Antibiotic Therapy for Ocular Infection
Robert W. Snyder
D. Snow Slade
The appropriate use of antibiotics to treat ocular infection requires an understanding of the disease processes being treated and knowledge of the pharmacology and pharmacokinetics of the drugs used. The prompt use of the appropriate antibiotic is essential to minimize ocular damage and vision loss in bacterial keratitis or endophthalmitis. We will review antibiotic therapy for various ocular infections.
General Principles of Antibiotic Therapy
Antibiotic therapy may result in killing the microorganism (bactericidal drugs) or inhibiting bacterial growth (bacteriostatic drugs). When bacteriostatic drugs are used to treat ocular infection, the host defense mechanisms are ultimately responsible for clearing and eradicating the infective organism. In bacterial keratitis, infection develops in the avascular cornea, and in endophthalmitis it develops in the fluid-filled aqueous or vitreous cavity. In either case, the immune system may be unable to control the organism fast enough to prevent sight-threatening sequelae. Within the first 24 hours pathogens may multiply and release toxins and degradative enzymes that destroy the function and integrity of ocular tissues. Thus, bactericidal drugs are preferred for the treatment of severe ocular infection. The most desirable agents are those that can quickly eradicate organisms and/or halt the transcription, translation, or processing of degradative enzymes and toxins. The cephalosporins, aminoglycosides, and fluoroquinolones are bactericidal agents, and topical formulations of these antibiotics are commonly used to treat ocular infection. Of these, the quinolones are faster acting and may effectively block transcription and minimize the production of new mRNA of bacterial toxins and degradative enzymes.1 Tetracycline, erythromycin, chloramphenicol, and sulfonamide are bacteriostatic and not frequently used for severe infection. These antibiotics should be reserved for situations where there is a specific benefit, such as tetracycline in the treatment of ocular rosacea.
Antibiotics are not equal in their potency or ability to kill organisms. Since the introduction of the sulfonamides and penicillins, bacteria have developed mechanisms to render these antibiotics ineffective. These bacteria are said to develop resistance, and the particular antibiotic to which they have developed resistance is no longer capable of consistently eradicating the bacteria. Organisms can acquire resistance mechanisms that can be passed on in extrachromosomal elements, or resistance genes can become stable elements in the bacterial chromosome. The genes that confer resistance are often spread among bacteria of the same species and sometimes across species. As an antibiotic is used more frequently, chances increase that a resistance mechanism will arise through spontaneous mutation or selection that will result in a resistant strain of bacteria. In ophthalmology we have seen this with penicillin derivatives and first-generation cephalosporin antibiotics, where Gram-positive bacteria often develop resistance to these drugs. We also see an increasing tendency of Gram-negative organisms to develop resistance to the aminoglycosides. Local hospitals, regional microbiology centers, and the U.S. Centers for Disease Control continually monitor patterns of resistance.
In response to bacterial resistance, the pharmaceutical industry is continually searching for new drugs (or modifications of existing ones) to identify effective antibiotics. The most recent addition to the armamentarium for ophthalmic use has been the fluoroquinolone class of antibiotics. When the third-generation fluoroquinolones were first made available as ophthalmological preparations in the early 1990s, they were a major breakthrough since they were capable of eradicating both Gram-positive and Gram-negative organisms. This broad spectrum of activity allowed the use of a single drug to treat serious and sight-threatening infections such as bacterial keratitis. Prior to this, a combination of an aminoglycoside and cephalosporin was necessary to provide the range of coverage necessary to kill “all bacteria.” The third-generation fluoroquinolones include ciprofloxacin, ofloxacin, and levofloxacin. Unfortunately, in the 15 years since their introduction, there have emerged both Gram-positive and Gram-negative bacteria resistant to these drugs.2,3 Modifications to the third-generation quinolones have provided new fourth-generation fluoroquinolone antibiotics for ophthalmology, namely moxifloxacin and gatifloxacin. These fluoroquinolones are rapidly replacing their older, third-generation counterparts.4
Much of the antibiotic resistance occurs as the result of widespread systemic use of antimicrobial agents. In general, it is thought that with long-term, subtherapeutic antibiotic therapy there is a greater chance of bacterial strains developing resistance to the drug that is being used.1,4 In systemic use the number of organisms exposed to a given therapeutic regimen is vastly greater than ophthalmologists can expose with topically applied ophthalmic preparations; a single pill taken orally may expose 1012 organisms, whereas a topical eye drop may only expose 106. To date, there have been no studies documenting increased resistance of bacteria following the short-term use of prophylactic topical antibiotics. It may be that increased antibiotic resistance for a given drug is related to the number of organisms exposed to it, and thus systemic antibiotic therapy has a much greater risk to create resistant organisms.
Blondeau has introduced the concept of mutant prevention concentration. With respect to the quinolones he recommends practitioners use an antibiotic dose where the tissue concentration will result in the killing of all susceptible and low-level resistant organisms, thus preventing the selection of low-level resistant strains.5 When we consider topical antibiotic treatment, the antibiotic concentrations in the tear film are usually high enough to prevent resistance and are not sufficiently absorbed in the rest of the body to result in the selection of resistant organisms. However, there may be a risk of drug resistance to bacteriostatic agents when topical formulas of these agents are used for prolonged periods.
Penetration into the cornea is essential to treat bacterial keratitis and to prevent infection following laser-assisted in situ keratomileusis (LASIK) surgery. There is a great deal of interest in using topical antibiotics to help prevent postoperative endophthalmitis. To date however, there have been no clinical trials that demonstrate efficacy of topically applied antibiotics in preventing endophthalmitis. Nonetheless, there is interest in comparing antibiotic penetration of various drugs into the anterior chamber, and identifying dosing regimens that can result in therapeutic levels in the aqueous humor. The factors that influence the intraocular penetration of antibiotics include the charge of the drug, the status of the corneal epithelium, the degree of ocular inflammation, the formulation and concentration of the drug, and the dosage regimen employed.6 The chemical properties of a drug influence its intraocular penetration according to its ability to diffuse across the layers of the cornea. The outer epithelial layer is rich in lipids, whereas the inner stroma of the cornea is composed primarily of water (about 70%). Biphasic solubility is necessary for a molecule to diffuse across both the epithelium and the stroma. The pH and buffering system of an antibiotic’s formulation can profoundly influence its ocular penetration, as can the molecular size of the drug.7,8 Most ophthalmic antibiotics that are commonly used as topical agents are molecularly small enough to diffuse through biologic membranes without difficulty. Bacitracin, colistin, and other polymyxins however, are higher molecular weight antibiotics that penetrate the cornea poorly.7
Some drug formulations can cause microdisruption of the corneal epithelium, and this “microtoxicity” enhances drug penetration because the epithelium is the principal barrier to penetration. This is thought to occur when ethylenediaminetetra acetate (EDTA) or sodium benzoate are used as preservatives. Ocular inflammation may also result in damage to the corneal epithelial barrier and enhance penetration.6,7,8
Increasing the concentration of the antibiotic will increase the diffusion gradient across the cornea and enhance its penetration.7,9 This is evident with the new fluoroquinolones gatifloxacin 0.3% and moxifloxacin 0.5%. In penetration studies following topical dosage, the final concentration of moxifloxacin in the eye is higher based upon the higher initial concentration of the drug.10 The newer fluoroquinolones as a group are especially fast at killing bacteria and can reduce the colony count by 99.9% in 2 to 4 hours when the concentration is maintained at two times the minimum inhibitory concentration for 90% of the isolates (MIC90). The quinolones penetrate the cornea well, and concentrations in the cornea that are ten times higher than the MIC are easily attained.11
One may enhance drug tissue concentrations by using formulations that increase the persistence of the drug in the tear film. This can be accomplished by making the preparation more viscous or by placing it in an ointment form. In theory, ointments have superior bioavailability to solutions because of the increased contact time on the eye. Hydrophilic antibiotics however, may crystallize within the ointment base and prevent their release and subsequent penetration. The net effect is a decrease in their bioavailability.
Fortified preparations of aminoglycosides have been shown to be more effective than standard-strength solutions in killing microorganisms of experimental keratitis models.12,13,14,15 In the past, fortified antibiotics were frequently advocated to treat bacterial keratitis. Of the fluoroquinolones, only levofloxacin and moxifloxacin have been formulated in fortified antibiotic preparations.
Specific dosing techniques can also be used to advantage in topical therapy for serious ocular infections. A loading regimen of one drop of gentamicin every minute for 5 minutes, repeated every 2 to 4 hours, is capable of producing high concentrations of antibiotic within the cornea (above 125 μg per gram of cornea),16 while rehydrating a cornea after LASIK with ofloxacin has been shown to produce concentrations of approximately 40 μg per gram of cornea.17 When treating bacterial keratitis, experts in the prequinolone era recommended a sustained administration of an antibiotic—one drop of 0.3% gentamicin sulfate every 30 to 60 minutes.18,19 Combination aminoglycoside and cephalosporin drops were also used with a similar frequency. As can be imagined, this led to significant compliance problems, and patients were often hospitalized to assure adequate therapy. With the newer fluoroquinolones, however, there is faster killing and often lower MIC breakpoints, allowing less aggressive dosing, i.e., every hour while awake and then every 4 hours after bed, with a reduction of the dosing regimen after the first 48 hours. Many experts will use gatifloxacin 0.3% or moxifloxacin 0.5% for primary treatment of contact lens associated keratitis or other forms of bacterial keratitis. Vancomycin or a cephalosporin may be added as a second topical agent when patients present with severe central ulcers, or ulcers that threaten perforation. When more than one antibiotic is used, 5 minutes should elapse between installations to prevent a “washout” of the first antibiotic.
Toxicity of topically applied antimicrobials is another important issue. Toxicity may be related to the antibiotic itself, or to the preservatives and vehicles used in the formulation. Antibiotics may retard epithelial healing. This may occur to a greater extent with fortified solutions.19,20 Thimerosal, a mercurial compound with relatively weak antimicrobial activity, can cause hypersensitivity reactions, keratoconjunctivitis with coarse corneal epithelial changes, giant papillary conjunctivitis, corneal stromal infiltrates, and calcific band keratopathy.21,22,23,24,25 Benzalkonium chloride (BAK) is known to inhibit epithelial adhesion, cause a loss of superficial epithelial cells, and delay healing of the epithelium. These epithelial toxic effects and the surfactant properties of BAK may enhance the penetration of topically applied antibiotics.26,27 More recent formulation of gatifloxacin has reduced the BAK to 0.005%, and toxicity has not been seen for epithelial healing in a photorefractive keratectomy model when compared to the nonpreserved moxifloxacin.28 Since the BAK itself is bactericidal, the addition of BAK to the formulation can significantly enhance the killing rate for topical gatifloxacin 0.3% (Zymar; Allergan Pharmaceutical Corporation)29; theoretically, the BAK facilitates faster killing for sight-threatening infections.
The use of topical, preoperative antibiotics as prophylaxis against endophthalmitis remains controversial.30,31,32,33,34 There are no prospective studies to substantiate their usefulness.35 Evidence to support their use postoperatively is also sparse. A study conducted by Christy and Sommer found benefit from subconjunctival plus topical antibiotic.36 On rare occasions, severe retinal toxic reactions have occurred with the postoperative administration of subconjunctival aminoglycosides, and cephalosporin administration may by preferred. Most of these reactions have been associated with inadvertent scleral perforation during the drug administration.37 Visualization of the needle tip during administration will reduce this risk.
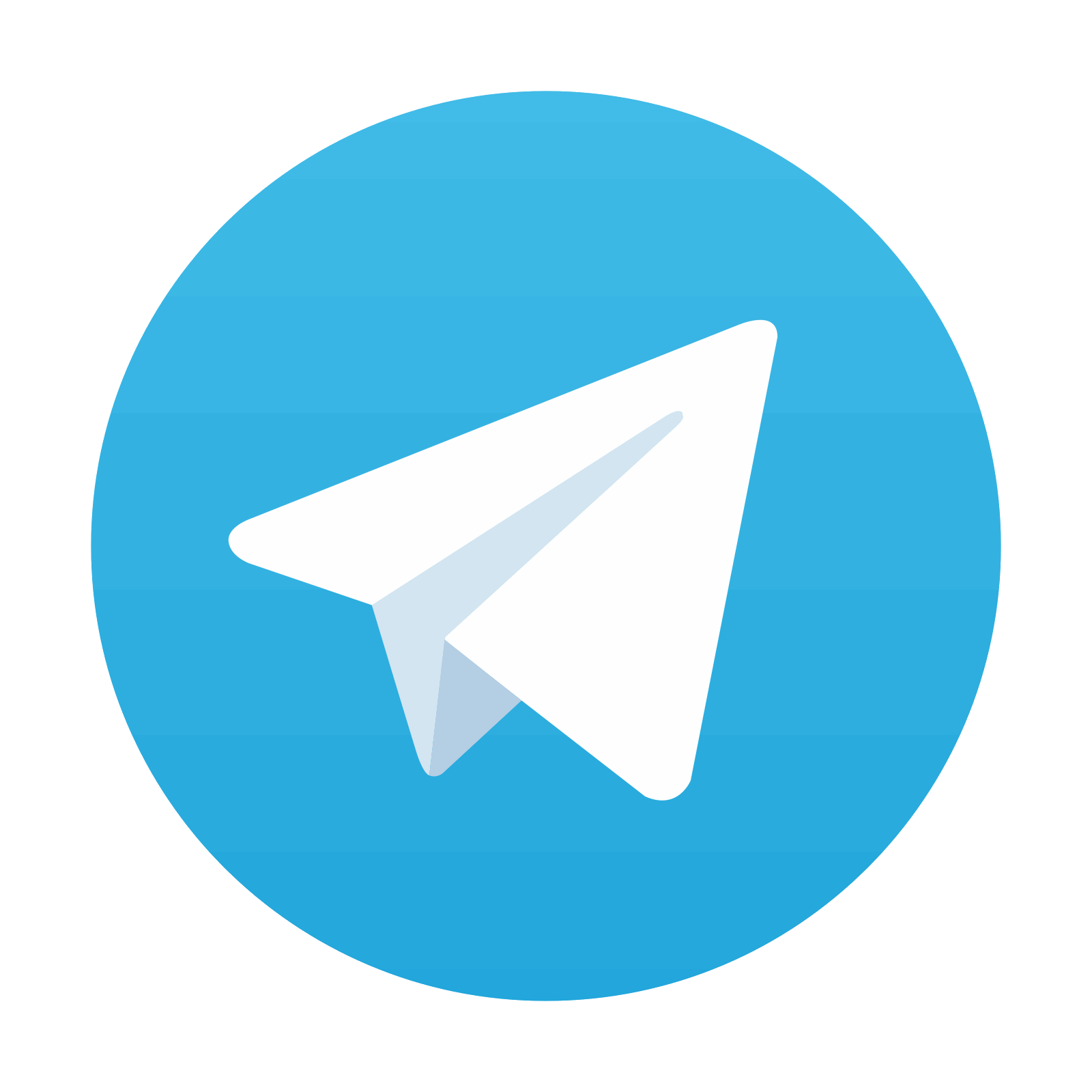
Stay updated, free articles. Join our Telegram channel

Full access? Get Clinical Tree
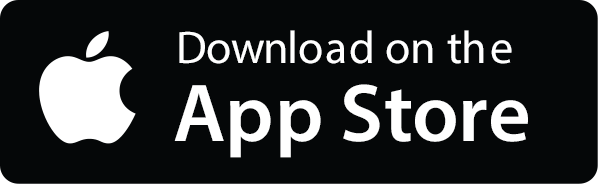
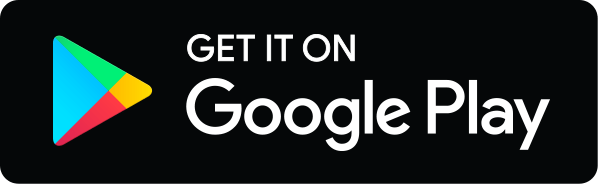