2 Anatomy and Physiology of the Auditory System Hearing and its disorders are intimately intertwined with the anatomy and physiology of the auditory system, which is composed of the ear and its associated neurological pathways. The auditory system is fascinating, but learning about it for the first time means that we must face many new terms, relationships, and concepts. For this reason it is best to begin with a general bird’s-eye view of how the ear is set up and how a sound is converted from vibrations in the air to a signal that can be interpreted by the brain. A set of self-explanatory drawings illustrating commonly used anatomical orientations and directions is provided in Fig. 2.1 for ready reference. Fig. 2.2 shows how the structures of the hearing system are oriented within the head. The major parts of the ear are shown in Fig. 2.3. One cannot help but notice that the externally visible auricle, or pinna, and the ear canal (external auditory meatus) ending at the eardrum (tympanic membrane) make up only a small part of the overall auditory system. This system is divided into several main sections: The outer ear includes the auricle and ear canal. The air-filled cavity behind the eardrum is called the middle ear, also known as the tympanic cavity. Notice that the middle ear connects to the pharynx by the Eustachian tube. Medial to the middle ear is the inner ear. Three tiny bones (malleus, incus, and stapes), known as the ossicular chain, act as a bridge from the eardrum to the oval window, which is the entrance to the inner ear. The inner ear contains the sensory organs of hearing and balance. Our main interest is with the structures and functions of the hearing mechanism. Structurally, the inner ear is composed of the vestibule, which lies on the medial side of the oval window; the snail-shaped cochlea anteriorly; and the three semicircular canals posteriorly. The entire system may be envisioned as a complex configuration of fluid-filled tunnels or ducts in the temporal bone, which is descriptively called the labyrinth. The labyrinth, which courses through the temporal bone, contains a continuous membranous duct within it, so that the overall system is arranged as a duct within a duct. The outer duct contains one kind of fluid (perilymph) and the inner duct contains another kind of fluid (endolymph). The part of the inner ear concerned with hearing is the cochlea. It contains the organ of Corti, which in turn has hair cells that are the actual sensory receptors for hearing. The balance (vestibular) system is composed of the semicircular canals and two structures contained within the vestibule, called the utricle and saccule. The sensory receptor cells are in contact with nerve cells (neurons) that make up the eighth cranial (vestibuloacoustic) nerve, which connects the peripheral ear to the central nervous system. The auditory branch of the eighth nerve is often called the auditory or cochlear nerve, and the vestibular branches are frequently referred to as the vestibular nerve. The eighth nerve leaves the inner ear through an opening on the medial side of the temporal bone called the internal auditory meatus (canal), and then enters the brainstem. Here, the auditory portions of the nerve go to the cochlear nuclei and the vestibular parts of the nerve go to the vestibular nuclei. The hearing process involves the following series of events. Sounds entering the ear set the tympanic membrane into vibration. These vibrations are conveyed by the ossicular chain to the oval window. Here, the vibratory motion of the ossicles is transmitted to the fluids of the cochlea, which in turn stimulate the sensory receptor (hair) cells of the organ of Corti. When the hair cells respond, they activate the neurons of the auditory nerve. The signal is now in the form of a neural code that can be processed by the nervous system. The outer ear and middle ear are collectively called the conductive system because their most apparent function is to bring (conduct) the sound signal from the air to the inner ear. The cochlea and eighth cranial nerve compose the sensorineural system, so named because it involves the physiological response to the stimulus, activation of the associated nerve cells, and the encoding of the sensory response into a neural signal. The aspects of the central nervous system that deal with this neurally encoded message are generally called the central auditory nervous system. To be meaningful, a study of the ear must begin with a study of the temporal bone. Most of the structures that make up the ear are contained within the temporal bone (Fig. 2.2). In fact, the walls of these structures and all of the bony aspects of the ear, except for the ossicles, are actually parts of the temporal bone itself. Recall from your anatomy class that the skeleton of the head is composed of 8 cranial bones and 14 facial bones. The right and left temporal bones compose the inferior lateral aspects of the cranium. Beginning posteriorly and moving clockwise, the temporal bone articulates with the occipital bone behind, the parietal bone behind and above, the sphenoid and zygomatic bones to the front, and the mandible anteriorly below. All of these connections, except for the articulation with the mandible, are firmly united, seam-like fibrous junctions called sutures. The articulation with the mandible is via the highly mobile temporomandibular joint. Lateral and medial views of the temporal bone are shown in Fig. 2.4. The lateral surface of the bone faces the outside of the head and the medial surface faces the inside of the head. The temporal bone is composed of five sections, including the mastoid, petrous, squamous, and tympanic parts, and the styloid process. Fig. 2.4 (a) Lateral and (b) medial views of the right temporal bone. (Adapted from Proctor [1989], with permission.) The squamous part is a very thin, fan-shaped portion on the lateral aspect of the bone. It articulates with the parietal bone posteriorly and superiorly, and with the sphenoid bone anteriorly. The prominent zygomatic process runs anteriorly to join with the zygomatic bone, forming the zygomatic arch. Just below the base of the zygomatic process is a depression called the mandibular fossa, which accepts the condyle of the mandible to form the temporomandibular joint just anterior to the ear canal. The petrous part is pyramid-shaped and medially oriented so that it forms part of the base of the cranium. This extremely hard bone contains the inner ear and the internal auditory meatus through which the eighth cranial nerve travels on its way to the brainstem, so that much of the discussion pertaining to the inner ear is also a discussion of this part of the temporal bone. The tympanic part is inferior to the squamous and petrous parts and anterior to the mastoid. The tympanic part forms the inferior and anterior walls of the ear canal, as well as part of its posterior wall. The styloid process is an anteroinferior pillar-like projection from the base of the temporal bone that varies widely in size. It does not contribute to the auditory structures but is of interest to us as the origin of several muscles involved in the speech mechanism. The outer ear is composed of the pinna and the ear canal, ending at the eardrum. The tympanic membrane is generally considered to be part of the middle ear system, which includes the middle ear cavity and its contents, and “ends” where the ossicles transmit the signal to the inner ear fluids at the oval window. The externally visible aspect of the ear is an oddshaped appendage called the pinna or auricle. The internal structure of the pinna is composed principally of elastic cartilage (except for the earlobe). It also contains some undifferentiated intrinsic muscle tissue, as well as several extrinsic muscles, although these are vestigial structures in humans. However, these muscles are not vestigial in many lower animals that are able to orient their pinnae with respect to the location of a sound source. The major landmarks of the pinna are highlighted in Fig. 2.5. Notice that the pinna is not symmetrical. For example, and most obviously, it has a flap-like extension that angles away from the skull in the backward direction, so that the pinna overlaps the side of the head posteriorly, superiorly, and inferiorly, but not anteriorly. It also has an intricate arrangement of ridges, bumps, and furrows. The entrance to the ear canal is at the bottom of a large, cup-shaped depression called the concha, and is partially covered by a posteriorly directed projection or ridge called the tragus. The ridged rim along most of the perimeter of the pinna is the helix. Starting at the very top of the pinna, the helix courses anteriorly and downward, making a hook-like turn in the posterior direction to form a shelf above the concha, which is the crus of the helix. Again, beginning at the top of the pinna, the helix courses downward along the posterior perimeter, reaching the earlobe, or lobule, at the bottom. The scaphoid fossa is the furrow just anterior to the helix as it courses down the posterior rim of the pinna. The ridge anterior to the scaphoid fossa is called the antihelix. The antihelix runs parallel with the helix and splits superiorly into the two crura of the antihelix. The splitting of the antihelix into two crura creates a triangle-shaped depression called the triangular fossa. If we follow the antihelix downward, it widens at the bottom to form the anti-tragus, an upward pointing mound that is located posteroinferior to the tragus and superior to the earlobe. The space or angle between the tragus and the antitragus is the intertragic incisure. The ear canal is more formally called the external auditory meatus (canal). On average, it is ~ 9 mm high by 6.5 mm wide, and is roughly 2.5 cm to 3.5 cm long. The ear canal is not quite a straight tube, but has two curves forming a slightly S-like pathway. These curves usually make it difficult to get an unobstructed view of the eardrum, so that it is usually necessary to straighten the canal before looking inside with an otoscope. An otoscope is the familiar instrument used for examining the ear (Fig. 2.6). The ear canal is straightened by gently pulling up and back on the pinna, as illustrated in Fig. 2.7. The external auditory meatus is lined with tight-fitting skin. However, the outer third of the canal is different from the inner two thirds in several ways. The underlying material is cartilage in the outer third and bone for the remainder of its length. The bony portion of the canal is derived from (1) the tympanic part of the temporal bone, which forms the floor and anterior wall, as well as the inferoposterior wall; (2) the squamous part, making up the roof and part of the posterior wall; and (3) the condyle of mandible, which contributes to the inferioanterior wall at the temporomandibular joint. The cartilaginous portion contains hairs as well as a plentiful distribution of sebaceous (oil) and ceruminous (wax) glands, although this is not the case for the bony portion of the canal. Sebaceous glands are also present in the concha. Their secretions serve lubricating and antimicrobial functions, and also help to keep the canal free of debris and even some foreign bodies and insects. The external auditory meatus ends at the tympanic membrane or eardrum, which is tilted at an angle of ~ 55° to the canal. The tympanic membrane is firmly attached to the tympanic sulcus, a groove in the bony canal wall, by a ring of fibrocartilaginous connective tissue called the tympanic annulus (or annular ligament). The ring has a deficiency at the top due to a tiny interruption in the tympanic sulcus known as the notch of Rivinus. The eardrum is a smooth, translucent, and sometimes almost transparent membrane with an average thickness of only ~ 0.074 mm. It is slightly taller (~ 0.9 to 1.0 cm) than it is wide (~ 0.8 to 0.9 cm), and it is concave outward rather than flat. The peak of the cone-like inward displacement is called the umbo. Structurally, the eardrum is often described as having three layers, although more correctly there are four of them. The most lateral layer of the tympanic membrane is continuous with the skin of the ear canal, and the most medial layer is continuous with the mucous membrane of the middle ear. Sandwiched between them are two fibrous layers. One of them is composed of radial fibers reminiscent of the spokes of a wheel, and the other layer is made of essentially concentric circular fibers. Fig. 2.6 Examples of otoscopes used for examining the ear, along with reusable and disposable specula. The speculum is the cone-shaped attachment that is inserted into the ear canal. Fig. 2.7 The ear canal is straightened to facilitate otoscopic inspection by gently pulling up and back on the pinna. The tympanic membrane is connected to the malleus, which is the first of the three middle ear bones. Specifically, a long, lateral process of the malleus called the manubrium attaches almost vertically to the eardrum, with its tip at the umbo and continuing upward toward the position of 1 o’clock in the right ear and 11 o’clock in the left ear. This attachment of the manubrium of the malleus forms the malleal prominence. Ligamentous bands called the anterior and posterior malleal folds run from both sides of the malleal prominence to the notch of Rivinus, forming a triangular area between them on the eardrum. The largest part of the tympanic membrane lies outside or below the malleal folds, and is called the pars tensa (“tense part”) because it contains all three layers described above. The superior area of the eardrum between the malleal folds is missing the fibrous layers, and is called the pars flaccida (“flaccid area”) for this reason. It is also known as Shrapnell’s membrane. Fig. 2.8 shows some of the major landmarks that are identified when looking at the eardrum through an otoscope. In addition to the landmarks already mentioned, notice that the light from the otoscope is reflected back in a characteristic way, called the cone of light or light reflex. This reflection is seen as a bright area on the anteroinferior surface of the eardrum, radiating from the tip of the manubrium to the 5 o’clock position in the right ear and the 7 o’clock position in the left ear. It is often possible to identify one or more middle ear landmarks, especially when viewing relatively transparent eardrums. The cavity in the temporal bone behind the tympanic membrane is called the middle ear, tympanum, or tympanic cavity. The posterosuperior portion of the middle ear space is usually viewed as an “attic room” above the main tympanic cavity, and is called the epitympanic recess or the attic. This space accommodates the more massive portions of the two larger ossicles, the incus and the malleus. Using a certain amount of artistic license, Fig. 2.9 depicts the middle ear space as a box-shaped room. Keep in mind that the box is only an analogy used to describe relative directions and relationships. In addition, Fig. 2.10 shows a more realistic drawing of the tympanic cavity and its contents looking backward toward its posterior wall. The tympanic membrane forms the lateral wall, and is shown folded downward to reveal the inside of the “room” in the figure. The middle ear really has irregularly shaped curved surfaces as in the other figures, not flat walls with right-angled corners. The floor of the tympanic cavity separates it from the jugular bulb below. The ceiling is the tegmen tympani, which is the thin bony plate that separates the tympanic cavity from the brain cavity above. Low down on the anterior wall (~ 3 mm up from the floor) is the opening of the Eustachian tube (sometimes called the auditory tube). The internal carotid artery canal is located on the other side of (i.e., anterior to) the anterior wall, just below the Eustachian tube. Just above the Eustachian tube is the tensor tympani semicanal, which contains the tensor tympani muscle. The tensor tympani semi-canal and Eustachian tube are separated by a bony shelf or septum. There is a curved bony projection on the anterior/medial wall that points into the middle ear space, called the cochleariform process. The tendon of the tensor tympani muscle bends around the cochleariform process and proceeds in the lateral direction on its way to the malleus (Fig. 2.10). The prominent bulge on the medial wall is the promontory of the basal turn of the cochlea. The oval window of the cochlea (with its attachment to the stapes) is located posterosuperior to the promontory, and the round window of the cochlea is posteroinferior to it. The facial nerve canal prominence is situated superior to the oval window. The posterior wall separates the tympanic cavity from the mastoid. The aditus ad antrum is an opening located superiorly on the rear wall, and provides communication between the epitympanic recess of the middle ear cavity and the antrum of the mastoid air cell system. The pyramidal eminence or pyramid is a prominence on the posterior wall that contains the body of the stapedius muscle. The stapedial tendon exits from the apex of the pyramid and proceeds to the stapes. The fossa incudis is a recess on the posterior wall that accommodates the short process of the incus. The chord tympani nerve is a branch of the facial (seventh cranial) nerve that enters the middle ear from an opening laterally at the juncture of the posterior and lateral walls, runs just under the neck of the malleus, and leaves the middle ear cavity via the opening of the anterior chordal canal (of Huguier) that is anterior to the tympanic sulcus. Fig. 2.9 An artist’s conceptualization of the middle ear cavity as a room, with the lateral wall (including the eardrum and attached malleus) folded down to reveal the inside. The stapes is shown in place in the oval window. (Adapted from Proctor [1989], with permission.) Fig. 2.10 Artist’s representation of the middle ear looking backward toward the posterior wall (m., muscle; n., nerve). (Adapted from Schuenke M, Schulte E, Schumacher U, Ross LM, Lamperti ED, Voll M. 2010. Head and Neuroanatomy [Thieme Atlas of Anatomy]. New York, NY: Thieme; Fig. 9.3b, p. 144, with permission.) Three tiny bones known as the ossicles or the ossicular chain transmit the sound-induced vibrations of the tympanic membrane to the cochlea via the oval window (Fig. 2.11). The ossicles are the smallest bones in the body, and include the malleus, incus, and stapes. Instead of being attached to other bones, the ossicular chain is suspended within the middle ear cavity by ligaments and tendons, as well as its attachments to the tympanic membrane and the oval window. The malleus (“hammer” or “mace”) is ~ 8 to 9 mm long and weighs ~ 25 mg. Its manubrium (handle) is firmly embedded between the fibrous and mucous membrane layers of the eardrum, forming the lateral attachment of the ossicular chain. The neck of the malleus is a narrowing between its manubrium and head. Its lateral process produces a bulge on the eardrum that is often visible otoscopically. There is also an anterior process near the junction of the neck and manubrium. The head of the malleus connects with the body of the incus by a diarthrodial or double-saddle joint, the incudomallear articulation, such that these two bones move as a unit. Fig. 2.11 The ossicular chain in place within the middle ear. (Adapted from Tos [1995], with permission.) The incus, which is ~ 7 mm long and weighs roughly 30 mg, is commonly called the “anvil” but looks more like a tooth with two roots. The short process is posteriorly oriented and is accommodated by the fossa incudus on the back wall of the middle ear. The long process descends from the body of the incus, parallel with the manubrium of the malleus, and then hooks medially to end at a rounded nodule called the lenticular process. In turn, the lenticular process articulates with the head of the stapes via a true ball-and-socket or enarthrodial joint called the incudostapedial joint. The stapes bears a close resemblance to a “stirrup,” which is its common name. Its head is connected via the neck to two strut-like processes called the anterior and posterior crura, which lead down to the oval shaped footplate. The opening between the crura and footplate is called the obturator foramen. The stapes weighs only 3 to 4 mg. It is ~ 3.5 mm long, and the footplate has an area of roughly 3.2 mm2. The footplate is attached to the oval window by the annular ligament, forming the medial attachment of the ossicular chain. In addition to its lateral attachment to the tympanic membrane and its medial attachment at the oval window via the annular ligament, the ossicular chain is also supported by several ligaments and the tendons of the two middle ear muscles. The superior malleal ligament runs from the roof (tegmen tympani) of the attic down to the head of the malleus. The anterior malleal ligament goes from the anterior tympanic wall to the anterior process of the malleus. The lateral malleal ligament extends from the bony margin of the notch of Rivinus to the neck of the malleus. The posterior incudal ligament (actually a fold of mucous membrane rather than a ligament) runs from the fossa incudus to the short process of the incus. The tensor tympani muscle is innervated by the trigeminal (fifth cranial) nerve. It is housed within the tensor tympani semicanal in the anterior middle ear wall superior to the Eustachian tube. This muscle is ~ 25 mm long and arises from the cartilage of the Eustachian tube, the walls of its semicanal, and the segment of sphenoid bone adjacent to the canal. The tensor tympani tendon bends around the cochleariform process and proceeds in the lateral direction to insert on the malleus at the top of the manubrium near the neck. Contraction of the tensor tympani pulls the malleus in the anteromedial direction, thereby stiffening the ossicular chain. The stapedius muscle is the smallest skeletal muscle in the body, with an average length of only 6.3 mm. It is contained within the pyramidal eminence of the posterior wall of the tympanic cavity, and is innervated by the facial (seventh cranial) nerve. The stapedius tendon exists via the apex of the pyramidal eminence and runs anteriorly to insert on the posterior aspect of the neck of the stapes. Contraction of the stapedius pulls the stapes posteriorly. Even though the middle ear muscles pull in more or less opposite directions, they both exert forces that are perpendicular to the normal motion of the ossicles, and their contraction has the effect of stiffening the ossicular chain, thereby reducing the amount of energy that is delivered to the inner ear. The acoustic reflex refers to the reflexive middle ear muscle contraction that occurs in response to high levels of sound stimulation (Gelfand 1984, 2010). In humans this is at least principally a stapedius reflex, while the tensor tympani contracts as part of a startle reaction to very intense sounds, and also in response to certain kinds of nonacoustic stimulation such as an air jet directed at the eye. The acoustic reflex involves both muscles in some animals. The fundamental aspects of the acoustic (stapedius) reflex arc are as follows (Borg 1973; Wiley & Block 1984). The sensory (afferent) pathway proceeds via the auditory nerve to the ventral cochlear nucleus, and then to the superior olivary complex on both sides of the brainstem (with the crossover to the opposite side by way of the trapezoid body). The motor (or efferent) pathway is followed bilaterally, and runs from the motor nuclei of the facial (seventh cranial) nerve on both sides, and then via the facial nerves to the stapedius muscles. The motor pathway for tensor tympani activation goes from the trigeminal (fifth cranial) nerve nuclei to the tensor tympani muscles via the trigeminal nerves. Because contraction of the stapedius and tensor tympani muscles stiffens the middle ear system, the transmission of low frequencies is reduced (Simmons 1959; Møller 1965; Rabinowitz 1976). This change has been observed as a decrease in hearing sensitivity or loudness for low-frequency sounds (Smith 1943; Reger 1960; Morgan & Dirks 1975), although these effects are not always found (Morgan, Dirks, & Kamm 1978). The purpose of the acoustic reflex is not really known, although several theories have been proposed. The protection theory suggests the acoustic reflex protects the inner ear from potentially damaging sound levels. However, it is unlikely that this is its main purpose because the reflex has a delay (latency) that would make it an ineffective protection against sudden sounds, and the contraction adapts over time, limiting its protection against ongoing sounds. The fixation theory holds that the middle ear muscles maintain the appropriate positioning and rigidity of ossicles, and the accommodation theory states that the muscles modify the characteristics of the conductive system so that the absorption of sound energy is maximized. Simmons (1964) suggested the acoustic reflex improves the audibility of environmental sounds by attenuating internal sounds. This enhancement of signal-to-noise ratio would improve the survival rates for both the fox who is hunting for dinner and the rabbit who is trying to avoid being the main course. Similarly, Borg (1976) proposed that one of the purposes of the reflex is to improve the listener’s dynamic range by attenuating low frequency sounds. Discussions of these and other reflex theories may be found in several sources (Jepsen 1963; Simmons 1964; Borg, Counter, & Rosler 1984). The Eustachian (auditory) tube provides for the aeration and drainage of the middle ear system, and makes it possible for air pressure to be the same on both sides of the eardrum. It runs from the anterior middle ear wall to the posterior wall of the nasopharynx behind the inferior nasal turbinate, as illustrated in Fig. 2.12 and Fig. 2.13. In adults the Eustachian tube follows a 3.5 to 3.8 cm long course that is directed inferiorly, medially, and anteriorly, tilting downward at an angle of ~ 45°. However, it is important to be aware that the Eustachian tube is almost horizontal in infants and young children (see Fig. 6.4 in Chapter 6). The first third of the tube beginning at the middle ear is surrounded by bone and the remainder is surrounded by an incomplete ring of elastic cartilage. The meeting point of the bony and cartilaginous portions is called the isthmus. The lumen of the Eustachian tube is narrowest at the isthmus, where it is only 1 to 2 mm across compared with 3 to 6 mm in the remainder of the tube. A prominence on the pharyngeal wall, the torus tubarius, is formed by the cartilage of the Eustachian tube and other tissues (e.g., the salpingopalatine, salpingopharyngeous, and tensor palatini muscles). Fig. 2.12 The Eustachian tube in relation to the ear. Note that the bony portion of the tube meets the cartilaginous portion at the isthmus. (Adapted from Hughes [1985], with permission.) The cartilaginous portion of the Eustachian tube is arranged as shown in Fig. 2.14. Notice that the cartilage hooks around the tube from above, forming the incomplete ring alluded to above. A portion of the tensor palatini muscle is attached to the hooked segment of the cartilage (Fig. 2.13 and Fig. 2.14). At rest, the cartilage keeps the Eustachian tube closed (Fig. 2.14a). The lumen of the tube is opened when the cartilage is uncurled due to the pull exerted by the tensor palatini muscle (Fig. 2.14b). This occurs during swallowing, yawning, and other actions that cause the tensor palatini muscle to contract. Negative pressure develops in the middle ear (compared with the outside, atmospheric pressure) when this mechanism fails to open the Eustachian tube frequently and effectively. We have all experienced this phenomenon as fullness in the ears that is (hopefully) alleviated when the tube “pops open” due to a swallow, yawn, or some other maneuver. Swelling and/or blockage by mucus due to colds or allergy, and obstruction of the pharyngeal orifice by hypertrophic (enlarged) adenoids, are just a few of the causes of a nonpatent Eustachian tube. The resulting negative pressure within the closed-off middle ear space often precipitates the development of clinically significant middle ear disease. Fig. 2.13 Relationship of the Eustachian tube to the tensor palatini muscle, highlighting the parts of the muscle that arise from the tubal cartilage, hook around the pterygoid hamulus of the sphenoid bone, and insert into the palate. (Adapted from Hughes [1985], with permission.) Fig. 2.14 (a) The hook-shaped arrangement of the Eustachian tube cartilage keeps the tube in the normally closed state. (b) The tube is opened when the cartilage hook is uncurled by action of the tensor palatini muscle. Sound entering the outer ear is picked up by the tympanic membrane. The vibrations of the eardrum are transmitted to the ossicular chain, which vibrates essentially in the right-left plane. This vibration is represented as a rocking motion of the stapes footplate in the oval window, as shown in Fig. 2.15. The resulting inward and outward displacements of the oval window are thus transmitted to the cochlear fluid. However, instead of just serving as an inert passageway that allows sound to travel to the cochlea from the surrounding air, the conductive mechanism actually modifies sound in several ways that have a direct bearing on how and what we hear. Sounds reaching the eardrum are affected by the acoustics of the external auditory meatus, which operates as a quarter-wavelength resonator because it is essentially a tube with one open end and one closed end (at the tympanic membrane). Sounds entering the ear will be enhanced if they are close to the resonant frequency range, resulting in a boost in the sound pressure level (SPL) reaching the eardrum, called the ear canal resonance effect. To determine this boost in level (or gain) at the eardrum, the sound presented from a loudspeaker is monitored by a microphone outside the patient’s ear and also by a special kind of microphone that monitors the sound level inside the subject’s ear canal, very close to the eardrum. The difference between these two measurements is the amount of gain provided by the ear canal resonance, shown as a function of frequency in Fig. 2.16. On this kind of graph, 0 dB means “unchanged,” that is, that the SPL at the eardrum is the same as it is outside the person’s ear. Positive values show the amounts of gain provided by the ear canal resonances (negative values mean that the level is lower at the eardrum than outside the ear). We see clearly that the resonance characteristics of the ear canal provide a sound level boost of as much as 15 to 20 dB in the frequency range from roughly 2000 to 5000 Hz. In addition, the middle ear has a resonant region between ~ 800 Hz and ~ 5000–6000 Hz (Zwislocki 1975). The resonant responses of the conductive system affect our hearing sensitivity for sounds at different frequencies, as discussed in Chapter 3. Fig. 2.15 The middle ear transmits the signal to the cochlea via a rocking motion of the stapedial footplate at the oval window. (After Bekesy [1941].) The graph in Fig. 2.16 is technically called the head-related transfer function (HRTF) because it shows how the sound reaching the eardrum is affected by the direction of the sound source relative to the head. In other words, the HRTF shows how the spectrum is changed by the acoustical path from the loudspeaker to the eardrum. Thus, it is sometimes also called the sound field to eardrum transfer function. The HRTF actually reflects the accumulated effect of all factors that influence the sound on the way from the loudspeaker to the tympanic membrane, including acoustical shadows, reflections, and diffraction due to the head and body, as well as the ear canal resonance. This is why the figure caption specifies the direction of the loudspeaker. These acoustical effects depend on the direction of the sound and are important cues for directional hearing, or the ability to identify the location of a sound source and differences in locations between different sound sources. Fig. 2.16 Average head-related transfer functions (sound level at the eardrum compared with outside of the ear) for sounds presented from a loudspeaker directly in front of the subjects. (Dotted line, Wiener & Ross [1946]; dashed line, Shaw [1974]; solid line, Mehrgardt & Mellert [1977].) (From Mehrgardt & Mellert [1977], with permission of the Journal of the Acoustical Society of America.) Sound direction is described in terms of angles around the head (Fig. 2.17). The easiest way to describe the location of a sound source is to give its angle relative to the head (e.g., so many degrees to the right, so many degrees straight up from the front, etc.). The horizontal plane refers to horizontal directions around the head toward the right and toward the left. Horizontal directions are usually called angles of azimuth. For example: 0° azimuth is straight ahead (in front of your nose); 90° azimuth is directly to the right (in front of your right ear); 180° azimuth is straight back (directly behind your head); and 270° azimuth is directly to the left (in front of your left ear). Thus, an azimuth of 45° means 45° to the right, and 315° azimuth is the same as 45° to the left. By the way, it is perfectly fine to say “45° to the right” and “45° to the left.” It is also acceptable (and often confusing) to use positive angles for one direction and negative values for the other, such as +60° azimuth for 60° to the right, and –60° azimuth for 60° to the left. Fig. 2.17 Directions around the head. (a) The horizontal plane goes around the head horizontally from right to left, expressed in degrees of azimuth. (b) The medial (median) plane goes around the head vertically from front to back, expressed in degrees of elevation. Azimuths of 0° and 180° are both dead center between the two ears. The same thing is true for elevations such as straight up above the center of the head, 45° upward from directly in front, 30° downward from directly in front, and 70° upward from straight back. These are examples of directions in the medial (or median) plane. The medial plane is the same as going around the head vertically in the mid-sagittal anatomical plane, so that all locations on the medial plane are equidistant from the two ears. In the medial plane, 0° is straight ahead, 90° is straight up, and 180° is straight back. To appreciate how directional differences are represented acoustically at the ears, let us suppose that a loudspeaker is located at an azimuth of 45° to the right, as depicted in Fig. 2.18a. Even though the original sound is the same, it reaches the left (“far”) ear differently than it does the right (“near”) ear. The far ear is subjected to an acoustical shadow when the head obstructs the path of the sound (Fig. 2.18b). As a result, the sound reaches the far ear at a softer level than it reaches the near ear. This level disadvantage at the far ear is called the head shadow effect. The head shadow occurs for frequencies that can be obstructed by the head. This occurs for sounds with wavelengths that are short compared with the size of the head. Recall that wavelength gets shorter as frequency increases. Hence, the head shadow affects relatively higher frequencies, especially those over ~ 1500 Hz. This level difference between the ears is called an inter-ear (interaural) intensity difference. Fig. 2.18 (a) Sound coming from a loudspeaker off to the right side arrives differently at the two ears. (b) An acoustical shadow (the head shadow effect) occurs for high-frequency sounds because their wavelengths are small compared with the size of the head. (c) Because of their large wavelengths, low-frequency sounds are not subjected to a head shadow because they are able to bend around the head. The result of these differences at the eardrum can be seen by comparing the head-related transfer functions obtained when a loudspeaker is positioned at different locations around the head. Fig. 2.19 illustrates the azimuth effect by showing how the head-related transfer functions are different for the right ear when a loudspeaker is at an azimuth of 45° to the right compared with when is 45° to the left (i.e., 45° versus 315°). The sound level at the right eardrum is greater when the sound comes from 45° to the right (“near ear”) compared with when it comes from 45° to the left (“far ear”). Notice that the shape of the curve also changes depending on the azimuth. This graph shows the results at two representative azimuths. Curves obtained from many azimuths all around the head would reveal a continuum of these kinds of differences (Shaw 1974). Low frequencies have wavelengths that are long relative to the size of the head, so that diffraction can occur. In other words, they are able to bend around the head to the far ear with little if any loss of level (Fig. 2.18c). However, the sound will arrive at the near ear earlier than at the far ear, constituting an inter-ear (interaural) time difference. Interaural intensity and time differences provide the principal cues needed for directional hearing. What does the pinna do for us? It has long been known that any amplification provided by the pinna is essentially negligible in spite of its funnellike appearance, and that its main contribution to hearing is in the realm of sound source localization (Bekesy & Rosenblith 1958). (To appreciate the importance of the pinna in directional hearing, one has only to watch a cat orient its pinnae toward a sound source.) The pinna provides sound localization cues because its asymmetrical and irregular shape, ridges, and depressions modify the spectrum of a sound in a way that depends on the direction of the source (Blauert 1983). The simplest example is that sounds coming from the rear are obstructed by the pinna so that some of the high-frequency components of their spectra are attenuated compared with the same sounds arriving from the front. These kinds of pinna-related spectral differences are particularly important when inter-ear sound differences are negligible or absent. This is the case for localizations in the medial plane and/or when trying to localize sounds with just one ear. The sound signal that reaches the ear in the form of air vibrations must be transmitted to the cochlea, which is a fluid-filled system. The impedance of the cochlear fluids is much greater than the impedance of the air. As a result, most of the sound energy would be reflected back if airborne sound were to impinge directly on the cochlear fluids, and only ~ 0.1% of it would actually be transmitted. This situation is analogous to the reflection of airborne sound energy off the water’s surface at the beach, which is why you cannot hear your friends talking when your head is under the water. The middle ear system overcomes this impedance mismatch by acting as a mechanical transformer that boosts the original signal so that energy can be efficiently transmitted to the cochlea. Fig. 2.19 Head-related transfer functions for the right ear when the loudspeaker is located at azimuths of 45° to the right (“near ear” situation) versus 45° to the left (“far ear” situation). Based on the data of Shaw (1974) and Shaw & Vaillancourt (1985). The transformer function of the middle ear is accomplished by the combination of three mechanisms: (1) the area ratio advantage of the eardrum to the oval window, (2) the curved membrane buckling effect of the tympanic membrane, and (3) the lever action of the ossicular chain. The largest contribution comes from the area advantage. Here, the force that is exerted over the larger area of the tympanic membrane is transmitted to the smaller area of the oval window, just as the force applied over the head of a thumbtack is concentrated onto the tiny area of its point (Fig. 2.20). Pressure is force divided by area (p = F/A), so that concentrating the same force from a larger area of the eardrum down to a smaller area of the oval window results in a proportional boost in the pressure at the oval window. The curved membrane buckling mechanism is illustrated in Fig. 2.21. The eardrum curves from its rim at both ends to its attachment to the manubrium toward the middle. As a result, eardrum vibration involves greater displacement for the curved membranes and less displacement for the manubrium, which might be envisioned as a buckling effect. A boost in force accompanies the smaller displacement of the manubrium because the product of force and displacement must be the same on both sides of a lever (F1 × D1 = F2 × D2). The third and smallest contributor to the middle ear transformer mechanism is the lever action of the ossicular chain. Fig. 2.22 shows how the malleus constitutes the longer leg of this lever and the incus is the shorter leg, as well as the axis of rotation. How much of a boost does this transformer mechanism provide? To answer this question we can plug some representative values into the relationships just discussed. The area of the eardrum is roughly 85 mm2; however, only about two thirds of this area vibrates effectively (Bekesy, 1960), so that the effective area of the eardrum is something like 56.7 mm2. The area of the oval window is roughly 3.2 mm2. Hence, the area ratio is 56.7 to 3.2, or 17.7 to 1. The ossicular lever ratio is ~ 1.3 to 1. So far, the total advantage is 17.7 × 1.3 = 23 to 1. In terms of pressure the decibel value of this ratio would be 20 × log (23/1) = 27 dB. However, if we add the curved membrane buckling advantage of 2 to 1, the ratio becomes 23 × 2 = 46 to 1. In decibels of pressure, the total advantage now becomes 20 × log (46/1) = 33 dB. This is only an approximation; the actual size of the pressure advantage varies considerably with frequency (Nedzelnitsky 1980).
General Overview
Temporal Bone
Outer and Middle Ear
Pinna
External Auditory Meatus
Tympanic Membrane
Middle Ear
Ossicular Chain
Middle Ear Muscles
Eustachian Tube
Functioning of the Conductive Mechanism
Head-Related Transfer Function
Pinna Effect
The Middle Ear Transformer
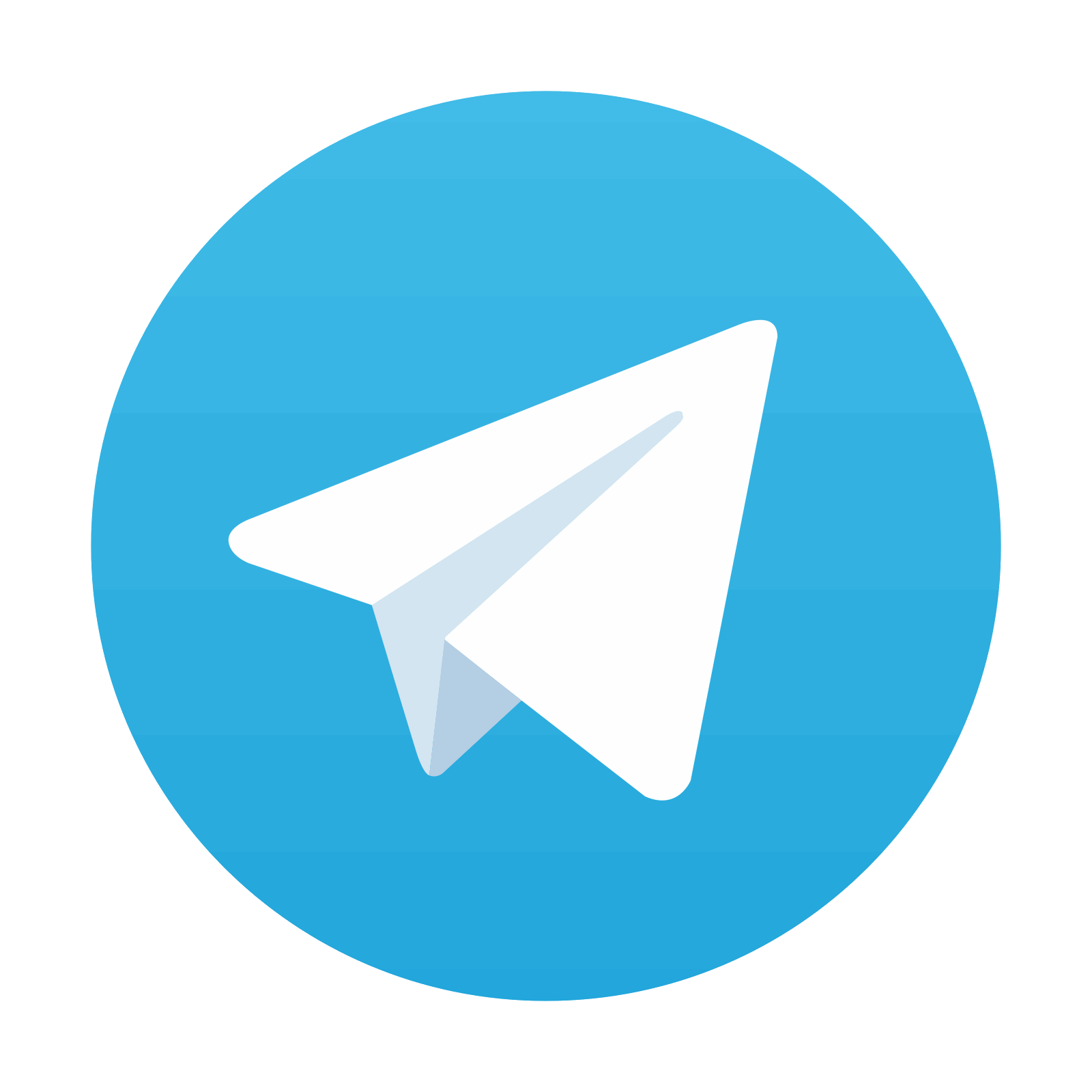
Stay updated, free articles. Join our Telegram channel

Full access? Get Clinical Tree
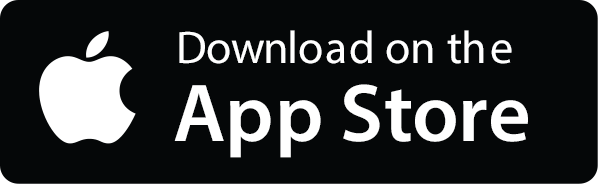
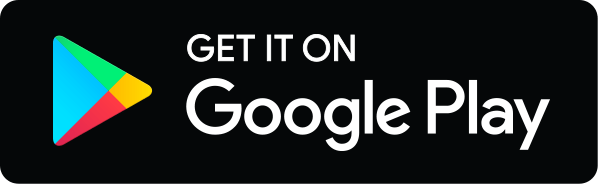