Vitreous Surgery in Proliferative Vitreoretinopathy
J. Michael Jumper
Andrew N. Antoszyk
Brooks W. McCuen II
The surgical management of retinal detachments complicated by severe proliferative vitreoretinopathy (PVR) has absorbed physicians’ interest for more than a century. Numerous observations of the clinical appearance and possible management of affected eyes have been reported, but it was the fundamental observations by Robert Machemer1,2 on the pathogenesis and treatment of PVR that acted as the catalyst for the vitreous surgery revolution. His work demonstrated that not only could previously unsalvageable eyes be successfully repaired but also that useful vision could be obtained. In this chapter we briefly review the history of vitreous surgery, the pathogenesis and classification of PVR, and the surgical management, results, and complications of vitreous surgery; we also discuss possible future advances in the management of this very complicated disorder.
HISTORY
The idea of releasing vitreoretinal traction dates back to the late 1800s when von Graefe3 and Deutschmann4,5 advocated cutting vitreous membranes and retina to manage retinal detachments. The importance of vitreous traction in the pathogenesis of retinal tears and subsequent retinal detachments was not appreciated until Leber’s observations in the latter half of the 19th century.6 Based on Leber’s findings, Gonin postulated that successful management of retinal detachments was feasible by complete drainage of subretinal fluid in conjunction with careful localization and treatment of retinal breaks.7,8 Many modifications soon followed, but one of the most important was popularized by Custodis9,10,11 and consisted of the use of an episcleral exoplant to close a retinal break.
The modern concept of removing the vitreous gel was pioneered by Kasner.12 In 1962, he performed the first open sky vitrectomy on an eye that had a severe anterior segment laceration. Using cellulose sponges and scissors, he removed the vitreous through the corneal wound and re-formed the eye with physiologic saline; ultimately useful vision was recovered. Kasner’s early work was done on traumatized eyes,12,13 but he later used the same technique to manage vitreous loss in patients undergoing cataract surgery.14
The next milestone in vitreous surgery was pioneered by Robert Machemer, who, having observed Kasner’s work, decided to develop a mechanical probe vitrectomy instrument for use through the pars plana.15,16 Machemer et al16,17 developed a series of prototype instruments that had irrigation, aspiration, and cutting abilities. The instrument, which was called a VISC (vitreous infusion suction cutter), had a cutter that was a small rotating knife, a suction system to facilitate vitreous and membrane removal, and an infusion system to keep the eye filled with saline. The first closed vitrectomy through a pars plana incision was performed in 1970 using this instrument. The patient had an uncomplicated vitreous hemorrhage and achieved 20/50 (6/15)* vision.15 The success of this procedure demonstrated that vitreous surgery could be performed in a “closed system” and was the initiating event of modern pars plana vitreous surgery.
Rapid advances followed.18 The modern-day microscope, which was first conceived of by Littman19 in 1954, consisted of a telecentric device with a fixed working distance, a manually adjusted variable magnification, and a paraxial illumination source. It was later modified by Parel et al17 in 1974 with the addition of motorized functions controlled by a foot pedal. The automated features included zoom magnification, fine focus, and two-dimensional (x–y) movement of the microscope head in a horizontal plane. The microscope provided stereopsis and magnification, which facilitated delicate intraocular manipulations. To perform surgery in the posterior segment under the microscope, the use of a contact lens was required. The original operating contact lens was a modified Goldmann planoconcave fundus contact lens that had irrigation added, permitting it to be floated on the corneal surface. This facilitated work in the anterior vitreous cavity, but working posteriorly was impaired because of light reflections and light dispersion. This problem was eventually eliminated with the advent of fiberoptic endoilluminators, which had the added benefit of enhancing resolution of fine structures under high magnification. Another helpful advance was the development of intraocular diathermy using radio-frequency electromagnetic waves to help control intraocular bleeding.17,19,20,21 Although this was proven effective, it was rarely required since most bleeding could be controlled by increasing the intraocular infusion pressure.17
Another significant advance came in 1972 when O’Malley and Heintz22,23 reported their “divided-system instrumentation” approach to pars plana vitreous surgery. Functions that had originally been relegated to a single instrument such as the VISC were now divided among two instruments and a separate infusion cannula. Divided-function instruments led to three-port and at times four-port surgery, permitting the reduction in the diameter of instruments. A rapid advance in the design and production of small-gauge instruments such as fiberoptic endoilluminators,22,23,24 microcutters,18,23,25 bipolar coagulators,26,27 micropicks,28,29,30,31 microforceps,32,33,34 microscissors (manual28,32,35,36,37,38,39 and automated),40,41 endophotocoagulators,42,43,44,45 and endocryoprobes46,47 ensued. The primary disadvantage of the divided-system approach was that it required the surgeon to learn a bimanual surgical technique. The advantages of the technique included (i) increased control over the rotation of the globe; (ii) easy exchange of instruments; (iii) maintenance of the intraocular pressure during instrument exchanges and surgical manipulations; (iv) facilitation of delicate membrane dissections; and (v) increased ease of performing infusion exchanges (e.g., fluid–gas, gas–silicone, fluid–silicone, fluid–perfluorocarbon liquid, perfluorocarbon liquid–silicone). Advances in technology and surgical techniques have more recently fostered a movement back to multifunction instruments. For instance, fiberoptics were added to infusion probes, picks, and microforceps.48,49,50,51,52,53
Today the emphasis is being placed on the development of multifunction instruments that remain of small diameter and permit true bimanual surgery. An illuminated blade,52,54 cannulated subretinal fluid aspirator,55 iris retractors,56,57,58 modified infusion cannulas,59,60 and 25-gauge microinstruments (25-gauge vitreous cutter, 25-gauge microscissors, and a vitreous membrane dissector)61 are just a few of the recent additions to the rapidly growing list of available tools for the vitreous surgeon. The limits of technology and the surgical skill of vitreoretinal surgeons appear to be approaching. There remain, however, retinal detachments complicated by severe PVR that cannot be satisfactorily repaired. Understanding the pathogenesis of PVR will help us identify new modalities of treatment.
PROLIFERATIVE VITREORETINOPATHY
SIGNIFICANCE
The most common cause for ultimate failure after retinal reattachment surgery is the development of PVR.62,63,64,65 Current techniques of retinal detachment repair, including scleral buckling, vitrectomy, and pneumatic retinopexy, are successful in up to 90% of cases, but 10% still ultimately fail because of the development of PVR. In the prospective, multicenter scleral buckling versus primary vitrectomy for rhegmatogenous retinal detachment study (SPR), PVR (grade B or C) developed in 14% of phakic patients and 19% of pseudophakic patients, respectively.66 To manage these patients successfully one must understand the pathology, pathobiology, and pathophysiology of the disease along with its natural biologic behavior. Successful treatment requires a complete understanding of the anatomic changes as the disease progresses, the intended goals of treatment, and the treatment alternatives.67,68,69,70,71
PATHOLOGY
PVR represents a pathologic growth of cells and extracellular matrix within and on the anterior and posterior surfaces of the retina, in the vitreous cavity (particularly the posterior hyaloid face), and in the vitreous base (Figs. 58-1 and 58-2).2,72,73,74,75,76,77 Predisposing factors that contribute to the development of PVR include chronic retinal detachments (>1 month), aphakia, vitreous hemorrhage, and increasing size of retinal breaks. In particular, patients with giant retinal tears (tear greater than three clock-hours in size) with a folded-over posterior flap are at high risk for PVR.78
The proliferative process can also occur anteriorly with growth of cellular membranes over the pars plicata of the ciliary body, the posterior surface of the iris, and even the pupillary margin.79,80 Cells contained within these membranes have contractile properties that can result in traction on either side of the retina, the ciliary body, and the posterior iris surface. Epiretinal proliferations may occur focally, giving rise to full-thickness radiating retinal folds in the pattern of a star or diffusely giving rise to large areas of fixed, irregular retinal folds.63 Subretinal proliferations usually begin as membranous sheets that are not clinically visible. With contraction the membranes develop numerous holes that may give a moth-eaten appearance to the posterior retinal surface. As the holes enlarge the membranes roll up, forming strands or bands that may be white or pigmented.81,82,83 Proliferation and contraction of membranes at the junction of the detached hyaloid face and peripheral retina (usually equatorial in location) results in central displacement of the retina.84 The contraction forces result in radial retinal folds with a funnel-shaped retinal detachment posterior to and stretching of the retina anterior to the junction zone (Fig. 58-3A). Last, anterior proliferation, which frequently but not exclusively occurs in the remnants of the vitreous base after vitreous surgery, can result in dragging of the peripheral retina and posterior vitreous base toward the membranes’ anterior attachments (pars plicata, posterior iris surface, pupillary margin). The resultant pathoanatomy of the contraction is a circumferential peripheral trough of varying width and depth (Fig. 58-3B).79,84
It is commonly believed that the primary effector cell for the development of PVR is the retinal pigment epithelial (RPE) cell.2,74,84 Ultrastructural analysis of epiretinal and subretinal membranes removed from human eyes with PVR and animal eyes with experimentally induced PVR have shown a predominance of RPE cells.72,75,80,81,82,83,85 Additional cell types identified in these specimens include glial cells (fibrous astrocytes), macrophages, fibrocytes, and myofibroblast-like cells. These latter cells usually have characteristics of fibrocytes, but occasionally they contain structures more commonly seen in RPE or glial cells.73,74,76,79,80,81,82 The exact origin of these cells is controversial,86 but they probably represent RPE and glial cells that are capable of transforming into myofibroblast-like cells with the capacity to synthesize collagen and contract.2,73,87,88 Charteris et al77 have shown that inner retinal Muller cell hypertrophy occurs in anterior PVR and that “glial bridges” form between the Muller cells and epiretinal membranes. Their observations suggest that this is a cause of retinal foreshortening and incomplete membrane peeling often observed in PVR surgery.
A frequent finding in epiretinal membrane formation is the deposition of pigmented dots or clumps on the inner retinal surface. They tend to be irregularly distributed but because of gravity they are most concentrated over the inferior retina.89 Laqua and Machemer74 have shown histologically that these dots are composed of pigmented epithelial macrophages. Their contributory role to the actual production of collagenous epiretinal membranes is believed to be negligible.
The composition of subretinal membranes seems to depend somewhat on the age of the detachment. The deposition of RPE cells on the posterior retinal surface can occur within 1 week of a detachment.2,90 The appearance of subretinal white precipitates (presumably pigment epithelial macrophages) are the earliest ophthalmoscopic sign of subretinal membrane formation.74,90 They tend to be irregularly distributed, but the heaviest aggregates are usually seen on the inferior retina. The growth pattern of these membranes is diffuse at first, but with cellular contraction, weak areas break, holes result, and subretinal strands are formed. The pigmentation of the subretinal bands depends on the concentration of pigment in the cells that make up the proliferation. As collagen is produced the membranes become more opaque and take on a yellow-white hue. RPE cells are the predominant component of the subretinal proliferations. In contrast, glial cells are rarely seen. The one exception occurs in chronically detached, gliotic-appearing retinas where extensive subretinal glial membranes are found in association with a dramatic loss of photoreceptors. The membranes are typically invisible on ophthalmoscopy but may give the retina a moth-eaten appearance.2,72,73,74,81,90
PATHOBIOLOGY
The pathobiology of PVR is not yet fully understood, although research continues to shed new light on its basic mechanisms.2,72,74 When a retinal tear occurs, evidence suggests that RPE cells are released through the break into the vitreous cavity (Fig. 58-4). As the retina detaches, some RPE may remain adherent to the retinal flap. With time these fall off and seed the vitreous cavity. In addition there is in vivo91 and in vitro92 experimental evidence to suggest that transscleral cryotherapy to the retinal breaks disperses large numbers of viable RPE cells into the vitreous cavity.93 The dispersed or stimulated RPE cells have been shown to be capable of releasing chemoattractants, inducing migration and chemotaxis. Experimentally, human RPE cells have been shown to release a chemoattractant for retinal astrocytes.94 They also produce and release platelet-derived growth factor (PDGF), which is a known mitogen and chemoattractant for human RPE and glial cells.95,96 Studies have also demonstrated that RPE cells are a source of transforming growth factor-β (TGF-β), which stimulates fibroblasts to proliferate and synthesize collagen and fibronectin.97 TGF-β is also a known chemoattractant for monocytes.98,99 Other soluble mediators implicated in the development of PVR include the following: pigment epithelium–derived factor (PEDF),100 tumor necrosis factor alpha (TNF-α),101 monocyte chemotactic protein-1 (MCP-1),102,103,104 and vascular endothelial growth factor (VEGF).105
The breakdown of the blood–retina barrier in patients with retinal detachments is demonstrated by flare in the vitreous cavity. This breakdown is exacerbated by cryotherapy and/or surgical intervention. Disruption of the blood–retina barrier permits serum with its various growth factors (PDGF, TGF-β) and components of inflammation (e.g., complement, immunoglobulins [IgG, IgA, IgE]), and extracellular matrix (e.g., fibronectin) to gain access to the vitreous cavity.106 Serum itself has been shown to be a potent stimulator of RPE cell migration and is chemotactic for human RPE107 and retinal derived glial cells.108 The primary factor in serum responsible for RPE migration and chemotaxis has been shown in vitro to be fibronectin.107 Fibronectin is an integral component of extracellular matrix and has been found to be a potent stimulator of cellular migration and proliferation for various cells.109 It is interesting that in vivo studies looking at vitrectomy specimens from patients with PVR have shown levels of fibronectin seven times higher than those in eyes with long-standing macular puckers or uncomplicated retinal detachments.110 A secondary but still potent stimulator of RPE migration found in serum is PDGF (Fig. 58-4).95
The role of inflammation in the pathogenesis of PVR remains to be defined. Studies, however, have identified various complement components (C1q, C4, C3, C3 c, C3 d) in specimens obtained from patients undergoing surgery for PVR, suggesting that the complement system is activated in patients with PVR.111,112,113 Some macrophages and B or T8 lymphocytes have also been identified.113,114 These findings, when taken together, suggest the possibility of an autoimmune response. The expression by various cells such as RPE, nonpigmented ciliary epithelium, and astrocytes of class II histocompatibility antigens (HLA-DR, HLA-DQ) also lends support to an autoimmune reaction with complement activation and possible cell-mediated immunity (Fig. 58-4).112,113,115
PATHOPHYSIOLOGY
Among the normal functions of the retinal pigment epithelium are the renewal of photoreceptor outer segments and the maintenance of a deturgesced subretinal space. When a retinal tear occurs, RPE cells normally adherent to Bruch membrane disperse and/or migrate into the vitreous cavity (predominantly inferiorly because of gravity),89,116 as well as onto the epiretinal and subretinal surfaces of the retina. The separation of RPE from Bruch membrane may be owing, in part, to proteolytic enzymes and proteins with counteradhesive properties.117 The dispersion of viable RPE cells is the inciting event in the pathogenesis of PVR (Fig. 58-4) and is increased when cryotherapy, which weakens the adherence between the RPE and its basement membrane, is followed by scleral depression.92,93 In addition, the blood–retina barrier is further disrupted.106 Serum with inflammatory mediators, growth factors, and extracellular matrix components enters the vitreous cavity. Freed viable RPE cells are exposed to a different extracellular milieu that stimulates them to proliferate and undergo a morphologic change from an epithelial-like appearance to one resembling a fibroblast or mesenchymal cell.118 The RPE cells release substances that are capable of attracting other cells such as astrocytes,94 fibroblasts,119 and monocytes.98 These cells are stimulated to proliferate by intraocular or plasma-derived growth factors.97,120 Some of these same cells are capable of producing growth factors, extracellular matrix (fibronectin), and collagen.93,121 Fibronectin and growth factors increase in the vitreous cavity and attract more pigment epithelial and fibroblast-like cells.97,107,110 More collagen is produced, cells contract, collagen matures, and retinal traction results. Thus, a potential PVR life cycle is set up.
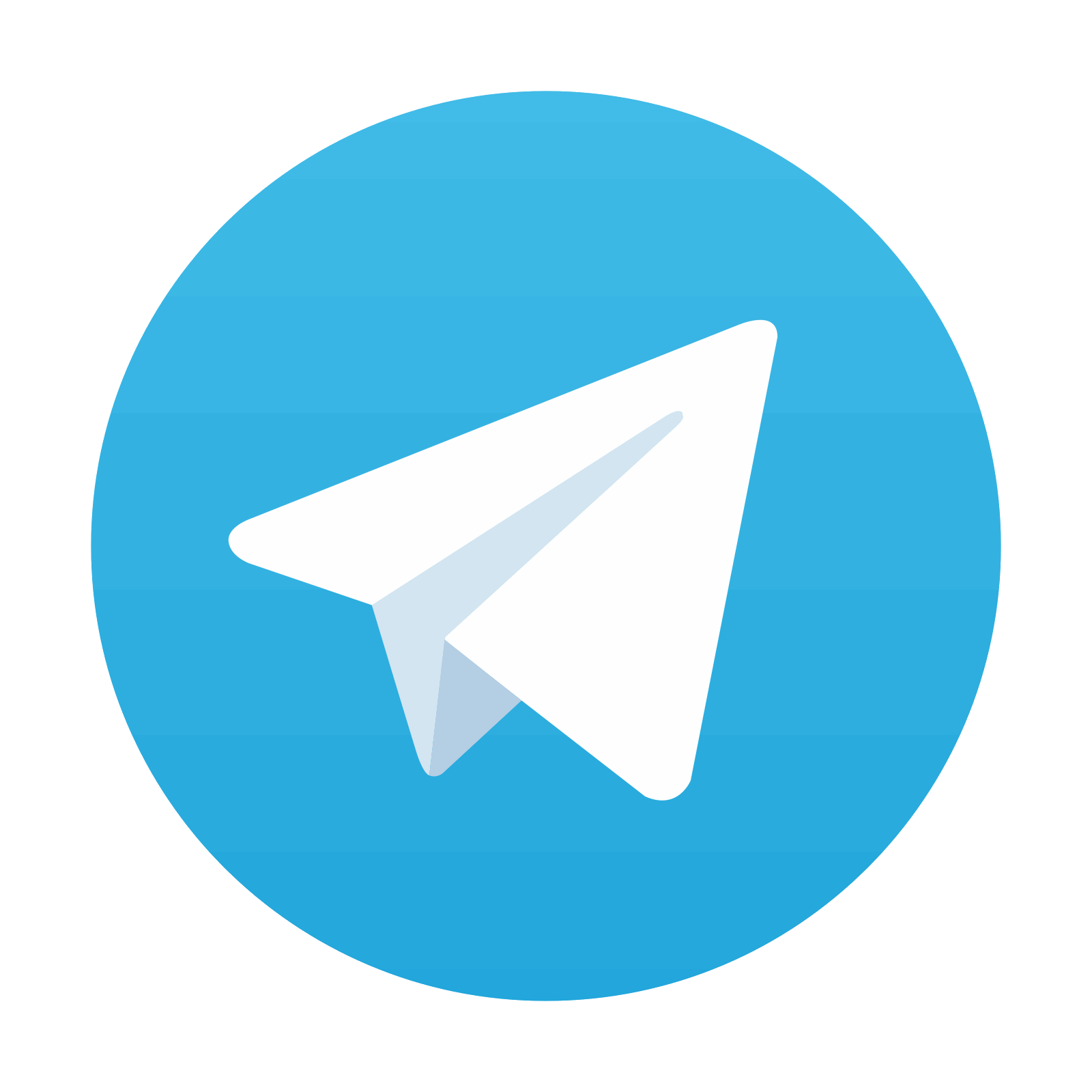
Stay updated, free articles. Join our Telegram channel

Full access? Get Clinical Tree
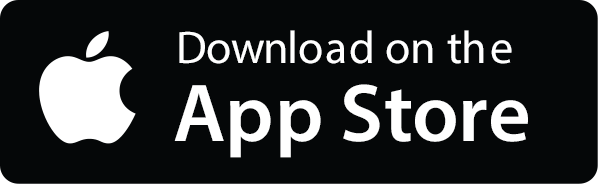
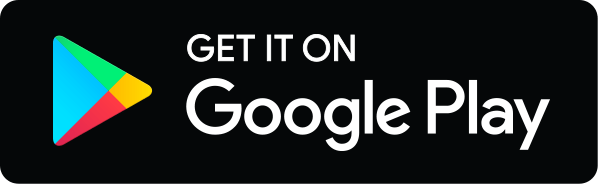