# of up-regulated metabolites
# of down-regulated metabolites
2D
HCK + T3
3
20
HCF + T3
15
1
3D
HCK + T3
56
3
HCF + T3
4
3
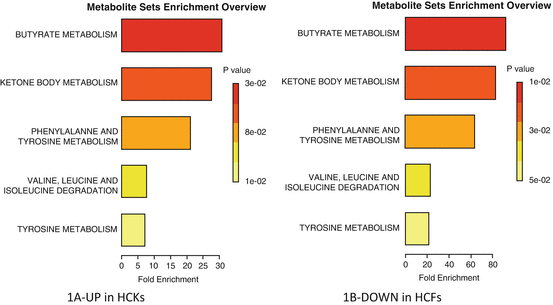
Fig. 5.1
Summary of pathway enrichment analysis in 2D system. Above is a display of the diversity of signaling pathways that are enriched on the basis of all the metabolites passing filtering criteria. The most significant p-values are in red while the least significant are in yellow and white. (a) Pathways affected based on metabolites that were at least twofold up-regulated in HCKs compared to HCFs, following TGF-β3 stimulation. (b) Pathways affected based on metabolites that were at least twofold down-regulated in HCFs compared to HCKs, following TGF-β3 stimulation
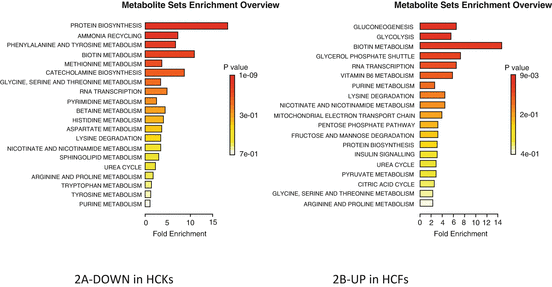
Fig. 5.2
Summary of pathway enrichment analysis in 2D system. Above is a display of the diversity of signaling pathways that are enriched on the basis of all the metabolites passing filtering criteria. The most significant p-values are in red while the least significant are in yellow and white. (a) Pathways affected based on metabolites that were at least twofold down-regulated in HCKs compared to HCFs, following TGF-β3 stimulation. (b) Pathways affected based on metabolites that were at least twofold up-regulated in HCFs compared to HCKs, following TGF-β3 stimulation
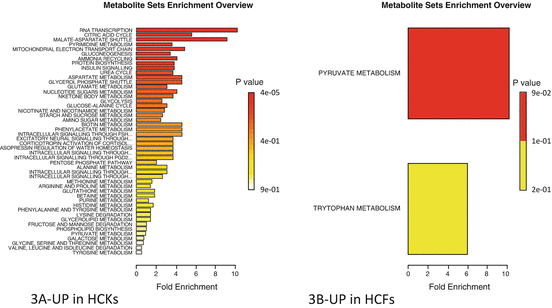
Fig. 5.3
Summary of pathway enrichment analysis in 3D system. Above is a display of the diversity of signaling pathways that are enriched on the basis of all the metabolites passing filtering criteria. The most significant p-values are in red while the least significant are in yellow and white. (a) Pathways affected based on metabolites that were at least twofold up-regulated in HCKs compared to HCFs, following TGF-β3 stimulation. (b) Pathways affected based on metabolites that were at least twofold up-regulated in HCFs compared to HCKs, following TGF-β3 stimulation
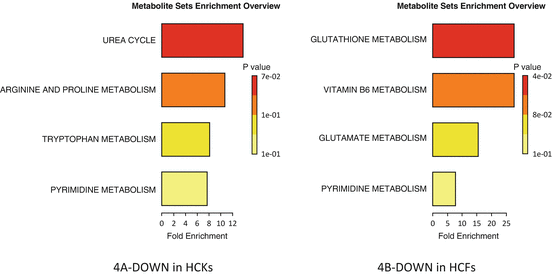
Fig. 5.4
Summary of pathway enrichment analysis in 3D system. Above is a display of the diversity of signaling pathways that are enriched on the basis of all the metabolites passing filtering criteria. The most significant p-values are in red while the least significant are in yellow and white. (a) Pathways affected based on metabolites that were at least twofold down-regulated in HCKs compared to HCFs, following TGF-β3 stimulation. (b) Pathways affected based on metabolites that were at least twofold down-regulated in HCFs compared to HCKs, following TGF-β3 stimulation
5.3 Results
5.3.1 Expression of Metabolites
We have identified 256 endogenous water-soluble metabolites of which more than 60 were significantly regulated between groups. We performed the analysis using only the metabolites that were up or downregulated by at least twofold. This cutoff was chosen in order to ensure that we included only the vastly abundant metabolites. We investigated both a conventional 2D system and a 3D self-assembled ECM. More specifically, in 2D cultures, we found three metabolites significantly upregulated in HCKs when stimulated with TGF-β3 and 20 downregulated. In contrast, we found 15 upregulated and 1 downregulated in HCFs (Table 5.1). When the cells were stimulated to secrete their own ECM, using our 3D system, metabolic regulation was altered. In HCKs, 56 metabolites were upregulated and 3 were downregulated, while 5 were upregulated and 3 downregulated in HCFs. Surprisingly, in our 3D model there were seven metabolites that were not found at all, in HCKs, with or without TGF-β3 and four in HCFs. Furthermore, we found eight metabolites that were completely missing in HCK Controls and one in HCF Controls. Those were identified only upon TGF-β3 stimulation. While the missing metabolites are interesting, further investigation is needed before we can draw any conclusions. This chapter will discuss specific changes in cellular metabolism based on the above findings.
5.3.2 Conventional 2D Cultures: Metabolic Activity
Using the Metaboanalyst software, we were able to dissect which metabolic pathways are affected based on our raw data. In cells that are conventionally cultured (2D) we found significant differences between HCKs and HCFs. As shown in Fig. 5.1a the predicted metabolic pathways that are affected in 2D—HCKs based on the three metabolites that are upregulated following stimulation with T3 are: Butyrate, Ketone body, and Phenylalanine/Tyrosine metabolism. All of these are associated with energy production and suggest that T3 stimulates activation of HCKs. Butyrates are important for cells since they provide energy. Without them cells undergo apoptosis [20]. In cornea, butyrate was found to stimulate fibronectin synthesis in cultured rabbit corneal blocks in situ [21]. Ketone bodies are three different water-soluble biochemicals that are produced by the liver from fatty acids and are another source of energy for the cells. In cornea, not much is known, however levobunolol, a ketone-containing drug, has been used on rabbit eyes [22]. That study concluded that levobunolol had significant effects on energy metabolism of corneal epithelium, iris-ciliary body, and lens. Phenylalanine/Tyrosine metabolism is considered to be one of the key steps in signal transduction and regulation of enzymatic activity. Any defects in the Phenylalanine/Tyrosine metabolism may lead to a variety of clinical disorders, including Tyrosinemia type II which results in painful corneal lesions and scars [23].
Surprisingly, the same pathways were predicted to be affected with HCFs following stimulation with TGF-b3, only this time the metabolite was downregulated suggesting that HCFs and HCKs metabolism is differentially affected by T3 (Fig. 5.1b). This would suggest that T3 is acting on HCFs positively by reducing the stress levels and moving the cells back towards HCKs.
On the other hand, based on the 20 metabolites downregulated upon T3 stimulation of HCKs, the pathways affected were: protein biosynthesis, ammonia recycling, phenylalanine/tyrosine, and biotin metabolism. Ammonia recycling is important to be kept under control. Organisms that cannot easily and quickly remove ammonia usually have to convert it to some other substance, like urea which is much less toxic. Insufficient removal of ammonia is toxic. This might not be important on an in vitro model, such as this one, where toxins are released into the media but it definitely provides an initial indicator. In cornea, not much is known about ammonia metabolism; however exposure to gaseous ammonia can cause blindness [24]. Phenylalanine/tyrosine seems to be affected both from metabolites that are up and downregulated with T3 (Fig. 5.2a) suggesting a more complex mechanism.
Based on the 15 metabolites that were upregulated with HCFs that were stimulated with T3, the pathways affected included: glucogenesis (GNG), glycolysis, RNA transcription, and biotin metabolism (Fig. 5.2b). GNG and glycolysis are part of the same process and are vital in humans for maintaining blood glucose levels to normal. In cornea GNG is important as glucose is used as fuel source and has been linked to wound healing. The other two pathways RNA transcription is clearly important for gene expression [25, 26] and biotin metabolism is vital for cell growth and production of fatty acids [27]. Clearly T3 presence in HCFs has a predicted positive effect in terms of the cellular metabolism.
5.3.3 Self-Assembled 3D Cultures: Metabolic Activity
Not surprisingly, the metabolic pathways affected on cells that were cultured in our 3D self-assembled model were different between HCKs and HCFs. Figure 5.3a is representation of the affected metabolic pathways in 3D—HCKs based on the 56 metabolites that are upregulated following stimulation with T3. The most significantly affected pathway is the RNA transcription which is the first step of gene expression. In other words HCKs in 3D constructs can be stimulated by T3 to initiate gene expression which can be vital if we are to target specific genes. Furthermore, we found that citric acid cycle, also known as the tricarboxylic acid cycle (TCA cycle), or the Krebs cycle [28–30], is affected. TCA cycle is a key component of the metabolic pathway by which all aerobic organisms generate energy. TCA also provides precursors of certain amino acids as well as the reducing agent NADH (Nicotinamide adenine dinucleotide) that is key in numerous biochemical reactions. In fact enzymes that are known to be involved or affect NADH metabolism are normally targets for drug discovery. Later in this chapter, the TCA and specific metabolites altered in both HCKs and HCFs are shown. Another metabolic pathway that is affected and is linked to energy is the malate-aspartate shuttle. The malate-aspartate shuttle is a biochemical system that generates ATP via translocation of electrons across cellular membranes [31]. Even though in cornea there are currently no studies specifically reporting the activity of this pathway, it is easily understood that there is a key role of the malate-aspartate shuttle, at least in terms of cellular energy. Other notable pathways, as with the 2D system, include GNG and protein biosynthesis; both vital in human’s blood glucose levels and in cornea’s fuel supply.
Surprisingly though, the metabolic pathways upregulated by T3 stimulation in HCF—3D constructs were minimum. Only, four metabolites were significantly upregulated: Acetylphosphate, 2,3-dihydroxybenzoic acid, Xanthurenic acid, and Cholesteryl sulfate. Based on these, Metaboanalyst predicted that two pathways are affected: Pyruvate and Tryptophan metabolism. The significance, as indicated by the p value in Fig. 5.3b suggests that only pyruvate metabolic pathway was altered. Pyruvate metabolism however, is very important. In fact, it is a key intersection in the network of metabolic pathways. Pyruvate can be converted into carbohydrates via gluconeogenesis, to fatty acids or energy through acetyl-CoA, and to the amino acid alanine. It is also the output of the anaerobic metabolism of glucose [32] which we saw being affected by T3 stimulation on most of our systems. Pyruvate alone and/or in combination with Lactate is known to be indicators for oxidative stress in cornea as well as being regulated in cyclodiathermy [33].
T3 stimulation caused some metabolites to be downregulated on 3D constructs and Fig. 5.4a, b shows the predicted pathways affected. For HCKs the Urea cycle and the arginine/proline metabolism (Fig. 5.4a) was affected and for the HCFs the Glutathione and Vitamin B6 metabolism (Fig. 5.4b). Urea was also affected, indirectly, via the ammonia recycling on our 2D system indicating similar effect in 2D and 3D systems. The effect on arginine/proline pathway is expected since a key component of the arginine/proline metabolic pathway is ornithine; and ornithine is primarily an intermediate of the urea cycle. In cornea, arginine downregulation is important and has been reported as a key mechanism for corneal allografts immune privilege [12]. Finally, HCFs showed regulation of Vitamin B6 and Glutathione, with the two of them actually related. Glutathione is vital for various protective roles in the human body and the Vitamin B6 as pyridoxal-5′-phosphate (PLP) required in the formation of glutathione precursors [34]. Glutathione has been reported multiple times as an oxidative stress indicator while Vitamin B6 depletion have been shown to increase glutathione concentrations in rat liver [35, 36] and human plasma [37].
5.3.4 TCA Regulation
In this paragraph we present a schematic color-coded representation of the specific metabolites that were up- or downregulated within the TCA cycle on our 2D and 3D systems with the two different cell types. The schematic was generated based on the raw data obtained from the samples.
TCA cycle is a series of enzyme-catalyzed chemical reactions that form a key part of aerobic respiration in cells. This cycle is also known as the Krebs cycle. The greatly simplified schematic shown here starts with glucose (without actually showing the glycolysis cycle) and continues with pyruvate which is the end product of glycolysis and the first step of all types of cell respiration in the mitochondria. TCA cycle is not only part of the glucose pathway for the purpose of breaking down of glucose but is also part of all metabolites, including sugars, amino acids, and fatty acids. It is primarily fed from glutamine which is then converted to glutamate via glutaminase and then catalyzed to α-ketogluterate via glutaminate dehydrogenase. Within each of these groups of molecules there is always a pathway that leads into the TCA cycle. It is easily understood that in order for us to determine and characterize the metabolic differences in HCKs and HCFs we need to look at the TCA cycle regulation first. Figure 5.5 shows the metabolites within the TCA cycle that were regulated in our 2D system. Oxaloacetate products such as Asparagine, Lysine, Methionine, and Isoleucine were downregulated in HCKs, along with Arginine which is downstream from α-ketoglutarate, and Alanine. No metabolites were upregulated in HCKs. On the other hand, HCFs showed five upregulated metabolites; Alanine, Lactate, Citrate, Isocitrate, and Lysine. Note that Alanine and Lysine are the common and differentially regulated ones between HCKs and HCFs.
