13 The Normal Retina, Retinal Imaging and the Interpretation of Pathological Changes
THE NORMAL RETINA
EMBRYOLOGY
The overall adult arrangement of retinal layers is present by 5½ months’ gestation but retinal development is not uniform. For example, although photoreceptors first differentiate at the macula, this is soon overtaken by development in other retinal areas so that at birth the macula is the only area not to be developed fully—it is not completely developed until about 3–4 months after birth, when the baby starts to fixate.
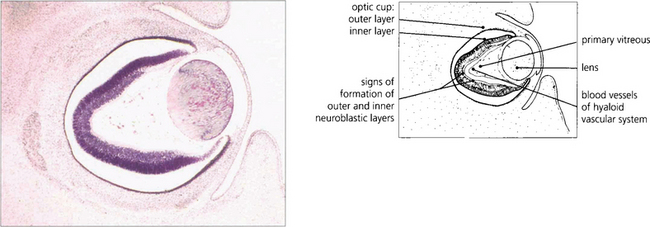
Fig. 13.1 An axial section of the eye of a 20-mm fetus (6 weeks’ gestation) shows the inner and outer layers of the optic cup; these will form the neurosensory retina and RPE respectively. The primary vitreous and hyaloid vascular system are visible, and the inner retina already shows signs of dividing into the inner and outer neuroblastic layers.
ANATOMY OF THE RETINA
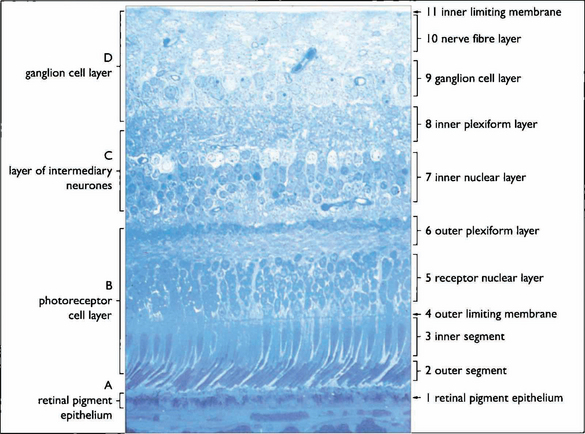
Fig. 13.3 The retinal cell layers are listed on the left and the apparent layers that these cells give rise to are shown on the right.
The retinal pigment epithelium
In younger eyes the border of the RPE cell adjacent to Bruch’s membrane is extremely convoluted and many mitochondria reside within this portion of the cell. These convolutions increase the surface area of the cell membrane which is covered with specific biochemical binding sites. RPE cells actively accumulate and transport metabolites diffusing through Bruch’s membrane from the underlying choriocapillaris and actively excrete waste products to the choriocapillaris (the retinal artery circulation does not contribute to the metabolic needs of the photoreceptors). Each RPE cell services up to 45 photoreceptors held in close physiological contact by membranous extensions from the surface of the RPE, termed ‘receptor sheaths’. These sheaths extend up to 50 per cent of the height of outer segments and play a major role in metabolite exchange between the cells. The extracellular space between the photoreceptors is filled with a glycosaminoglycans ground substance called the interphotoreceptor matrix. This differs in structure and chemical composition around rods and cones. There is no anatomical bond between the RPE cell and photoreceptor so that these two layers can be easily separated pathologically (e.g. by retinal detachment). However, the cone matrix sheaths are more strongly adherent to the RPE and resistant to the cone outer segment being withdrawn; this may explain the increased resistance of the macula to retinal detachment.
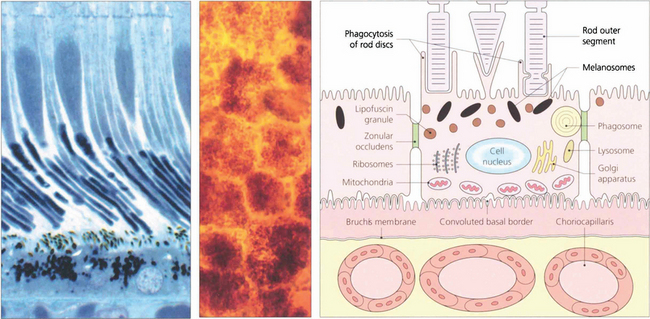
Fig. 13.5 A flat preparation shows the monolayer of hexagonal RPE cells and the natural colouration of their melanin granules. Tight junctions or zonular occludens between RPE cells prevent free diffusion from the choriocapillaris into the neural retina. These junctional complexes extend around the entire circumference of each RPE cell and bind it to its neighbours. The tight junctions and the active transport mechanisms together constitute the ‘outer blood–retinal barrier’ which ensures that photoreceptor cells are exposed only to selected molecules. The line drawing illustrates the intracellular anatomy. The complex of a pigment epithelial cell and its photoreceptors is a highly metabolically active area and specific failures in the metabolic route to and from the photoreceptor produce many of the inherited retinal dystrophies.
The photoreceptor cells
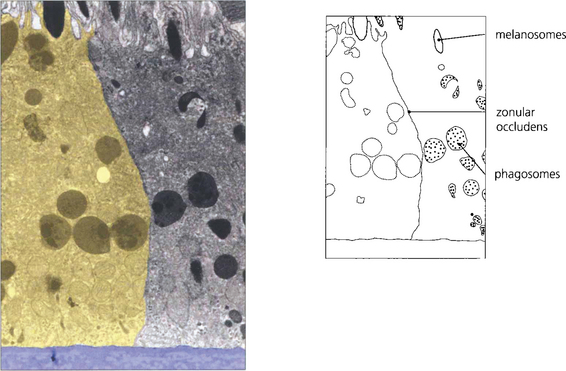
Fig. 13.6 The zonular occludens junction between RPE cells can be seen here. These surround the cell ensuring that the metabolites entering and leaving the retina from the choroid must pass intracellularly.
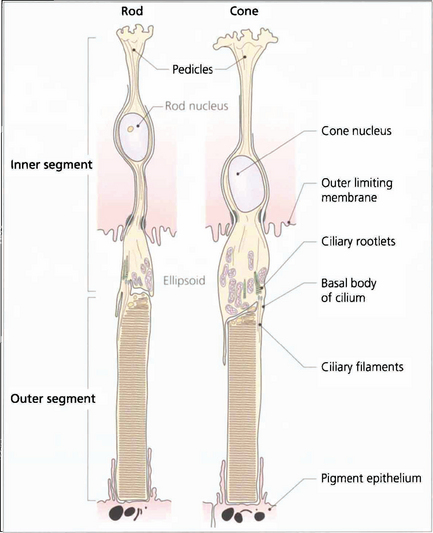
Fig. 13.7 The structure of a photoreceptor consists of:
• An outer segment, which is the light-sensitive portion of the cell and consists of a stack of hollow coin-like discs whose membranes contain the visual pigment molecules. In each rod there are about 1000 discs that are separate from all the others and the boundary membrane of the cell. Rod outer segments can be thought of as analogous to a stack of coins in a tube. If the tube is broken and the stack disturbed individual coins will be lost. In cones the outer segments differ in that all the ‘discs’ are joined to the boundary membrane with an aperture; through this hole the discs are in contact with the extracellular space and, therefore, with each other. Consequently, it is not possible to isolate a single ‘disc’ from a cone outer segment.
• A constricted region called the cilium which resembles the structure of other cilia in that it contains a number of paired microtubules.
• An inner segment which is the manufacturing portion of the cell; in each case this is divided into two, an outer ellipsoid and an inner myoid. The ellipsoid contains mitochondria and provides energy for the transduction processes in the outer segment, and the myoid contains Golgi bodies and ribosomes for manufacture of cell components and membranes.
• An outer connecting fibre that runs from the inner segment to the nucleus. In cones this tends to be short as the nuclei are situated in the outer part of the outer nuclear layer close to the outer limiting membrane. For rods the length varies with nuclear position.
• An inner connecting fibre that runs from the nucleus to the synaptic region. In cones it is this structure that becomes elongated as the fibres run out of the foveal pit to make contact with the displaced intermediary neurones and form the fibre layer of Henlé.
• A synaptic region. In rods this is sometimes called the rod spheral and in cones the cone pedicle. In both cells the synaptic region contains vesicles and mediation of transsynaptic information is by chemical transmitters. Both cells exhibit so-called invaginated connections with components from intermediary neurones deep to their synapse surface. These invaginated synapses are called ‘triads’ because they contain three processes, usually one from a bipolar cell and two from horizontal cells. Rods have a single triad whereas cones may have up to 20.
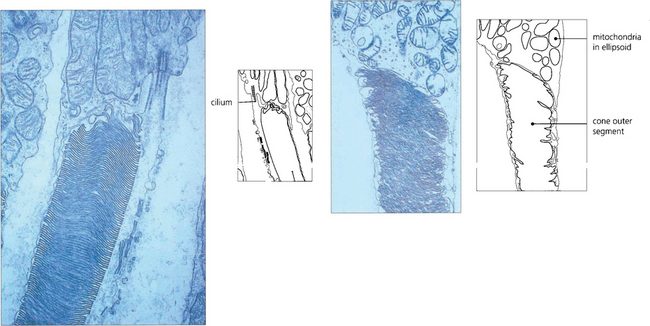
Fig. 13.8 Electron micrograph shows the junction between the inner and outer segment of a rod. Note the cilium. Photoreceptor discs are formed by invagination of the outer segment membrane. In contrast, cone outer segments remain attached to the cell membrane over a small part of their circumference.
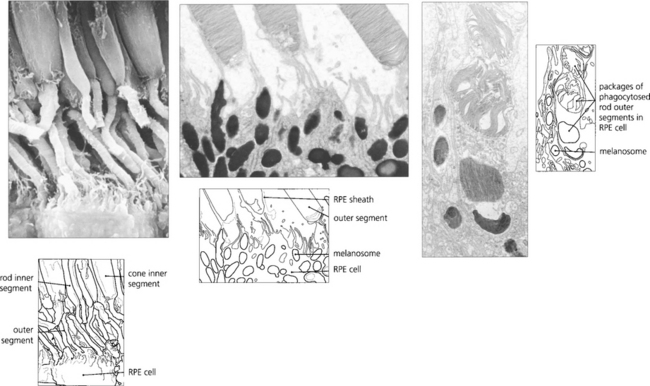
Fig. 13.9 Unlike neurones photoreceptor cells continually replace a major structural portion of themselves throughout life and again this process differs between rods and cones. In rods new discs are formed in the region of the cilium at the rate of about 1–5 an hour and as each new disc is formed older ones are progressively displaced towards the RPE. The oldest discs are shed from the tips of the outer segments in packets of about 30 at a time in a balanced process that does not radically alter rod length. Packets of rod discs are shed first thing in the morning or, if in periods of prolonged darkness, at the onset of light. The shed discs are phagocytosed by the RPE and, therefore, the rod outer segment is replaced entirely every 8–14 days. Less is known about cones although the evidence suggests that their discs are also renewed, however, the process is much slower than that for rods so that it takes about 9 months to 1 year for the outer segment to be replaced fully. This time course is supported by clinical observations on the recovery of cone function after retinal detachment surgery. In contrast to rods, cones shed their phagosomes at night. This circadian rhythm is one of many thought to be initiated by the effect of light absorption on the photopigment melanopsin which is located in some retinal ganglion cells.
Topographical variation in the retina
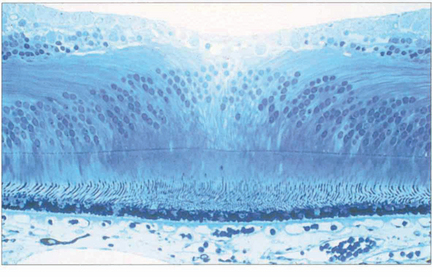
Fig. 13.11 In the foveola, the neural retina is about 170 mm thick, compared to 350 mm in the fovea. This thinning arises because the neurones of the inner retina, together with the ganglion cells, are displaced radially as the nerve fibre layer of Henlè; incident light can thus fall on the highly specialized cones without passing through a potentially light-scattering medium of neural retina and retinal capillaries.
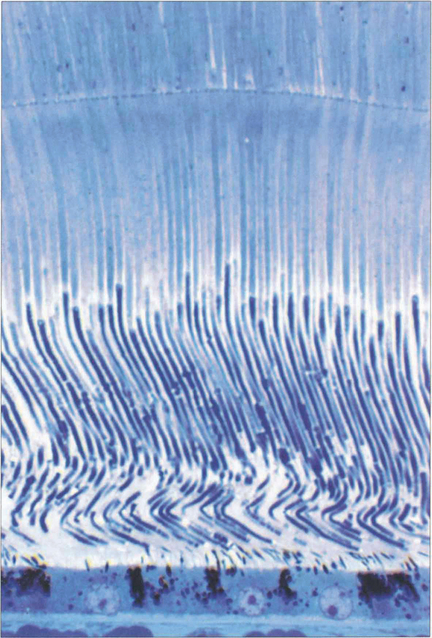
Fig. 13.12 At the fovea the cones have a tall slender shape with a diameter of 1.5μm permitting a resolving power of 20° of arc at the nodal point of the eye.
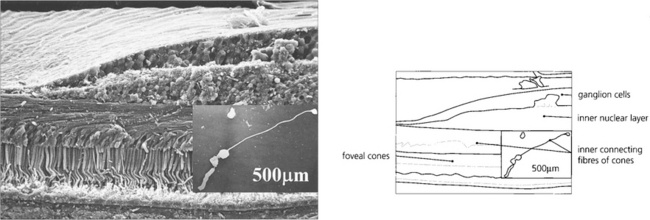
Fig. 13.13 For the foveal cones to connect with the displaced inner retinal neurones, the inner connecting fibres of the cones become very elongated and collectively form the fibre layer of Henlé. Macular xanthophil pigment is located in this layer and absorbs blue light, which is potentially harmful to the photoreceptors. Pathological oedema and exudation readily accumulate here because the inner connecting fibres are not held tightly together. This is seen clinically as the radially oriented cystoid spaces of macular oedema or as a macular star with lipid exudation.
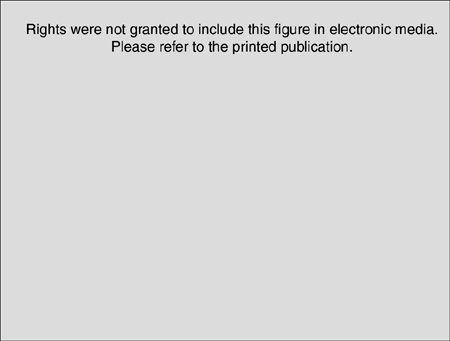
Fig. 13.14 (Left) At post-mortem examination, the macula has a yellowish colour from the xanthophil pigment (hence the name macula lutea). This pigment has a role in protecting the photoreceptors from short wavelength blue light which has the ability to produce cellular damage as a result of the generation of free radicals. (Right) Histological examination shows the pigment in Henlés layer.
Left image from Miller D (ed.) Clinical Light Damage to the Eye. New York: Springer, 1987.
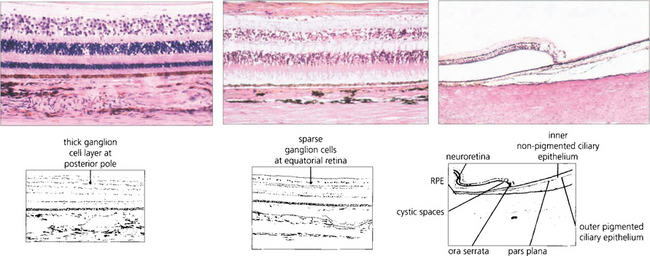
Fig. 13.15 In the posterior pole the ganglion cell layer is several cells thick. Towards the periphery the retina becomes thinner and the ganglion cell layer less dense. In the elderly the peripheral retina adjacent to the ora serrata may show cystic spaces within the attenuated neural retina. At the ora serrata the inner retinal layers are lost and the photoreceptors become shorter and fewer until finally the retina is lost to fuse with the nonpigmented monolayer of the pars plana which runs forwards over the ciliary processes (see also Ch. 9).
Retinal blood vessels
The central retinal artery supplies all the cells of the neural retina with the exception of the photoreceptors which receive their metabolic supply from the choroid by active transport through the RPE (see Ch. 9
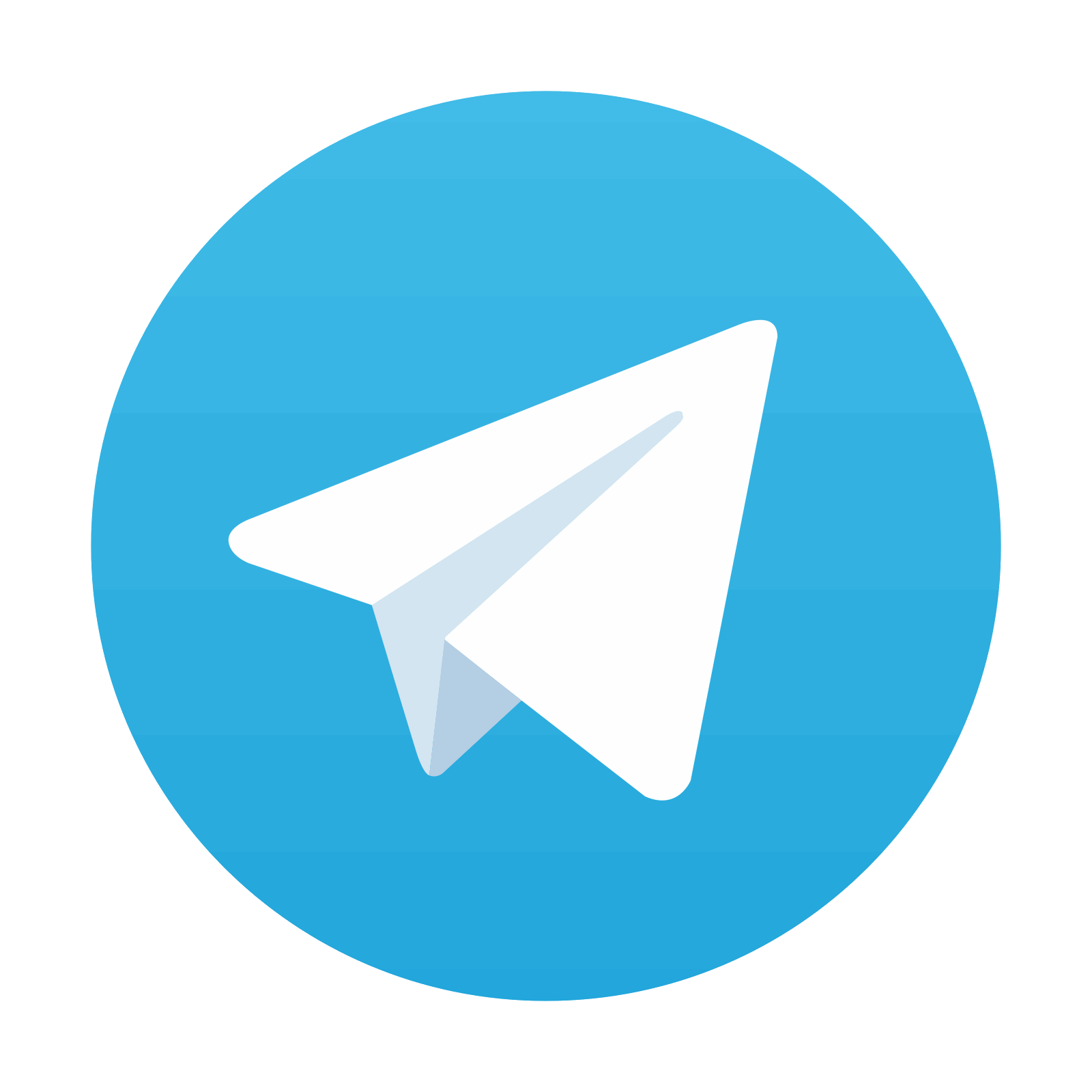
Stay updated, free articles. Join our Telegram channel

Full access? Get Clinical Tree
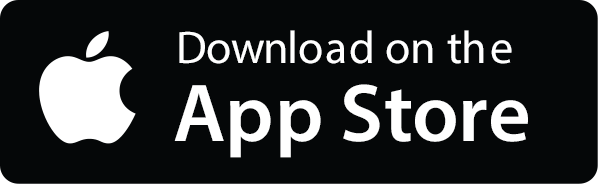
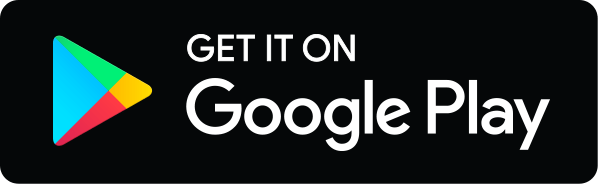