Fig. 21.1
Schematic illustration of normal development, de-differentiation, and transdifferentiation. The top of the hill represents an immature status of the cell, and the bottom represents a differentiated status. (a) In normal development, a pluripotent cell (a red ball on the top of the hill) rolls down to the bottom to differentiate into a mature cell (a gray ball). (b) A mature cell can be reprogrammed to become a pluripotent cell (induced pluripotent stem cell (iPSC)), which is represented by rolling up the hill. (c) A mature cell becomes another type of mature cell without producing a pluripotent intermediate, which is represented by jumping over the hill. This schematic illustration was modified from that presented in [1]
21.1.2 Transdifferentiation and Transcription Factors
Originally, transdifferentiation protocols used the overexpression of only 2–5 transcription factors, which are also called “reprogramming factors.” To find a combination of the minimal set of transcription factors, most experiments used 5–20 transcription factors that satisfied the following criteria: (1) the transcription factors are specifically expressed in embryonic or mature target cells or their progenitor cells and are important for the development, maintenance, or both of the desired cell type and (2) target organs exhibit severe defects when the transcription factors are mutated [5–9]. After confirming that simultaneous transfection of all the transcription factors transformed fibroblasts or other types of cells into the desired cells, the effects of withdrawing individual transcription factors were observed to determine the minimal set of genes that can transform fibroblasts into the desired cells. For example, transdifferentiation of pancreatic β-cells requires three reprogramming factors: Ngn3, Pdx1, and Mafa [5]. All of them are important for the development of the pancreas and pancreatic β-cells, although there are many other factors that play pivotal roles in the development of pancreatic β-cells. However, the reasons why only these three factors are sufficient to induce pancreatic β-cells remain to be elucidated. Currently, a combination of microRNAs and transcription factors is also used to induce more efficient transdifferentiation [10].
21.1.3 Advantages and Disadvantages of Transdifferentiation
Although regenerative therapy using transdifferentiation is a promising method, there are some problems in its application. One problem is that no set of reprogramming factors has been shown to work robustly in both mouse and human cells, as observed in cardiomyocyte regeneration. This indicates that the results of animal in vivo experiments cannot be extrapolated to clinical applications. This situation is different from that of iPSCs, which can be induced by same set of reprogramming factors in both mice and humans. Enormous efforts will be necessary to understand the difference between animal and human studies in transdifferentiation.
Other problems include successful transdifferentiation only occurring with in vivo experiments [5] and problems with reproducibility [4, 11], indicating that more detailed analyses are still required. However, transdifferentiation will provide a more promising method for regenerative medicine than the stepwise differentiation from pluripotent stem cells. To achieve regeneration by transplanting pluripotent stem cells (embryonic stem cells or iPSCs) that are induced to develop into desired cell types, sufficient numbers of cells must be prepared through efficient expansion. The induced cells must be efficiently delivered into the damaged organs and properly engrafted. Engrafted cells may require treatment to survive in the donor sites. Potential tumorigenicity of residual stem cells [12] and possible immune rejection also need to be considered. These pitfalls of cell-based therapies can be avoided by delivering reprogramming factors directly into damaged organs to induce regeneration through transdifferentiation.
21.2 Transdifferentiation and Hair Cells
21.2.1 Transdifferentiation and Avian Hair Cells
In the context of the regeneration of auditory or vestibular hair cells, transdifferentiation is an important phenomenon. In birds, vestibular hair cells regenerate throughout their life [13] and even auditory hair cells can regenerate after injury [14, 15]. Two underlying mechanisms of these regenerative processes have been identified. One is the proliferation of supporting cells that surround hair cells [14, 15]. Incorporation of 3H-thymidine showed that supporting cells induced mitosis and daughter cells contributed to the regenerated hair cells. The other mechanism was the direct transdifferentiation of supporting cells into hair cells [16, 17]. Pharmacological blockade of mitosis with Ara-C or aphidicolin after hair cell injury in mature birds caused cell cycle arrest but did not prevent new hair cell production, indicating that newly regenerated hair cells were produced by mechanisms other than proliferation [16, 17]. Transdifferentiation was also morphologically confirmed by the identification of cells that had features of both hair cells and supporting cells [18]. Transdifferentiation may occur readily between hair and supporting cells in birds because both cells share common progenitors during their development [19].
21.2.2 Transdifferentiation and Mammalian Hair Cells
In mammals, hair cells in the postnatal stage never regenerate spontaneously because the proliferation of sensory epithelia ceases during the embryonic stage. This was proved by conducting experiment using 3H-thymidine incorporation [20]. In addition, no spontaneous transdifferentiation from supporting cells to hair cells has been observed in mammals. However, with accumulating knowledge of inner ear development, overexpression of a transcription factor and the manipulation of signaling pathways have been attempted in order to induce the regeneration of hair cells.
21.2.2.1 Transdifferentiation and Atoh1
Among several transcription factors related to the development of hair cells, Atoh1 is the most promising factor for the regeneration of hair cells. Atoh1 is one of the proneural basic helix-loop-helix transcription factors that forms a dimeric complex with other basic helix-loop-helix transcription factors to activate transcription of target genes in order to maintain the cell cycle or to promote differentiation. Disruption of Atoh1 with gene targeting caused complete loss of hair cells [21, 22], as well as supporting cells [22] in mice. On the basis of this result, Atoh1 was overexpressed in postnatal mammalian cochlea in vitro by using electroporation [23] and in vivo by using adenovirus vectors [24, 25], to induce transdifferentiation of hair cells. Atoh1 overexpression in rat cochlear explant culture showed induction of ectopic hair cell-like cells in the greater epithelial ridge [23], suggesting the transdifferentiation of epithelial cells in this region to hair cell-like cells. This study confirmed that the transfected cells expressed hair cell markers and that they had morphological characteristics of hair cells including cuticular plate and stereocilia although the functions of hair cells were not examined. Overexpression of Atoh1 in guinea pig cochleae in vivo using an adenovirus vector induced the development of ectopic hair cell that received neural axons from auditory nerves [25]. The source of transdifferentiated hair cells includes interdental cells, inner sulcus cells, and Hensen’s cells. Moreover, when Atoh1 was overexpressed in the guinea pig cochleae after pharmacological hair cell injury, functional recovery as well as hair cell regeneration was observed [24]. In this study, the regenerated cells showed a mixed phenotype with features of both outer hair cells and supporting cells, as observed in transdifferentiation of hair cells in birds. However, Atoh1 overexpression in the cochlea using transgenic mice showed that the competency of hair cell transdifferentiation was limited to the neonate and juvenile stages [26, 27]. Moreover, Liu et al. showed that permanent expression of Atoh1 induced hair cell loss [27]. These results indicate that Atoh1 alone is insufficient as a reprogramming factor and Atoh1 should be combined with other transcription factors to induce a more complete transdifferentiation of hair cells.
21.2.2.2 Transdifferentiation and Notch Signaling
As a cell fate determinant, Notch signaling has important roles in various tissues including the central nervous system, hematopoietic system, and inner ears. Genetic disruption of Jag2, one of the ligands of Notch signaling, caused an increase in the numbers of hair cells and decreased the number of supporting cells [28], suggesting that cell fate determination between hair and supporting cells is regulated by Notch signaling. This was confirmed by more complete blockade using gene targeting of Rbpj, a common transcriptional regulator downstream of all Notch receptors [29, 30]. These studies using Rbpj mutant mice also showed that Notch signaling is necessary for the maintenance of sensory epithelium progenitors. On the basis of these findings, transdifferentiation from supporting cells to hair cells was examined by inhibiting Notch signaling. A conditional knockout of Rbpj in neonatal mouse cochlear explants showed that myosin7a-positive hair cell-like cells were induced from non-sensory epithelial cells in the cochlea by cell autonomous effects of Rbpj gene deletion [31], although the induced cells did not have stereocilia. Similar results were obtained through the pharmacological inhibition of Notch signaling by using a γ-secretase inhibitor [31] (Fig. 21.2). γ-Secretase inhibitor treatment on adult cochlea with injured hair cells caused the transdifferentiation of hair cells from supporting cells and functional recovery, although the hearing threshold change was very small [32].
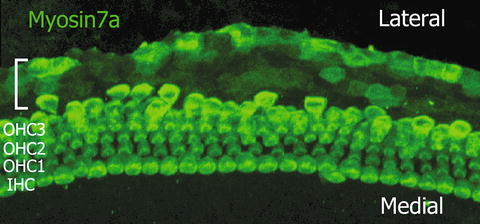
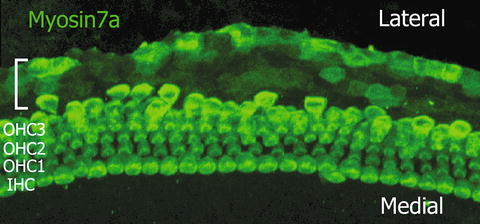
Fig. 21.2
γ-Secretase inhibitor induced ectopic hair cells. myosin7a (a hair cell marker) was visualized in green. Ectopic hair cells were observed (bracket) lateral to one row of inner hair cells (IHC) and three rows of outer hair cells (OHC1-3).
21.2.3 Future Directions for Transdifferentiation-Mediated Hair Cell Regeneration
Currently, only a single factor has been manipulated to induce transdifferentiation of hair cells in the cochlea, and the induced hair cells are not identical to physiological hair cells. This indicates that a single factor is insufficient and that several factors should be combined to achieve transdifferentiation of hair cells. To achieve more complete hair cell regeneration, it will be important to find a minimum but promising set of reprogramming factors. Atoh1 and inhibition of Notch signaling will be candidates to achieve this. Proper estimation of induced hair cells is also necessary to determine the combination required for successful transdifferentiation. To evaluate the transdifferentiation of hair cells properly, it is important to delineate the key characteristics that define hair cells, which include gene expression profiles, cellular organization of structural proteins, morphology, epigenetic marks, and functional attributes such as resting membrane potential and mechano-electrical transduction.
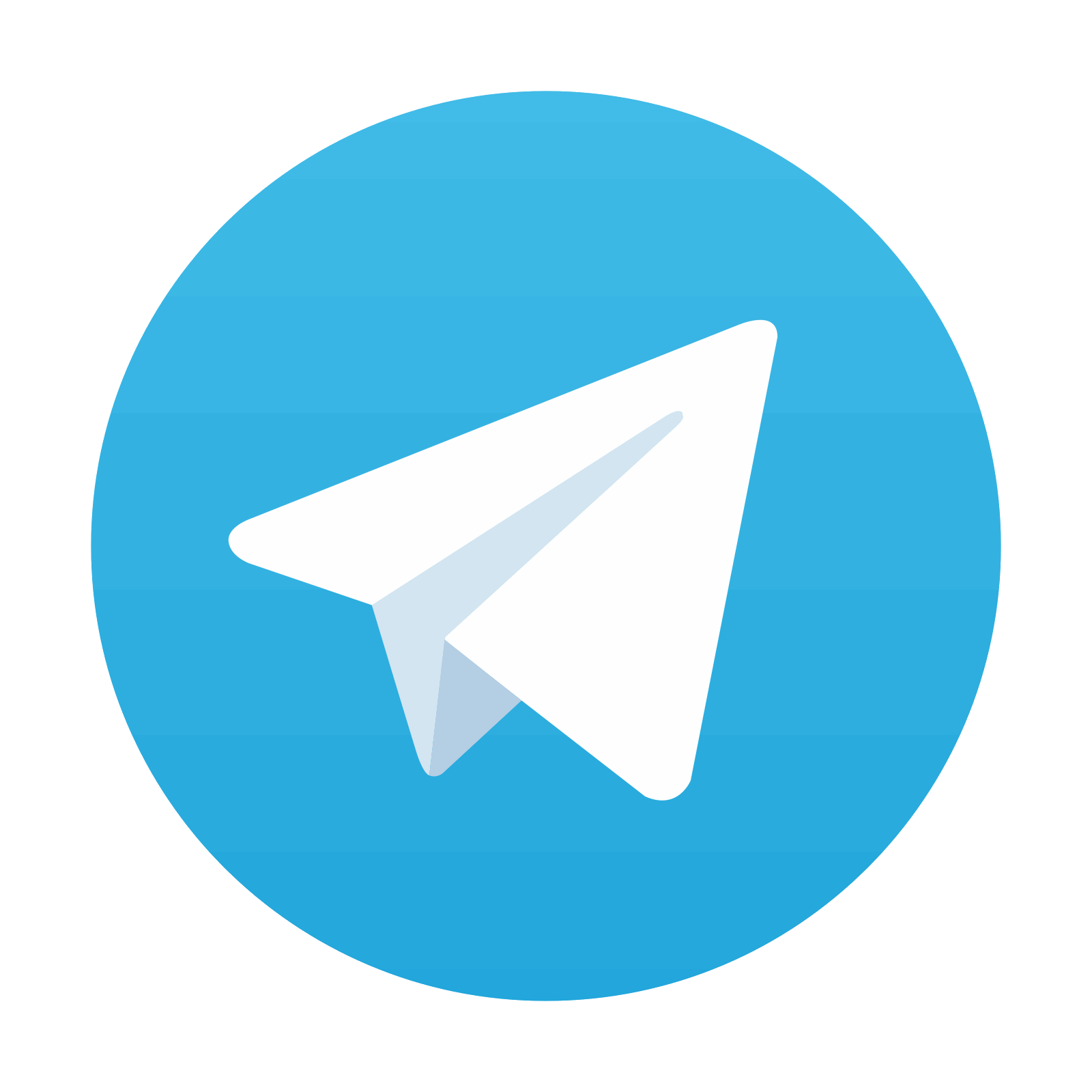
Stay updated, free articles. Join our Telegram channel

Full access? Get Clinical Tree
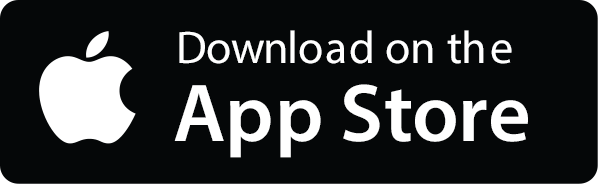
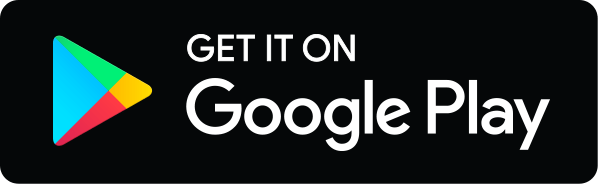