(1)
University of Sydney, Sydney, Australia
Overview (Fig. 12.1)
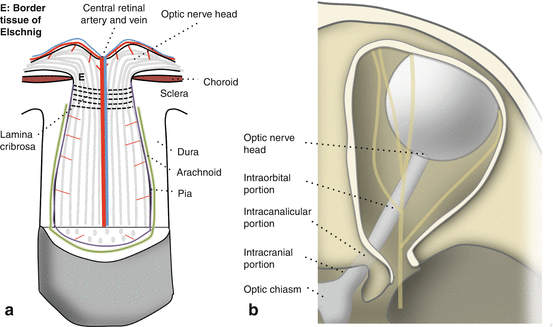
Fig. 12.1
The optic nerve. (a) Structure. (b) Divisions
-
The optic nerve is a central nervous system (CNS) white matter tract that transmits visual information from the eye to the brain.
-
The optic nerve consists of:(a)Retinal ganglion cell (RGC) axons(b)Supportive glial tissue(c)Vascular tissue
-
It is surrounded by three layers of meningeal tissue (pia, arachnoid, and dura).
-
The RGC axons:(a)Course along the retinal nerve fiber layer (RNFL) to enter the optic disc(b)Continue through the intraorbital, intracanalicular, and intracranial portions of the optic nerve(c)Pass through the optic chiasm and optic tract toward the CNS
-
The optic nerve has a limited capacity for regeneration after significant damage, resulting in irreversible visual loss.
Optic Nerve Divisions (Fig. 12.1b)
1.
2.
Optic nerve head (Fig. 12.2a) [4]
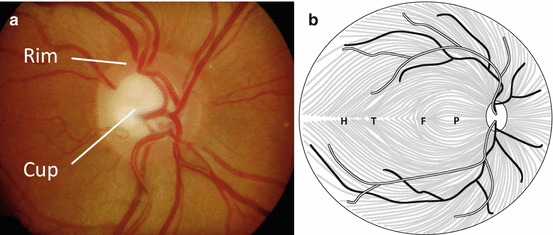
Fig. 12.2
(a) The optic nerve head. (b) Topographical organization of the retinal nerve fiber layer
-
The optic nerve head (optic disc) is located within the eye, consisting of a rim and a cup.
-
RGC axons from the RNFL turn 90° to dive into the disc to form the neuroretinal rim.
-
The RGC axons exit the eye through pores of the lamina cribrosa, a perforated portion of sclera that provides structural support to the optic nerve head.
3.
Intraorbital portion
-
The intraorbital portion courses through the orbit from the lamina cribrosa toward the optic canal.
-
It has redundancy in length to prevent globe tethering on eye movement and proptosis.
-
However, severe proptosis may result in optic nerve stretch and injury [5].
4.
Intracanalicular portion [5]
-
The optic nerve passes through the optic canal in the sphenoid bone and enters the cranial cavity.
5.
Intracranial portion
-
The intracranial optic nerve travels upward, posteriorly and medially.
-
It meets the contralateral nerve at the optic chiasm.
Topographic Organization of the Optic Nerve
1.
Retinal nerve fiber layer [7] (Fig. 12.2b)
-
Superior and inferior fibers are segregated by the horizontal raphe (H) which divides the inferior and superior visual fields.
-
Temporal macular fibers (T) course around the fovea (F) to enter the disc superiorly or inferiorly.
-
Nasal macular fibers travel in the papillomacular bundle (P) to enter the temporal aspect of the optic disc.
-
Fibers nasal to the optic disc enter nasally.
2.
Optic nerve head [8]
-
In the optic nerve head, peripheral retinal fibers are found peripherally and macular fibers centrally.
3.
Intraorbital, intracanalicular, and intracranial portions
-
From the intraorbital portion, the organization of fibers changes:(a)Temporal fibers gather temporally.(b)Nasal fibers gather in the nasal portion in preparation to cross at the chiasm.
Meningeal Layers Covering the Optic Nerve [5, 9]
-
The dura is a thick, tough fibrovascular tissue continuous with the CNS dura.
-
The arachnoid is a loose, thin, and vascular connective tissue.
-
The pia is very thin and tightly adherent to the optic nerve.
-
The pia extends septae into the nerve parenchyma that contain supportive blood vessels.
Central Nervous System Targets of Optic Nerve Projections (Fig. 12.3)
1.
Lateral geniculate nucleus (LGN) (90 % of all RGC axons)
2.
3.
4.
Pulvinar nucleus
5.
Hypothalamus
-
The hypothalamic suprachiasmatic nucleus receives RGC axons involved in the control of circadian rhythms [19].
Optic Nerve Parenchyma: Cellular Components
-
Glial cells provide structural and metabolic support for the RGC axons.
1.
Oligodendrocytes (Fig. 12.4)
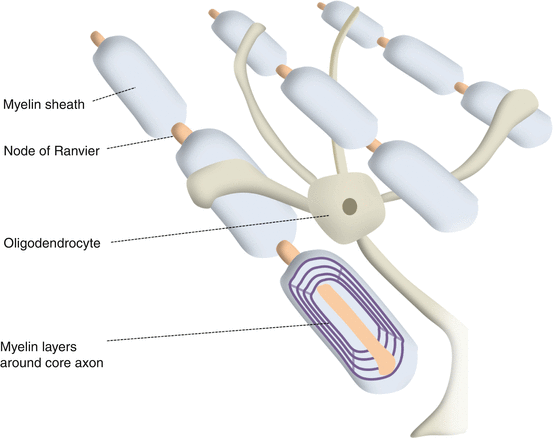
Fig. 12.4
Oligodendrocytes
-
Oligodendrocytes form a myelin sheath around axons posterior to the lamina cribrosa [21].
-
Myelin, a fatty multilaminated structure, provides electrochemical insulation to the axon [22].
-
Each oligodendrocyte has 20–30 processes that each myelinate a small portion of an axon.
-
Between each segment of myelin is the node of Ranvier.
-
The action potential jumps from one node to the other (saltatory conduction) to greatly increase the speed and efficiency of conduction [23].
2.
Oligodendrocyte origins and development
-
Oligodendrocytes are derived from oligodendrocyte precursor cells that migrate from the brain [24].
-
Their differentiation and renewal is controlled by neurotrophic factors including:(a)Platelet-derived growth factor (PDGF)
-
Myelination begins at 32 weeks gestation from the lateral geniculate nucleus.
-
Myelination progresses as far as the lamina cribrosa; the process is complete by 2 years of age [26].
3.
Astrocytes
-
Astrocytes are common CNS glial cells that express glial fibrillary acid protein [29].
-
They provide crucial supportive functions within the optic nerve, including:(i)Maintenance of water and electrolyte (especially K + ) homeostasis [30]
-
Electrolyte homeostasis is necessary for optimal electrical function of the axon.
-
K+ is released by axons on action potential repolarization.
-
Astrocytes absorb excess extracellular K+.
(ii)Metabolic supply to axons [25]-
Astrocytes store glycogen.
-
They can shuttle lactate to adjacent axons in ischemic or hypoglycemic states.
(iii)(iv)Response to optic nerve injury-
Astrocytes hypertrophy and extend cellular processes in response to parenchymal injury or loss, a process known as gliosis [32].
-
4.
Microglia [35]
-
These are bone marrow-derived phagocytic scavenger cells that resemble macrophages.
-
They move to sites of injury, proliferate, phagocytose, and degrade extracellular material.
-
They express cell surface antigen, stimulating T lymphocytes and activating the immune system.
Optic Nerve Axonal Physiology
1.
Retinal ganglion cell presynaptic input (see Chap. 8, The Retina).
-
Presynaptic input to RGCs is from bipolar (graded potentials) and amacrine cells (graded and action potentials).
-
Glutamate is the main excitatory neurotransmitter for RGC presynaptic input; it activates N-methyl-D-aspartate (NMDA) and non-NMDA ionotropic receptors, and metabotropic receptors [36].
-
Retinal Müller cells control extracellular levels of glutamate by active uptake via glutamate transporters and conversion to glutamine [37].
-
This prevents excessive glutamate-related excitotoxicity.
2.
Axonal conduction: action potential (Fig. 12.5, Table 12.1)
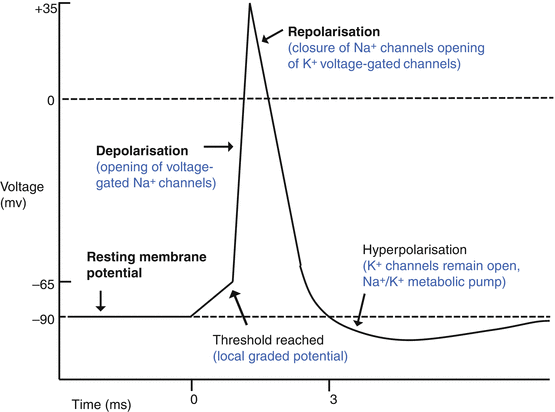
Fig. 12.5
The action potential
Component |
Explanation |
---|---|
Overview |
An action potential (AP) is an all-or-nothing phenomenon: the amount of voltage change (depolarization) is always the same |
Intracellular ionic concentration |
Axons have high K + and low Na + intracellular content compared to extracellular space |
AP generation – chemical events |
Synaptic input to the RGC cell results in membrane depolarization at the base of the axon, the axon hillock |
AP generation – electrical events |
Partial depolarization results in opening of voltage-sensitive Na + channels
An inflow of Na+ causes the membrane to depolarize |
AP propagation |
Increased potential rapidly affects adjacent sections of the axon
This causes adjacent Na + channels to open and the membrane to depolarize
This results in rapid transmission of the action potential down the axon |
Membrane repolarization |
This occurs due to opening of K + channels and gated closure of the Na + channels
The K + current dominates the membrane potential which hyperpolarizes
This occurs more slowly than depolarization |
Restoration of ionic equilibrium |
Transmembrane Na + /K + ATPase actively pumps Na+ out and K+ in. This restores ionic equilibrium and aids in restoration of resting membrane potential |
Saltatory conduction |
The myelin sheath increases the speed and efficiency of conduction
It insulates the axon from transmembrane leakage of charge
Only the exposed axonal membrane at the nodes of Ranvier depolarizes
The current passes from one node to another by saltatory conduction
This reduces the amount of ionic flux required to transmit action potentials |
3.
Axonal transport
-
This can be anterograde (cell body to axonal terminal) or retrograde (axon terminal to cell body).
-
Anterograde transport can be fast or slow.(i)(ii)
Optic Nerve Blood Vessels (See Chap. 11, Ocular Circulation)
-
Each section of the optic nerve has a unique blood supply (Table 12.2, Fig. 12.6).Table 12.2Blood supply to the optic nerve portions
Stay updated, free articles. Join our Telegram channel

Full access? Get Clinical Tree
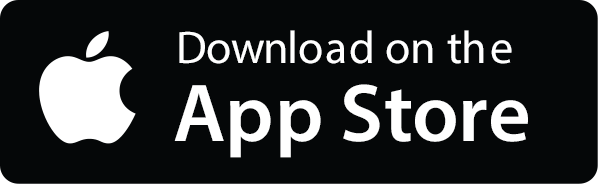
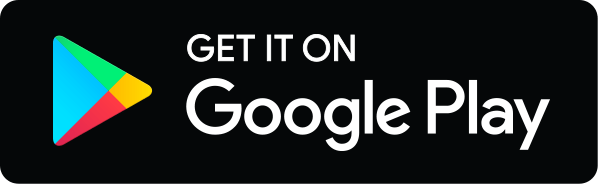