Fig. 3.1
Inner ear and sensory epithelia. (a) Schematic representation of the inner ear (orange) and its sensory epithelia (blue). There are six sensory epithelia in the inner ear. (b) Paint filling of E17.5 mouse inner ear
Hair cells are named after their hair bundles (stereocilia and kinocilium) on their apical side. Once the stereocilia deflect toward a specific direction, cation channels called MET channels open to induce the influx of potassium ion, causing the depolarization of hair cells [1]. The structure that mechanically opens MET channels was identified in the stereocilia [2] and is called as tip-link. Hence, the presence of MET channel in the stereocilia is widely recognized. The depolarization of hair cells results in the opening of the voltage-gated calcium ion channels and the influx of calcium ion induces the release of neurotransmitter, glutamate [3, 4], from the basal end of hair cells to cochlear and vestibular nerves that innervate cochlear and vestibular hair cells, respectively. Molecular analyses of the important structures in hair cells are in progress. Especially, the molecular components of MET channels have been investigated for a long time by several researchers. The candidate molecules that constitute MET channels were identified as transmembrane channel-like gene family 1 (Tmc1) and transmembrane channel-like gene family 2 (Tmc2) [5]. The molecular components that constitute the tip-link were determined as cadherin 23 and protocadherin 15 [6–8]. The genes for Tmc1, cadherin 23, and protocadherin 15 are causative genes of hereditary hearing loss, which indicate the importance of hair cells in hearing.
3.2 Characteristics of Each Hair Cell Type
There are two different types of hair cells in the cochlea (inner and outer hair cells) and in the vestibular organs (type I and II hair cells), respectively. Inner and outer hair cells are different in their position, ultrastructure, arrangement of stereocilia, innervation, and function. In the organ of Corti, inner hair cells (IHCs) are arranged in a single row adjacent to the modiolus. In contrast, outer hair cells (OHCs) are lined in three rows near the lateral wall (Fig. 3.2). IHCs are goblet-shaped (Fig. 3.3a) and their stereocilia are arranged in flattened U shape (Fig. 3.2). OHCs are long and cylindrical in their shape (Fig. 3.3a) and have stereocilia arranged in a W or V shape (Fig. 3.2). The innervation of IHCs is unique because IHCs are innervated mostly by afferent fibers that occupy 95 % of the cochlear afferent fibers [9]. Efferent fibers toward IHCs mostly make synapses with afferent fibers of cochlear nerve that innervate IHCs. OHCs are innervated by enormous efferent fibers and have only a few afferent fibers. The difference in the innervation pattern of IHCs and OHCs reflects the difference in the functions between both hair cells. IHCs mainly transduce auditory stimuli to the cochlear nerve as sensory end organs. In contrast, the depolarization and hyperpolarization of OHCs alter the length of the body of the OHC [10] rather than causing afferent nerve stimulation. This motility of OHCs contributes to the amplified vibration of the basilar membrane where IHCs and OHCs reside, resulting in the high sensitivity and frequency selectivity of hearing. Efferent stimuli from superior olivary complex to OHCs inhibit the electromotility of OHCs providing inhibitory modulation of the hearing [11]. The electromotility of OHCs is dependent on a novel motor protein, prestin [12]. Genetic disruption of prestin adversely affects the auditory brainstem response, distortion product of otoacoustic emissions, and cochlear microphonics in vivo as well as the electromotility of OHCs itself in vitro [13]. This, in turn, suggests the significance of OHC electromotility in the hearing of mammals.
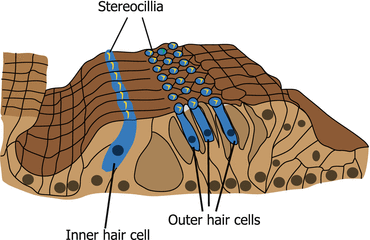
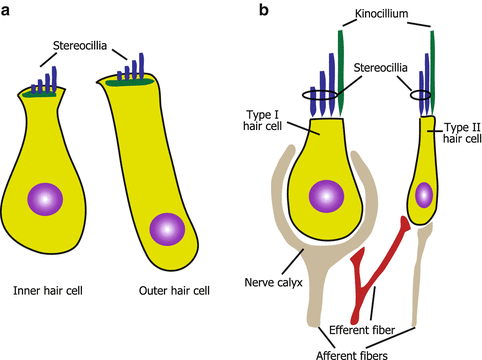
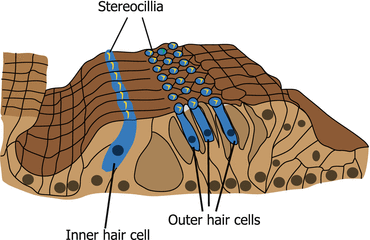
Fig. 3.2
Organ of Corti. Inner and outer hair cells are arranged in one and three rows, respectively. The stereocilia of inner and outer hair cells are arranged in U and V shapes, respectively
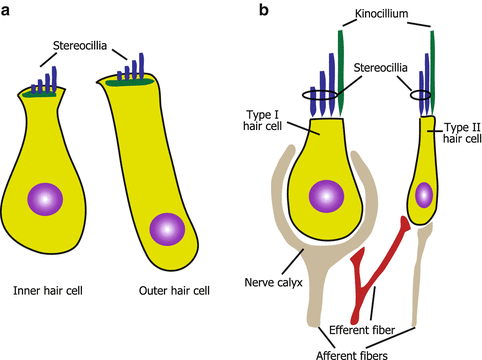
Fig. 3.3
Morphology of hair cells. (a) Cochlear hair cells. (b) Vestibular hair cells
Vestibular hair cells are morphologically similar to cochlear hair cells. However, vestibular hair cells have kinocilia (Fig. 3.3b) even at the postnatal stage, whereas cochlear hair cells lose kinocilia. Vestibular organs have two types of hair cells, namely, type I and II hair cells. Type I hair cells are flask-shaped and type II hair cells are cylindrical (Fig. 3.3b). Another morphological difference between these two hair cells is the difference in the innervation patterns of afferent fibers [14]. Type II hair cells have button endings of afferent fibers that is also seen in cochlear hair cells (Fig. 3.3b). In contrast, type I hair cells have calyx endings, which surrounds almost the entire basolateral surface of type I hair cells (Fig. 3.3b). The calyx-type afferent fibers have lower gains from head rotations compared to button-type afferent fibers [15], which reflect the functional difference between type I and II hair cells in the vestibular organs.
To distinguish cochlear and vestibular hair cells, which include the inner and outer hair cells, and type I and II hair cells, by using a molecular approach is still challenging. However, prestin is an OHC-specific motor protein and it is not expressed in other types of hair cells [12]. Considering that partial inhibition of Hedgehog signaling causes ectopic vestibular hair cell-like cells in the cochlea [16], this signal pathway may have important roles in the differentiation of cochlear hair cells.
Both cochlear and vestibular hair cells are surrounded and mechanically supported by a number of supporting cells. In the cochlea, some supporting cells form rigid scaffolding surrounding the hair cells. Supporting cells separate the apical surface of IHCs and the entire cell bodies of OHCs from adjacent hair cells.
High-potassium environment must be established and maintained to induce depolarization of hair cells subsequent to the opening of MET channels. To maintain this environment, tight junctions are used in the cochlea. Tight junctions are present at the apical circumference of epithelial cells, and they effectively seal all cells within an epithelium together. In the cochlea, extensive tight junctions are formed between the apical surface of hair cells and supporting cells so that potassium ion can be kept from the basolateral sides of the hair cells. The molecular components of tight junctions in the cochlea included various types of claudins [17], some of which are causative molecule of hereditary hearing loss.
3.3 Arrangement and Planar Cell Polarity
As already described, cochlear hair cells are arranged as a single row of IHCs and three rows of OHCs. Once the arrangement is disrupted, hearing function of the cochlea is lost [16]. The organization of the hair cells in a precise arrangement is dependent on several molecular mechanisms. One of the mechanisms is Notch signaling [18, 19] that controls the cell fate determination in various organs. When Notch signaling is inhibited genetically [18, 19] or pharmacologically [20], the number of IHCs and OHCs increased at the expense of the number of supporting cells. This indicates that the strict cell fate determination between hair and supporting cells by Notch signaling determinates the arrangement of IHCs and OHCs. The arrangement of cochlear hair cells is also controlled by the elongation force of the cochlea. When extension of the cochlea is inhibited by pharmacological or genetic disruption of non-muscle myosin II, which controls the convergence and extensions of organs, the arrangement of cochlear hair cells is severely disrupted [21].
In addition to hair cell arrangement, the polarity of hair cells is tightly controlled. One example of this highly regulated process is the arrangement of stereocilia. The direction of the stereocilia is always stable and the vertex of the U or W is always directed toward the lateral walls (abneural direction) of the cochlea. The regulation of such polarity within a two-dimensional surface is called planar cell polarity (PCP). PCP was originally investigated using the orientation of the hair on the cuticle of Drosophila and several genes were identified to be involved in PCP. Disruption of PCP-related gene homolog in mammals induced disorientation of stereocilia [22].
3.4 Regeneration of Hair Cells
As described in this chapter, hair cells are highly differentiated and, as a result, they have many specialized characteristics in their function, morphology, and innervation. As a target of regenerative medicine, hair cells are challenging cells because of these highly differentiated characteristics. It is easy to characterize mature hair cells by their specific morphology, physiological characteristics, or several specific markers. However, to regenerate functional hair cells after their injury, supporting cells and innervation from afferent and efferent fibers as well as hair cells themselves must be regenerated and integrated as a functional organ. Moreover, in 1967, Ruben reported that mammalian cochlear hair cells stop their proliferation during developmental stages [23]. This suggests that mammalian cochlear hair cells never regenerate physiologically after birth because of the lack of stem cell population that usually proliferates slowly. It is important to describe how hair cells regenerate in the avian species that can regenerate hair cells continuously in the vestibular organs and after injury in the auditory organs. In the regeneration of avian hair cells, two mechanisms occur and supporting cells are the source of the regenerated hair cells in both mechanisms. One mechanism involves the proliferation of supporting cells [24, 25] and the other involves the transdifferentiation from supporting cells [26].
To overcome the limited regenerative ability of mammalian hair cells, induction of proliferation and/or transdifferentiation in the mammalian inner ears or transplantation of stem cells that are able to transform to any type of cells in the body is necessary. Induction of proliferation and transdifferentiation may result in the efficient regeneration of hair cells because this approach does not require the in vitro preparation of stem cells that sometimes take a long time. Both induction of proliferation and transdifferentiation have already been tried in neonatal mice. In these studies, growth factors or cell signaling pathways that play pivotal roles in the development of hair cells were used or manipulated. The induction of proliferation to repair mammalian hair cells was achieved with insulin-like growth factor 1 (IGF-1) [27]. Inhibition of Notch signaling with either genetic or pharmacological methods induced transdifferentiation of supporting cells into hair cells in the mammalian cochlea [28]. Although both experiments used neonatal cochlear organotypic cultures, these results suggest the possibility of hair cell regeneration even in the postnatal mammalian inner ears.
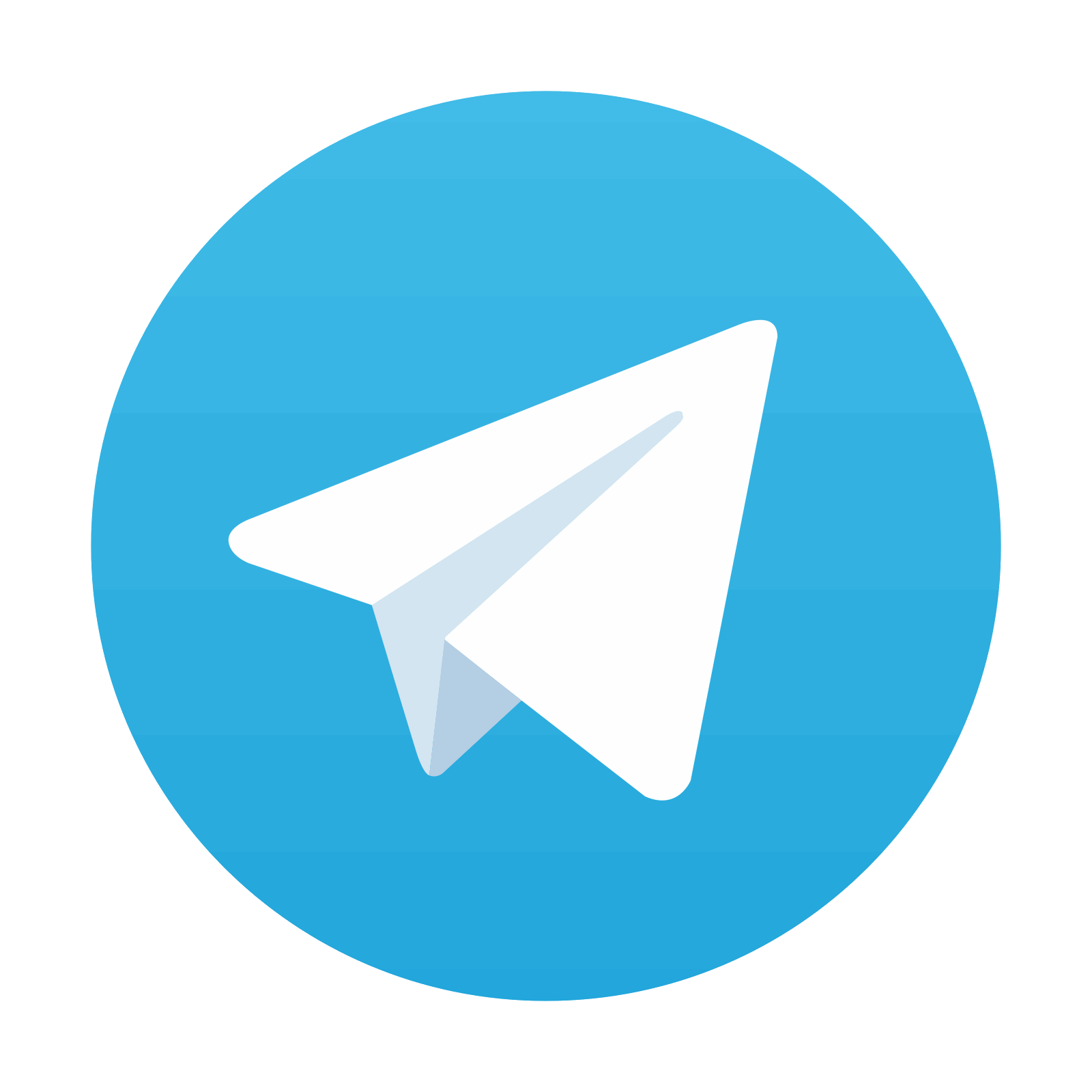
Stay updated, free articles. Join our Telegram channel

Full access? Get Clinical Tree
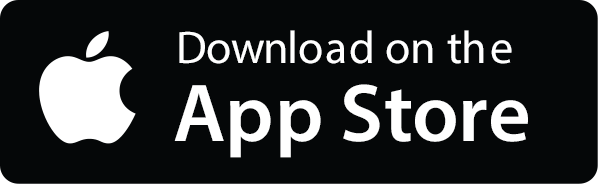
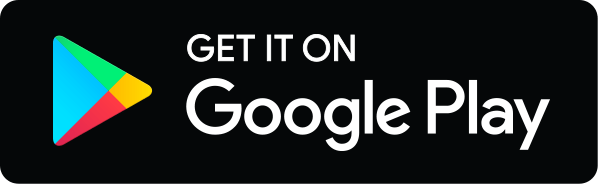