11 The Lens
DEVELOPMENT AND GROWTH OF THE LENS
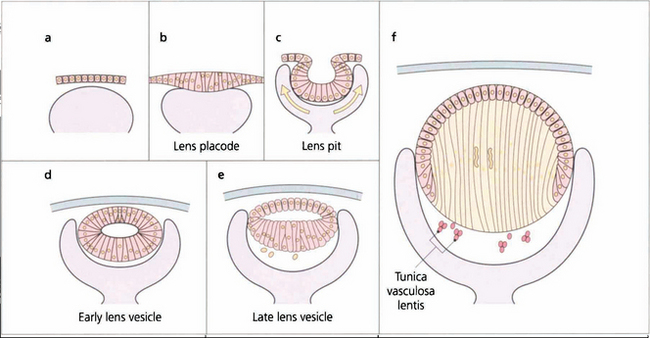
Fig. 11.1 Recently there has been considerable research on the genetic control of lens development and on the growth factors such as fibroblast growth factor and insulin-like growth factor that modulate the development of the lens vesicle and its invagination into the optic vesicle. The lens placode differentiates from the surface ectoderm at the 4-mm stage (about 3 weeks after conception). By 14 mm (6 weeks) it has separated from the surface ectoderm to form the lens vesicle and the embryonic lens nucleus then begins to form. The lens is supported by the tunica vasculosa lentis which is derived from the hyaloid vascular system. This blood supply is fully formed by 3 months’ gestation after which it begins to atrophy and has disappeared by the end of the seventh month of gestation.
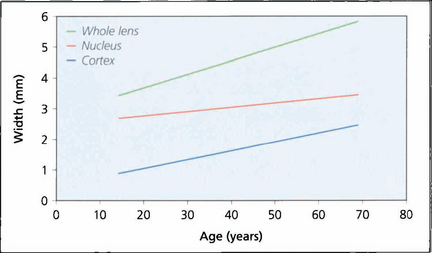
Fig. 11.2 A normal lens increases in thickness with age. The observed increase is the sum of growth by fibre accretion and central compaction. Increasing thickness is largely the result of cortical growth; lenses that develop cataract tend to be smaller than their age-matched norm until the onset of hydration with maturity.
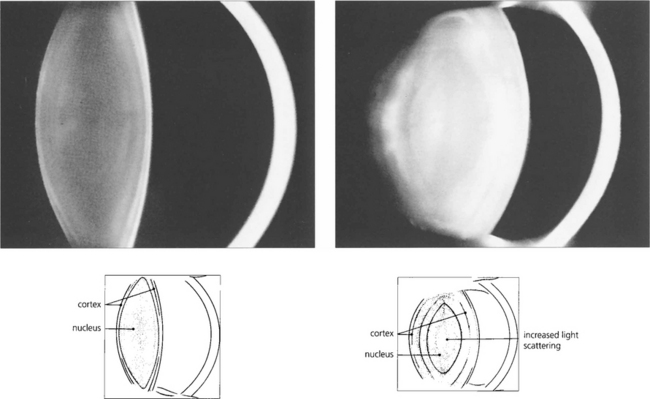
Fig. 11.3 These Scheimphlug images compare the lens of a 10-year-old child with that of an elderly person. In the child the lens is composed largely of nucleus, the cortex being represented only by a thin bright band. The lens in the older person is greatly increased in thickness due almost entirely to growth of the cortex with the nucleus remaining almost unchanged. The scattering properties of the lens are greatly increased in the elderly so that the lens appears to be brighter; in addition the radii of curvature of its surfaces, particularly the anterior surface, are reduced so that the lens becomes more convex.
By courtesy of Mr N Phelps Brown.
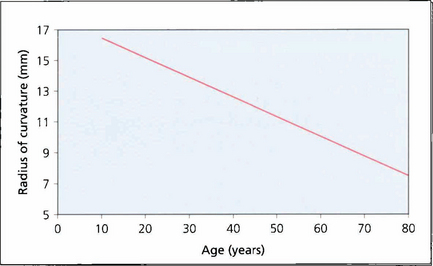
Fig. 11.4 The radius of curvature of the anterior surface of the lens becomes progressively shorter with age (i.e. more convex), resulting in increased postive spherical aberration (see Ch. 1). The cornea has a positive spherical aberration too so that with age there is increasing aberration which leads to image degradation and loss of contrast. This is of some interest as intraocular lenses are now being manufactured with a negative spherical aberration to neutralize the corneal aberration leading to better contrast post- operatively.
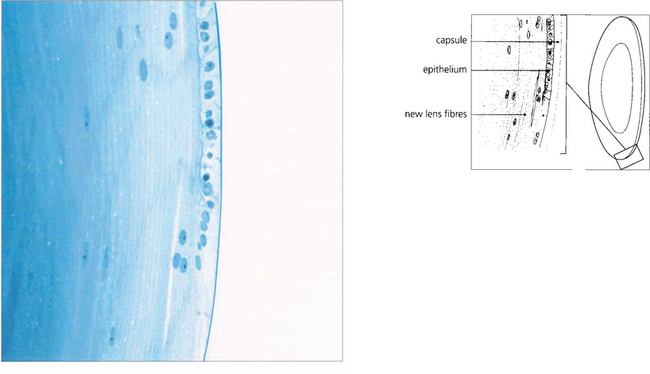
Fig. 11.5 This histological section shows the anterior surface of the lens at the equator. The capsule appears structureless and stains weakly. It is the basement membrane of the lens epithelium and consists of type 4 collagen. It varies in thickness between 10 and 20μm and is thinnest at the anterior and posterior poles and the equator. The lens epithelium, which is present only anteriorly, is composed of a single layer of nucleated cells. It is responsible for the continual production of new lens fibres which are seen to be separating from the epithelial layer in the lower part of the figure. Although the young fibres are nucleated they eventually lose their nuclei as they sink into the cortex.
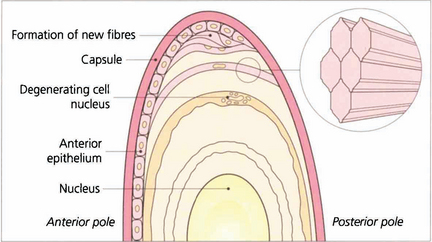
Fig. 11.6 Lens fibres are hexagonal in shape and lie in layers rather like the skin of an onion. Individual fibres are connected to one another by ‘ball and socket’ interdigitations. Initially they are attached to the capsule anteriorly and posteriorly (their basement membrane). With time and the formation of new fibres they lose their capsular attachments, their nuclei and interdigitations and cell membranes to become compacted as insoluble protein in the nucleus.
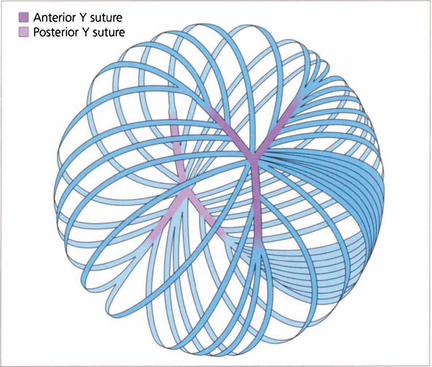
Fig. 11.7 Each fibre is a long ribbon-like structure with ends that stretch to each anterior and posterior pole where they meet a fellow fibre from the opposite side. If the system was completely symmetrical they would meet at a single point but this is not the case and they meet one another in a complicated branching system known as suture lines. The suture lines of the fetal nucleus are easily seen in the normal adult lens as an erect Y anteriorly and an inverted Y posteriorly. With increasing age the sutures become a more complex branching system extending out into the cortex, each lens fibre running anteroposteriorly across the equator of the lens and interdigitating with its neighbours.
ACCOMMODATION
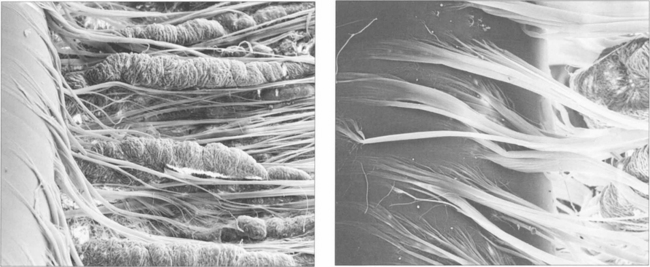
Fig. 11.9 (Left) Zonular fibres can be seen inserting into the lens capule from between the ciliary processes (right).
By courtesy of Professor John Marshall.
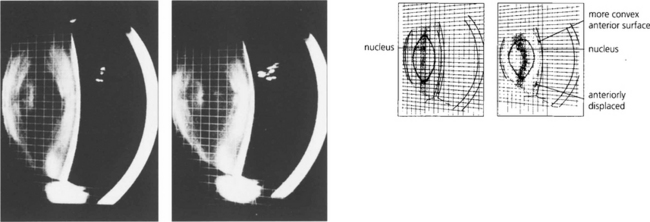
Fig. 11.10 These slit-image Scheimphlug photographs show the changes in a 30-year-old lens focused at infinity (left) and exerting 10D of accommodation (right). The increased curvature of the anterior lens surface and its slight anterior positioning can be seen clearly. The posterior lens surface remains relatively unchanged.
By courtesy of Mr N Phelps Brown.
ANOMALIES OF SHAPE AND POSITION
Anomalies of lens shape and position are rare disorders either resulting from primary lens pathology or due to secondary zonular changes. They are either genetic (in which case there may be other systemic abnormalities) or a result of trauma or pseudo lens exfoliation, which is associated with zonular weakness. Pupil block glaucoma is a common feature of subluxating or dislocating lenses (see Ch. 8). Intact lenses that dislocate posteriorly can lie in the vitreous or on the retinal surface for many years without causing ocular damage.
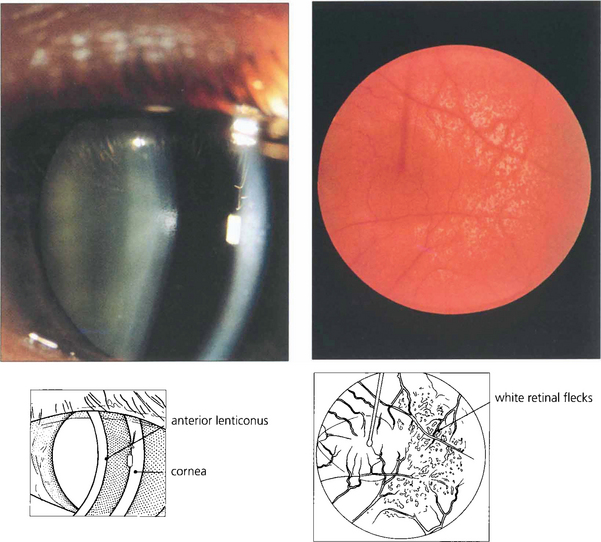
Fig. 11.11 Anterior lenticonus is a feature of Alport’s syndrome. This is a dominant disorder of variable penetrance that is due to a basement membrane defect. As well as the lens defect, which causes irregular astigmatism, the condition is associated with renal failure and neuronal deafness. Patients may have an associated retinopathy of white inner retinal flecks.
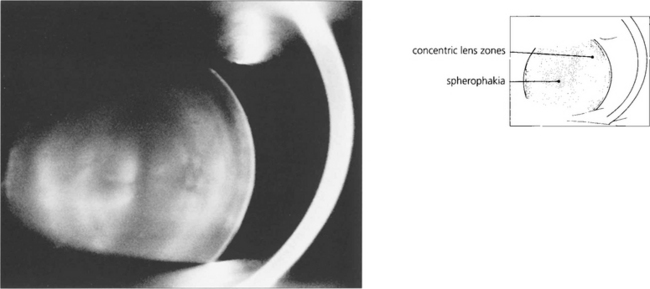
Fig. 11.12 Spherophakia is seen in a number of conditions in which the lens has an abnormally short radius of curvature and may also be exceptionally small (microphakia). Extreme myopia results. Although this lens is normally situated, there is usually a tendency for lenses to subluxate and dislocate anteriorly.
By courtesy of Mr N Phelps Brown.
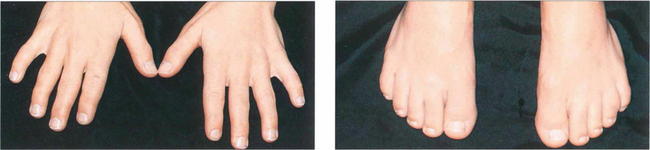
Fig. 11.13 The Weil–Marchesani syndrome is inherited recessively and characterized by short stature and stubby fingers (left) and toes (right), which have stiff joints. Spherophakia, lenticular myopia of 10–20D and lens dislocation are common. Heterozygotes may show a milder form of the disease.
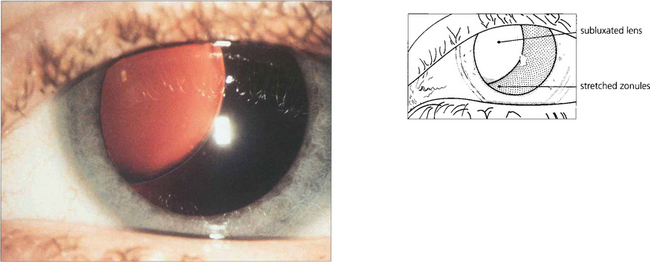
Fig. 11.14 Marfan’s syndrome is a dominantly inherited disorder that affects the eye, skeleton and cardiovascular system. It is due to a deficiency of fibrillin, a component of the microfibrillar system associated with elastin and encoded on chromosome 15q. The lens tends to dislocate upwards (in contrast to homocystinuria where it dislocates inferiorly). The lens is more spherical, causing myopia with marked astigmatism. Management of these patients has been transformed by a complex procedure of lens removal, placement of a sclerally sutured endocapsular ring to recentre the bag and IOL implantation.
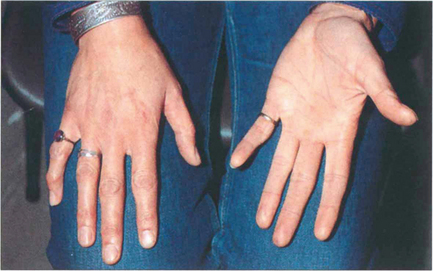
Fig. 11.15 Patients with Marfan’s syndrome have arachnodactyly and an arm span greater than their height. All patients require cardiac monitoring to detect the potentially fatal complications of aortic valve incompetence and a dissecting ascending aortic aneurysm.
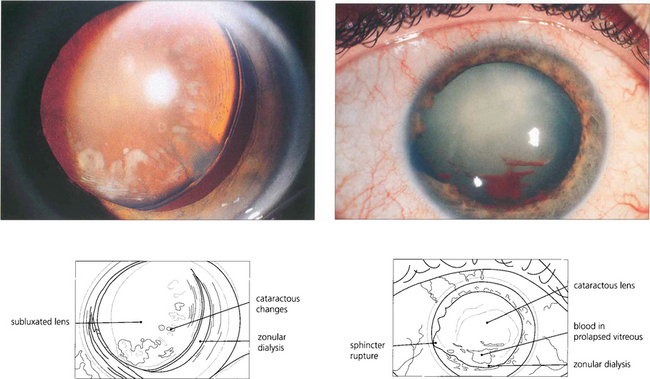
Fig. 11.17 Traumatic zonular dehiscence (left) should be looked for by eliciting phacodonesis on preoperative mydriasis in any patient who has a history of ocular injury. This patient (right) has a traumatic mydriasis, cataract and vitreous in the anterior chamber indicating zonular damage following a blow to the eye; ultrasonography is required to exclude posterior rupture of the lens capsule if the cataractous change is too dense to visualize the capsule.
CATARACT
A cataract is any opacity within the lens. Cataracts are classified according to their morphology and position within the lens and graded by the degree of opacity or ‘maturity’ produced. If lens damage is insufficient to progress to maturity a localized opacity is produced in the injured region that becomes surrounded by new lens fibres as they are laid down beneath the capsule (see glaucomflecken Ch. 7). The three major types of age-related cataract are nuclear, cortical and posterior subcapsular opacity; many patients have combinations of these. It has been suggested that these represent different disease processes: nuclear changes being caused by protein denaturation, cortical by damage to lens fibres and posterior subcapsular cataract by migration of lens epithelial cells posteriorly. This remains to be proven. Occasionally the morphology of a cataract may give an indication of its aetiology (e.g. posterior subcapsular cataract with trauma or steroids) and this may have important medicolegal implications. The morphology does, however, influence the patient’s symptomatology.
Genetic factors have shown to be important risk factors for age related nuclear and cortical opacity; other recognized cataractogenic environmental risks are sunlight, smoking, dehydration and chronic diarrhoea (Table 11.1).
Congenital | Acquired |
---|---|
Maternal infection (e.g. rubella) | Age related |
Genetic | Metabolic (diabetes, hypothyroidism, atopy) |
Metabolic (e.g. galactosaemia) | Drugs (steroids) |
Chromosomal (e.g. Down’s syndrome) | Intraocular disease (uveitis, retinitis pigmentosa) |
Ocular developmental (e.g. Peters’ anomaly) | Trauma (blunt injury, radiotherapy, intraocular surgery) |
Trauma | Genetic (age related nuclear and cortical, Dystrophia myotonica) |