7 The Lens
The structure and embryology of the lens are discussed in Chapter 1.
CONGENITAL ANOMALIES OF THE LENS
The posterior surface of the lens in an infant’s eye often has a dimpled configuration called posterior umbilication (Fig. 7-1A). Posterior umbilication is an artifact caused by fixation and is not present in vivo.
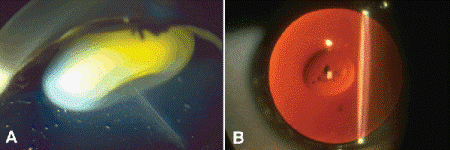
Fig. 7-1. A. Posterior umbilication, infant eye. The concave shape of the posterior lens is called posterior umbilication. Posterior umbilication is a fixation artifact that affects the lens in infants. The hyaloid artery persists in this specimen. B. Posterior lenticonus. Posterior lenticonus appears as “oil droplet” in retroillumination. Anomaly occurred unilaterally in healthy patient.
The surface of the lens has a conical configuration in lenticonus. Lenticonus can be anterior or posterior, and probably is caused by focal thinning of the lens capsule. Anterior lenticonus usually is bilateral, and may be associated with Alport syndrome of hereditary hemorrhagic nephritis and deafness, which is caused by mutations in several genes for Type IV or basement membrane collagen. The lens capsule in Alport syndrome is thin and has linear dehiscences. The renal disease presumably is caused by analogous abnormalities in the glomerular basement membrane. Many cases of Alport syndrome are caused by mutations in the COL4A5 gene on the X chromosome and show X-linked inheritance.
Posterior lenticonus usually is a unilateral sporadic condition that is not associated with other ocular or systemic disease. When the lens is retro-illuminated, the lenticonus often appears as an “oil droplet” (Fig. 7-1B).
Lens coloboma (lens notching) is a secondary phenomenon caused by a focal absence of zonular fibers in a contiguous coloboma of the ciliary body. Rarely, lens coloboma can herald the presence of a pediatric ciliary body neoplasm, usually a medulloepithelioma.
Congenital or developmental cataracts (Fig. 7-2) occur in isolation or in association with systemic or ocular anomalies. Approximately one third of congenital cataracts are inherited, one third are idiopathic, and the remaining third have systemic associations. Most hereditary cataracts are inherited in an autosomal dominant fashion with high penetrance. At least 39 loci for isolated or primary congenital cataracts have been identified. These include genes for a variety of lens crystallins, gap junction and membrane proteins, and growth and transcription factors.
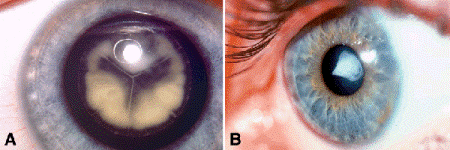
Fig. 7-2. Developmental cataracts. A. Congenital opacity involves embryonic nucleus of lens, which is delimited by “Y” sutures. B. Anterior pyramidal cataract. Cone of collagenous connective tissue projects from anterior surface of lens. Anterior pyramidal cataract is a development variant of anterior subcapsular cataract.
Developmental cataracts can affect different parts of the lens. Anterior pyramidal cataract (Fig. 7-2B) is a congenital form of anterior subcapsular cataract. The opacity beneath the anterior lens capsule is a white plaque of dense collagen.
A spectrum of opacities that involve the posterior pole of the lens is related to faulty resorption of the embryonic hyaloid vascular system that nourishes the developing lens in utero. An innocuous spot called a Mittendorf dot marks the site where the hyaloid artery was attached to the posterior lens capsule. Mittendorf is found in about 25% of normal individuals and is best seen on retroillumination. A patent hyaloid artery may persist in some adults. Posterior remnants of the hyaloid artery and Bergmeister papilla may be evident as vascular loops and glial veils on the optic disc.
The most severe condition related to incomplete resorption of the embryonic vasculature is persistent hyperplastic primary vitreous (PHPV) or persistent fetal vasculature (PFV), a congenital anomaly that often is found in microophthalmic eyes (Fig. 12-17). PHPV/PFV is characterized by a retrolental plaque of vascularized connective tissue that is thought to represent a residuum of the embryonic primary vitreous. The tips of the ciliary processes are attached to the margins of this retrolental plaque. As the eye enlarges, the ciliary processes are drawn centrally and stretched, and may be seen clinically when the pupil is dilated. PHPV/PFV produces leukocoria (a white pupil), and is an important lesion in the differential diagnosis of the childhood retinal malignancy retinoblastoma. Goldberg applied the term PFV to this syndrome to emphasize the involvement of other fetal ocular vessels such as iris shunts.
Zonular cataracts are marked by the opacification of a single zone of lens fibers. Lamellar cataract is a form of zonular cataract that results when a group of lens fibers are opacified at one point during development and subsequently are buried by the formation of new, healthy clear cortex. Damage to developing lens fibers during an attack of neonatal tetany is a classic cause of lamellar cataract. Analogous to tree rings in chronodendrology, the opacified fibers serve as a clinical marker that allows one to roughly estimate when an insult occurred during lens development.
Cataracts occur in patients who have a bewildering number of genetic syndromes, genetic diseases, and developmental disorders. A search for cataract on the Online Mendelian Inheritance in Man (OMIM) Web site in 2010 retrieved 364 separate items. Rare syndromic associations include chondrodysplasia punctata and the Hallermann-Streiff, Nance-Horan, Rothmund-Thomson, Marinesco-Sjogren, and hyperferritinemia-cataract syndromes.
Congenital cataract and glaucoma occur together in patients who have Lowe syndrome, an X-linked oculocerebrorenal syndrome characterized by renal rickets and amino aciduria. The lens in Lowe syndrome is small and discoid in shape, and may have lens capsular incresences. Obligate female carriers of Lowe syndrome may have punctate opacities and plaque-like posterior subcapsular cataracts. Thirteen percent of patients with Down syndrome (trisomy 21) have cataracts.
The clinical triad that comprises Gregg syndrome of rubella embryopathy includes cataract, deafness, and cardiac anomalies such as patent ductus arteriosus. Rubella cataracts are typically dense, pearly-white and nuclear. Lens epithelial nuclei may persist for decades in the embryonic nucleus of a rubella cataract. Virus has been cultured from rubella cataracts several years after birth.
CATARACT
Cataract is opacification or optical dysfunction of the crystalline lens. Cataract is an extremely common and economically important cause of visual loss. Derived from the Greek word for waterfall, the term cataract probably refers to the white appearance of some senile cataracts that was likened to rapidly flowing “white water.”
Cataract is the end stage or final common pathway of lens pathology. Although lens opacification often occurs as the result of aging, a host of other factors can cause cataracts, including trauma, drugs, toxins, radiation, inborn errors of metabolism, and concurrent ocular or systemic disease.
Cataract is essentially a clinical diagnosis denoting loss of optical function. Visual function is best determined by the ophthalmologist during a clinical examination. In most institutions, cataracts are no longer submitted to pathology. It actually is difficult to diagnose cataract histopathologically in many cases, especially when modern surgical techniques of extracapsular lens extraction such as phacoemulsification have been used. After the contents of the optically dysfunctional lens have been removed, a prosthetic intraocular lens (IOL) is inserted in the lens capsular bag. The “IOL” obviates the need for aphakic spectacles postoperatively (Fig. 7-3).
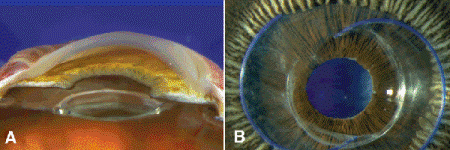
Fig. 7-3. Pseudophakia. A. Optic of posterior chamber IOL is seen within lens capsular bag in posterior chamber. Some residual lens cortical material is present. Eye was enucleated for uveal melanoma. B. Blue haptics of older three-piece posterior chamber IOL are confined to lens capsular bag. Iris pigment epithelium and ring of ciliary processes are evident.
Some degree of nuclear sclerosis (Fig. 7-4) develops in all individuals as they age. The inevitability of nuclear sclerotic cataract is inherent in the lens’ normal pattern of growth and development. The lens is derived from surface ectoderm, and, like the skin, it grows continuously, albeit slowly, throughout life. Growth of the lens results from the continuous accretion of new secondary lens fibers around its circumference. The formation of the new lens fibers buries the older fibers, which are sequestered centrally in the lens nucleus. (Mature skin cells are desquamated.) With the passage of years, the older cells gradually degenerate. This is not surprising because mature lens cells are anucleate and lack the metabolic machinery for protein synthesis and repair. Eventually, the highly specialized lens proteins called crystallins become denatured, and the cytoplasm of the lens fibers becomes increasingly dehydrated. The degenerative process is also marked by the accumulation of a yellow-brown pigment called urochrome that is probably related to photo-oxidation (Fig. 7-4B).
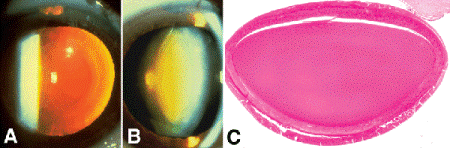
Fig. 7-4. Nuclear sclerosis. A. Sclerotic nucleus is evident as oil droplet in red reflex. Patient developed cataract after filtering surgery. B. Slit beam discloses yellow urochrome pigment in nucleus. C. Artifactitious clefts delimit boundary between the sclerotic lens nucleus and the cortex. The nucleus is more eosinophilic than the cortex and lacks the artifactitious clefts that normally form when the lens is sectioned. (H&E ×10)
Histopathologically, nuclear sclerosis is marked by increased eosinophilia and homogeneity of the lens nucleus, and an absence of the artifactitious cracks that normally occur between lens fibers during microtomy (Fig. 7-4C). As the nucleus becomes denser, its index of refraction, and hence refractive power, increase, causing lenticular myopia (grandma’s second sight). Presbyopic patients often find that they are able to read without their reading glasses, as they develop nuclear sclerosis and become progressively myopic. Nuclear sclerosis also distorts color vision because of the yellow-brown urochrome pigment, which accumulates in the lens and filters out blue wavelengths of light. After nuclear sclerotic cataracts are removed, patients may complain about blue vision. In advanced nuclear sclerosis, cataracts may be amber-colored (brunescent) or even black (cataracta nigra). Calcium oxalate crystals occasionally are found in lenses with nuclear sclerosis. These oval crystals exhibit vivid rainbow-like birefringence during polarization microscopy (Fig. 7-5).
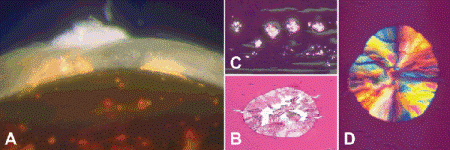
Fig. 7-5. Oxalate crystals, nuclear sclerosis. A. Oxalate crystals are evident as orange sperules in severely scerotic, brunescent nucleus. Peripheral degenerated cortex contains foci of calcification. B–D. Polarization microscopy highlights oval birefringent crystals of calcium oxalate in nuclear sclerotic cataract. The crystal in part D exhibits vivid rainbow-like pattern of birefringence. (A. H&E with crossed polarizers ×25, B. H&E ×100, C. H&E with crossed polarizers ×250)
Cortical cataract (or soft cataract) is marked by degeneration of the fiber cells comprising the lens cortex. The incipient stage of cortical cataract is marked clinically by the development of vacuoles or clefts containing clear watery fluid (water clefts). As the disease progresses, the foci of degenerated cortical material appear white in direct illumination and are seen as black shadows in retroillumination. The cortical opacities often begin near the equator, and may involve a wedge-shaped sector of cortex. Sparkling crystals of cholesterol and insoluble amino acids develop in some cases. The term Christmas tree cataract is applied to cataracts that contain many crystals.
Histopathologically, cortical degeneration is marked by clefts and spaces in the cortex filled with liquefied cortical material, which oozes from fractured lens fibers. Spherules of degenerated lens cytoplasm called morgagnian globules typically are seen (Fig. 7-6). Total cortical liquefaction occurs in morgagnian cataracts (Fig. 7-7). Morgagnian cataracts are typically swollen and intumescent because the osmotic effect of the degenerated cortical material causes the lens to imbibe aqueous humor. In some patients, the swollen lens may precipitate closed angle glaucoma (phacomorphic glaucoma). The sclerotic nucleus typically resists liquefaction and sinks inferiorly in the capsular bag of liquefied cortex (Fig. 7-7A). The milky, fluid cortex often contains crystals of cholesterol. The denatured lens protein can leak through the intact lens capsule in advanced cases and stimulate a bland macrophagic response. This may lead to a variety of secondary open angle glaucoma called phacolytic glaucoma, which is caused by obstruction of the trabecular meshwork by macrophages that have ingested lens material and free high molecular weight lens protein, as well (Figs. 3-6B and 8-15A,B). Dystrophic calcification is often found in the degenerated cortex of long-standing cataracts. Blind painful eyes occasionally contain totally calcified lenses that are rock-hard and cannot be sectioned without prior decalcification.
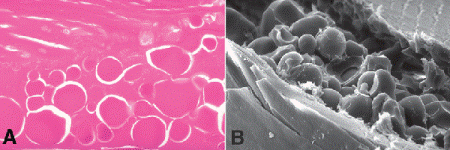
Fig. 7-6. Cortical cataract, morgagnian globules. A. Liquefied cortex and morgagnian globules fill cleft in cortical cataract. Morgagnian globules are spherules of degenerated lens protein that have leaked from fragmented lens fibers. B. Scanning electron micrograph of cortical cataract showing morgagian globules in cleft. (A. H&E ×100, B. SEM ×320)
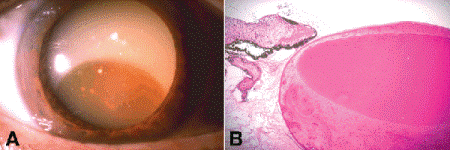
Fig. 7-7. Morgagnian cataract. A. Morgagnian cataract results when the lens cortex undergoes total liquefaction. The densely sclerotic nucleus is resistant to liquefaction and sinks to the bottom of the bag of liquefied cortex. Calcium oxalate crystals are evident as lighter spherules in the amber nucleus. (Photo courtesy of Prof. Dr. med. Wolfgang Lieb, University of Würzburg.) B. Degenerated lens cortex surrounds the intensely eosinophilic sclerotic nucleus. Although most of the cortex has liquefied, a few curved disrupted lens fibers are seen posteriorly. (H&E ×10)
The two other histologic subtypes of cataract, anterior subcapsular cataract and posterior subcapsular cataract are caused by abnormalities in the anterior lens epithelium. Anterior subcapsular cataract is marked histopathologically by a white plaque of dense collagenous connective tissue that forms beneath the anterior lens capsule (Fig. 7-8). Contraction of the fibrous tissue causes characteristic sinuous folds in the anterior capsule (Fig. 7-8B). The subcapsular collagen is synthesized by lens epithelial cells. The cells are found within the plaque surrounded by capsules of basement membrane material that evidence their lens epithelial lineage (Fig. 7-8C). In some instances, a second, delicate, new layer of lens capsule is found beneath the anterior subcapsular fibrous plaque. This is made by lens epithelial cells that have migrated beneath the plaque. Adhesions between the iris and lens (posterior synechiae) and/or anterior segment inflammation often stimulates the proliferation and fibrous transformation of the lens epithelium. Although anterior subcapsular cataracts frequently are found in blind painful eyes in the ophthalmic pathology laboratory, they are not observed clinically very often because they typically are obscured by posterior synechiae and pupillary membranes. Capsular fibrosis, which causes posterior capsular opacification and wrinkling after planned extracapsular cataract surgery, reflects an identical process of lens epithelial proliferation and fibrous transformation (Fig. 7-9A,B).
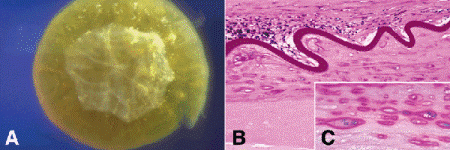
Fig. 7-8. Anterior subcapsular cataract. A. The irregular white opacity on the anterior surface of the lens is a plaque of collagen that has been synthesized by lens epithelial cells. The anterior lens capsule is folded and the lens nucleus is sclerotic. B. Sinuously folded anterior lens capsule covers anterior surface of fibrous plaque made by lens epithelial cells irritated by inflammatory membrane in posterior chamber. C. Lens epithelial cells within the plaque surrounded by capsules of PAS-positive basement membrane. (B. PAS ×100, C. PAS ×250)
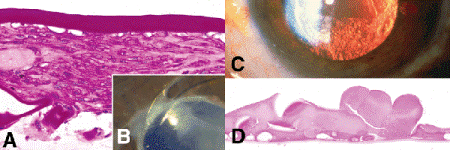
Fig. 7-9. A. Capsular fibrosis, post-extracapsular cataract extraction (post-ECCE). Lens capsular bag contains mass of collagenous connective tissue made by residual lens epithelial cells that reside in basement membrane capsules. Anterior capsule is above, posterior capsule below. B. Macrophoto in inset shows capsular folds and opacification near edge of IOL. C. Elschnig pearls, post-ECCE. Relucent spherules derived from residual lens epithelial cells are seen within lens capsule after extracapsular cataract extraction. D. Elschnig pearls are analogous to bladder cells found in posterior subcapsular cataract (PSC). Nuclei of Elschnig pearls on posterior capsule (below) are not evident in this plane of section. (A. PAS ×100, D. H&E ×100)
In posterior subcapsular cataract, lens epithelial cells migrate posteriorly beneath the lens capsule behind the lens equator where the monolayer of anterior lens epithelium normally terminates. Noxae such as inflammation or ciliary body tumors stimulate the abnormal epithelial migration. Situated aberrantly at the posterior pole of the lens, the lens epithelial cells attempt to form new secondary lens fibers, but abortive lens fibers called Wedl or bladder cells result (Fig. 7-10). Filled with lens protein, the Wedl cells are large and round or oval and have a single nucleus. Posterior subcapsular cataracts tend to interfere with near vision early because the opacity is located near the nodal point of the eye’s visual system. Patients with posterior subcapsular cataract also are prone to develop severe glare symptoms, especially during night driving. The nuclei of bladder or Wedl cells serve to distinguish them from morgagnian globules, which are round anucleate spherules of degenerated lens protein.
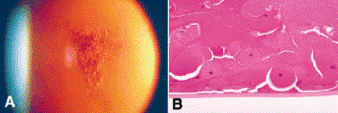
Fig. 7-10. Posterior subcapsular cataract. A. Posterior subcapsular opacity disclosed by retroillumination comprises relucent spherules consistent with bladder cells. B. Wedl or bladder cells represent abortive attempts by lens epithelial cells to form new lens fibers. They develop from aberrantly situated lens epithelial cells that have migrated posteriorly. Several of the bladder cells contain nuclei. The nuclei in some of the other large cells are not present in this plane of section. (B. H&E ×100)
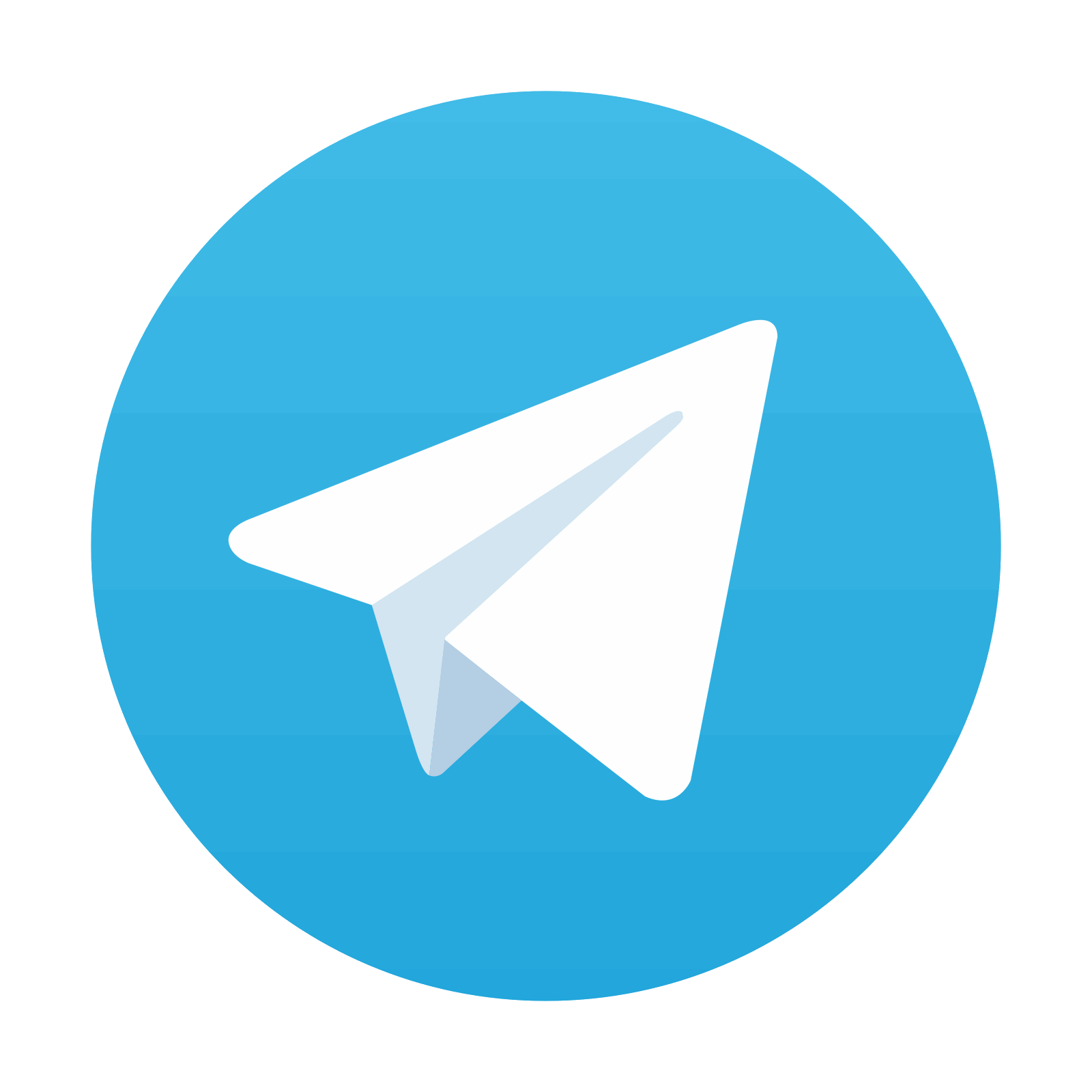
Stay updated, free articles. Join our Telegram channel

Full access? Get Clinical Tree
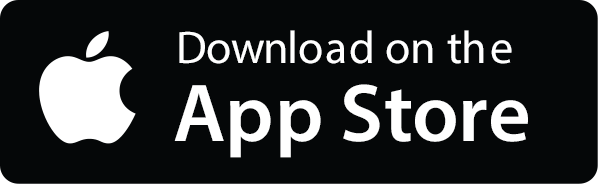
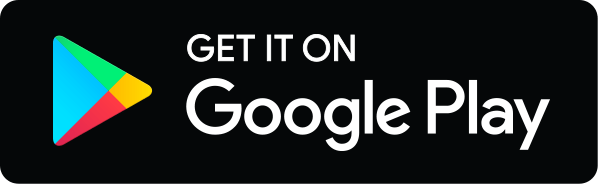