(1)
University of Sydney, Sydney, Australia
Overview
1.
Eye movements: functional domains and control systems
A diverse range of eye movements is required for the visual system to function optimally.
Oculomotor tasks can be grouped into four domains [1]:
(a)
Gaze stabalisation
(b)
Tracking a moving object
(c)
Exploring space
(d)
Maintaining binocular alignment
These tasks are achieved by six eye movement control systems (Table 18.1).
Eye movement control system
Outline
Functional domain
Stimulus
Conjugacy
1
Vestibulo-ocular reflex
Stabilizes gaze relative to changes in head position
Gaze stabilization
Change in head position
Conjugate
2
Optokinetic reflex
Maintains fixation on a moving target
Gaze stabilization
Full-field retinal slip
Conjugate
3
Position maintenance
Small ocular movements during steady gaze
Gaze stabilization
Microtremor, correction of ocular drift or fading image
Conjugate or non-conjugate
4
Saccades
Rapid eye movements to bring object of interest from the periphery to central gaze
Exploring space
Object of interest in the periphery
Conjugate
5
Smooth pursuit
Following a moving object
Tracking a moving object
Image slip from fovea
Conjugate
6
Vergence
Convergent or divergent movement to maintain motor fusion
Maintaining binocular alignment
Accommodation or diplopia induced by an approaching (or receding) object
Non-conjugate
All systems require three-dimensional control of eye position (vertical, torsional, and horizontal) about the x, y, and z axes of Fick (see Chap. 17. Movements of the Eye) [2, 3].
2.
Feedback and feed-forward control
Neural control of eye movements relies on feedback and feed-forward control systems:
(i)
Feedback
Feedback from retinal image motion, object displacement, or ocular rotation velocity is used to adjust motor responses to minimize subsequent errors [18].
3.
Hierarchy of oculomotor control
A hierarchy of neural control exists for each class of eye movement (Table 18.2).
Level of neural control
Anatomical substrate
Function
1
Cortical higher centers
Frontal and supplementary eye fields
Extrastriate and parietal cortex
Generation and planning of ocular movements.
Integration of movement planning with three-dimensional spatial maps constructed from visual sensory information
2
Subcortical areas
Superior colliculus, substantia nigra, cerebellum
Contribution to the temporal sequence of neural codes for controlling eye movements
The superior colliculus is involved in integrating sensory information for planning saccades and maintaining intersaccadic fixation
The cerebellum is involved in fine-tuning eye movements and long-term adaptation to improve future accuracy
3
Premotor nuclei (brainstem gaze centers)
Paramedian pontine reticular formation (PPRF)
Rostral interstitial medial longitudinal fasciculus (riMLF)
Interstitial nucleus of Cajal (INC)
Control and execution of horizontal (PPRF), vertical (riMLF), and torsional (INC) movements, respectively
Orchestration of the direction, amplitude, velocity, and duration of eye movements
4
Ocular motor nuclei
Cranial nerve nuclei III (oculomotor), IV (trochlear), and VI (abducens)
The final common pathway for eye movement control
5
Extraocular muscles
Superior, inferior, medial, and lateral recti
Superior and inferior oblique
Rotation of the globe
Force Generation for Extraocular Muscle Contraction (Fig. 18.1)
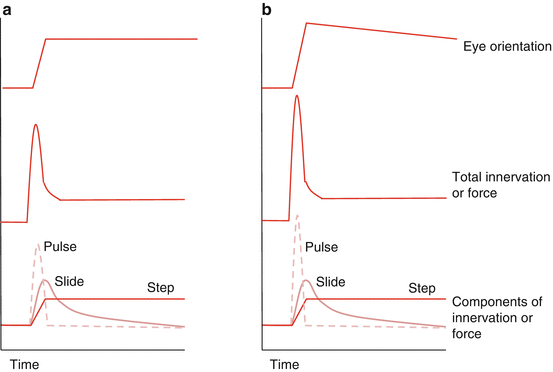
Fig. 18.1
(a). A pulse, slide, and step appropriately matched resulting in a successful saccade. (b) Mismatched signal (pulse too large) results in overshoot and drift back to the target (Based on Quaia, 2011) [43]
The neural signal required to generate an eye movement must:
(a)
Overcome the viscous properties of the orbit to move the globe to its new position, and
Pulse is related to the speed of EOM shortening; step is related to the functional EOM length.
The eye movement also contains a slide, which is intermediate between pulse and step.
The slide is adjustable and may help adapt for small pulse-step mismatches [41].
Premotor Nuclei
1.
Horizontal gaze center
Horizontal gaze is executed by:
The neural output from each must be equal to maintain Hering’s law.
Signal between the PPRF, abducens, and contralateral oculomotor nuclei is transmitted via the medial longitudinal fasciculus (MLF), a dorsal brainstem white matter tract [28].
Vestibular projections influence horizontal gaze through the vestibulo-ocular reflex.
2.
Vertical and torsional gaze centers
Vertical gaze is executed by the oculomotor and trochlear nuclei in the midbrain.
3.
Neural integrators
4.
Premotor nuclei cell types
Four types of neurons are involved in the premotor control of ocular movements.
(i)
(ii)
Burst neurons (long-lead, excitatory, and inhibitory subtypes)
Long-lead burst neurons receive input from the superior colliculus (SC) and frontal eye fields (FEF); they discharge 200 milliseconds before the saccade.
The excitatory burst neurons control saccadic duration and velocity [51].
Inhibitory burst neurons inhibit the antagonist muscles to the saccade [57].
(iii)
Ocular Motor Nuclei
The ocular motor nuclei give rise to the ocular motor nerves (cranial nerves 3, 4, and 6) [21].
These innervate the extraocular muscles to control eye position and movement (Table 18.3).
Cranial nerve
Cranial nerve nucleus
Brainstem location
Output muscle supply
3
Oculomotor
Oculomotor (skeletal muscle)
Edinger-Westphal (parasympathetic)
Midbrain, level of the superior colliculus
Levator palpebrae superioris
Superior rectus
Medial rectus
Inferior rectus
Inferior oblique
Sphincter pupillae (parasympathetic)
4
Trochlear
Trochlear
Midbrain, level of the inferior colliculus
Superior oblique
6
Abducens
Abducens
Pons
Lateral rectus
Each neuron projects to a group of extraocular muscle fibers, forming a motor unit.
All neurons contribute to all classes of eye movement.
More powerful motor units are progressively recruited as the eye moves into the EOM field of action [37, 58].
A neuron increases contractile force by increasing the frequency of spike potentials [44].
Eye Movement Control Systems (Table 18.1)
There are six systems of eye movement control that plan, coordinate, and execute motor activity.
1.
The vestibulo-ocular reflex (VOR)
The VOR generates eye movements to maintain eye position despite changes in head position [7].
The VOR uses signals generated in the vestibular apparatus, namely, the semicircular canals, utricle, and sacculus (Fig. 18.3).
Fig. 18.3
The vestibular apparatus
(i)
The angular VOR
The three semicircular canals allow the detection of rotation in multiple planes.
Signals from the semicircular canals cause eye rotation opposite in direction to head rotation [10].
(ii)
The linear VOR
The utricule and sacculus respond to head linear acceleration and tilt, generating the linear VOR.
The response is tonic involving the cyclo-vertical EOMs (superior and inferior oblique and recti) [60].
(iii)
Control pathways
The VOR is mediated by a 3-neuron arc involving the vestibular ganglion, vestibular nuclei, and ocular motor nuclei (Fig. 18.2) [61, 62].
The VOR has a short latency (7–15 msec) and is not under voluntary control; however, it can be dominated by optokinetic stimuli or reduced by steady fixation [10, 61].
(iv)
VOR-induced nystagmus
Large angle head rotations can exceed the ability of the VOR to maintain accurate fixation.
This results in a vestibular jerk nystagmus, characterized by:
Nystagmus can be induced by caloric testing; semicircular canals are stimulated by irrigating the ear with cold water, producing nystagmus with slow rotation towards the irrigated ear [68].
The slow-phase movements of nystagmus are identical to those induced by head movement [69].
2.
Optokinetic reflex (OKR)
This generates eye movements to maintain fixation in response to whole-field retinal image slip [8].
The OKR can be elicited by a persistently moving visual target or by head movement (causing a stationary image to move off the retina in the opposite direction).
(i)
(ii)
Control pathways
Visual information travels from the retina via the optic nerve and chiasm to specific nuclei in the pretectal area (e.g., the nucleus of the optic tract (NOT)) that respond to retinal image slip [71].
When stimulated these generate signal in the vestibular nuclei, resulting in an ocular motor response similar to the VOR (Fig. 18.4) [1, 72].
(iii)
Development of the optokinetic reflex pathways
3.
Position maintenance
They occur because it can be difficult to sustain fixed gaze, particularly in eccentric gaze, when there is a tendency towards drifts to the center [77].
These movements can be corrective for gaze direction and are probably important in preventing fading of image due to Troxler’s phenomenon (see Chap. 21. Visual Adaptation) [78, 79].
They include:
(i)
Tremor
This is a high-frequency, small-amplitude movement.
It possibly originates from asynchronous firing of motor units [1].
(ii)
Slow irregular drifts
These are long, slow, non-conjugate drifts in eye position.
(iii)
Microsaccades
4.
Saccades
Get Clinical Tree app for offline access
Saccades are rapid, accurate, conjugate eye movements to keep an object of interest on the fovea [15, 16].
Saccades consist of a rapid movement controlled by pulse/slide signal, followed by steady fixation determined by step signal; these are precisely matched by the cerebellum [41, 54, 80, 81].
Functions include:
(a)
Redirection of the eye so the image of a peripheral object of interest is brought to the fovea
(b)
Return of gaze to remembered locationsStay updated, free articles. Join our Telegram channel
Full access? Get Clinical Tree
