedded in paraffin for histological analysis, thus facilitating images analysis by physicians. The imaging system is compact, can be placed in the operating room, and provides a sufficient resolution (1 μm axial and lateral) for distinguishing brain tissue microstructures.
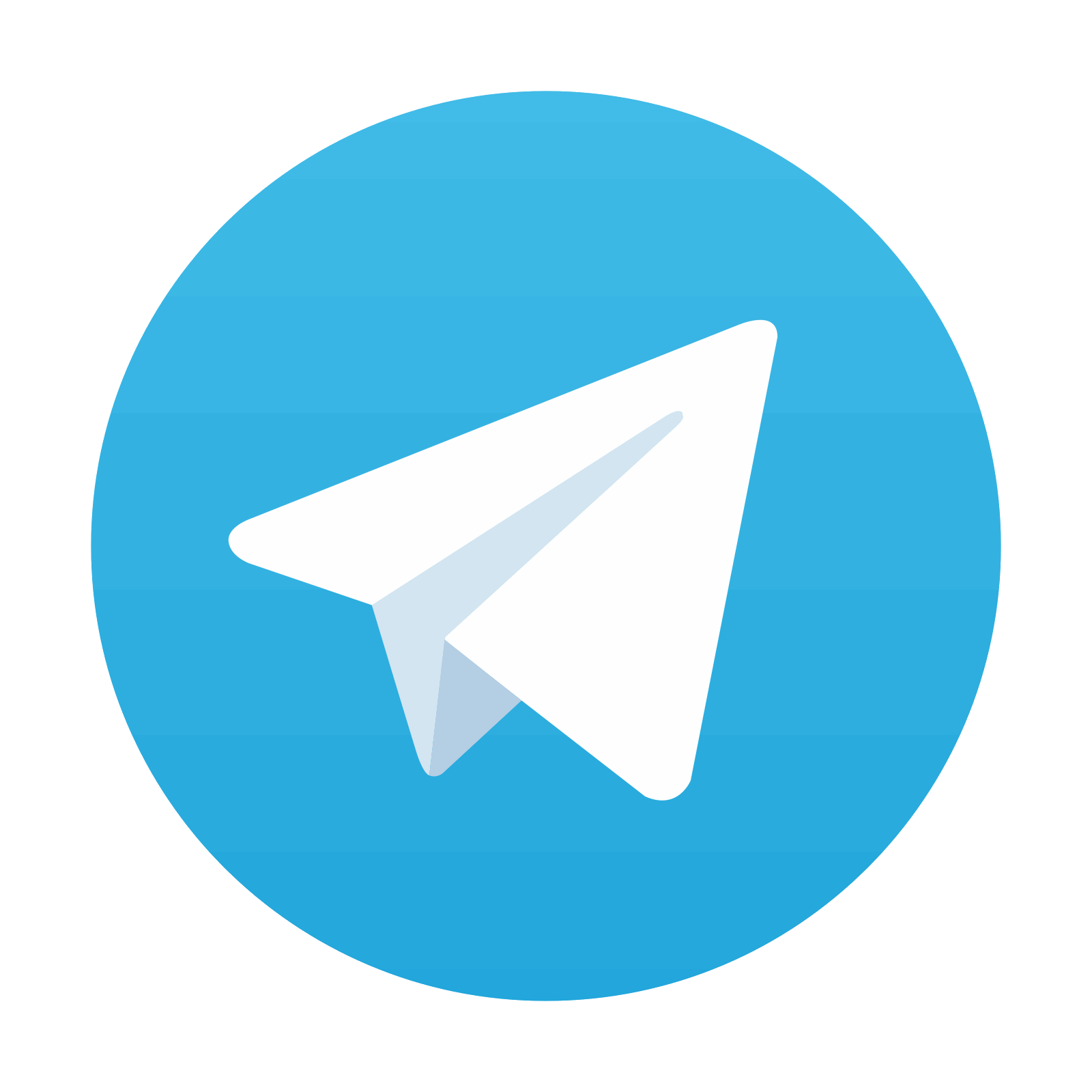
Stay updated, free articles. Join our Telegram channel

Full access? Get Clinical Tree
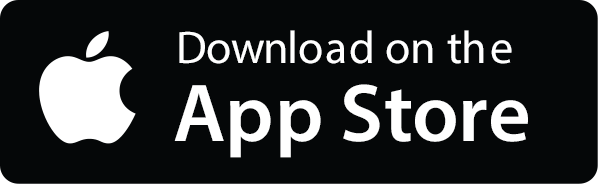
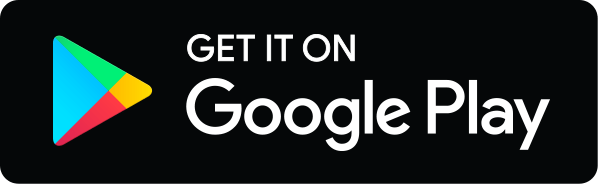